DOI:
10.1039/C8RA05690K
(Paper)
RSC Adv., 2018,
8, 27131-27143
An efficient and ecofriendly synthesis of highly functionalized pyridones via a one-pot three-component reaction†
Received
3rd July 2018
, Accepted 24th July 2018
First published on 30th July 2018
Abstract
A simple and convenient protocol has been developed for the synthesis of N-amino-3-cyano-2-pyridone derivatives by a one-pot reaction of cyanoacetohydrazide, activated nitrile substrates (malononitrile, ethyl cyanoacetate, cyanoacetamide) and aromatic aldehydes in the presence of piperidine in water or a mixture of water and ethanol. The sequence of cascade reactions includes Knoevenagel condensation, Michael addition, intramolecular cyclization, imine-enamine tautomerization and oxidative aromatization. The main advantages of this procedure are availability of starting compounds, simple procedure, mild conditions, easy purification of products and the use of water or water/ethanol as green solvents.
Introduction
The chemistry and applications of pyridine structures have recently attracted a lot of attention due to their uses as synthetic intermediates and their biological importance as agrochemicals,1–3 pharmaceuticals,4–8 dye intermediates,9,10 insecticides, adhesives,11 antifungals, antibacterials,12–14 antidepressant agents,15,16 and antitumor agents.17 In fact the pyridine ring has been found in more than 7000 drugs which are already in existence.18
Furthermore, 2-pyridones are a unique category of pharmacophores which exhibit several biological activities such as antitumoral,19 antimalarial,20 analgesic,21 and anti-HIV.22
Also cyanopyridines are important intermediates for the synthesis of nicotinamide, nicotinic acid and isonicotinic acid. 3-Cyano-2-pyridones are one of the biodynamic cyanopyridine derivatives (Scheme 1).23 The significance of 3-cyano-2-pyridone frameworks in the past few decades is undeniable. For example they are the structural basis of the alkaloid ricinine, the first known alkaloid containing a cyano group. Milrinone is a 3-cyano-2-pyridone derivative that has been used for the treatment of congestive heart failure.24,25 Another derivative 3-cyano-2-pyridone has shown anticancer activity which might be due to the interference of the molecule with PDE-3,26 PIM-1 kinase,27 and survivin protein.28
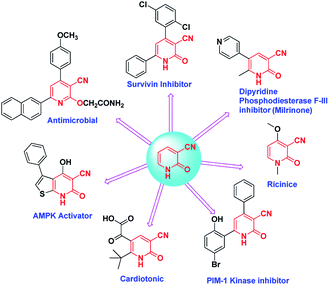 |
| Scheme 1 Biodynamic activities of different derivatives of 3-cyano-2-pyridone. | |
Due to the great importance of this skeleton, the development of efficient and environmentally benign methodologies for the synthesis of diverse functionalized 2-pyridones is still highly desired. There are some strategies for the synthesis of 3-cyano-2-pyridone derivatives which already have been reported, most of them proceed through the regioselective cyclocondensation of an acetonitrile derivative (cyanoacetate ester, cyanoacetamide or malononitrile) with a suitable carbonyl substrate in a [3 + 3] mode. In fact, Michael addition of acetonitrile derivatives to an appropriate carbonyl substrate (1,3-dicarbonyl or α,β-unsaturated compound) and subsequent hydrolytic cyclization followed by oxidative aromatization leads to the corresponding 3-cyano-2-pyridones. Here, we outline some of the most efficient methods (Scheme 2).
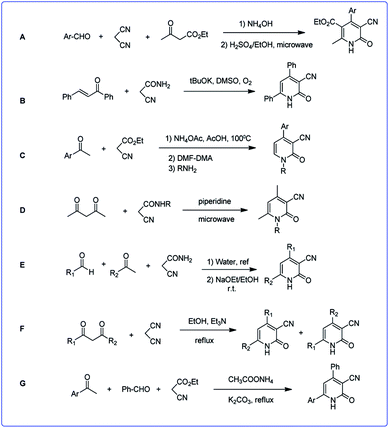 |
| Scheme 2 Summary of previous studies of 3-cyano-2-pyridones synthesis. | |
Reaction of malononitrile, ethyl cyanoacetate and aromatic aldehydes in a two-step reaction led to corresponding 3-cyano-2-pyridones (A).29 In another work the reaction of various enones with cyanoacetamide, led to 3-cyano-2-pyridones by operating in DMSO and in the presence of excess t-BuOK under an oxygen atmosphere (B).30 The Knoevenagel product of aromatic ketones and ethyl acetoacetate reacted with N,N-dimethylformamide-dimethylacetal (DMF-DMA) to produce enaminonitriles. By adding various types of primary nucleophilic amines to enaminonitrile, 3-cyano-2-pyridone derivatives were formed (C).31
Condensation of acetylacetone and corresponding N-substituted cyanoacetamide using microwave irradiation led to pyridones (D).32 In a two-step reaction, firstly acrylamides were obtained via the reaction of aromatic aldehydes and cyanoacetamide in water, then acrylamides were reacted with enolates to produce corresponding 2-pyridones (E).33
Also 3-cyano-2-pyridinone derivatives were prepared in the reaction of 1,3-dicarbonyl compounds with malononitrile followed by cycloaddition and isomerization (F).34 In another study a one-pot reaction of acetophenone derivatives, malononitrile or ethyl cyanoacetate, an aldehyde, and ammonium acetate in the presence of K2CO3 led to the corresponding structures (G).35
Many of the established procedures carried out under harsh reaction conditions. On the other hand, one of the goals in modern synthetic organic chemistry includes the use of safer, easier and more effective methods.
Results and discussion
Here we report a one-pot three-component reaction between cyanoacetohydrazide, acetonitrile derivatives (malononitrile, ethyl cyanoacetate, cyanoacetamide) and aromatic aldehydes. This strategy led to the N-amino-3-cyano-2-pyridones in good yields via a multicomponent reaction. Multicomponent reactions (MCRs) are extremely convergent one-pot processes, in which three or more reagents are combined sequentially to create complex products, with almost all the atoms coming from the starting reagents.36–39
The reaction of cyanoacetohydrazide, malononitrile or ethyl cyanoacetate and aldehydes has been reported in 1984 and 1997 with different procedure and there are no complete spectral data about the structures of products.40,41 Furthermore, synthesis of pyridones using of both cyanoacetamide and cyanoacetohydrazide has not been reported so far.
We succeeded in synthesizing three categories of highly functionalized 2-pyridone structures containing 1,6-diamino-4-aryl-2-oxo-1,2-dihydropyridine-3,5-dicarbonitrile, ethyl 1,6-diamino-4-aryl-3-cyano-2-oxo-1,2-dihydropyridine-5-carboxylate and 1,6-diamino-4-aryl-3-cyano-2-oxo-1,2-dihydropyridine-5-carboxamide.
Continuing our research on multi-component reactions using cyanoacetohydrazide, we will describe in this paper a very efficient and environmentally benign strategy for the synthesis of N-amino-3-cyano-2-pyridone derivatives by a one-pot three component reaction of cyanoacetohydrazide 1, acetonitrile derivatives 2 (malononitrile 2a, ethyl cyanoacetate 2b, cyanoacetamide 2c) and aromatic aldehydes 3 (Scheme 3).
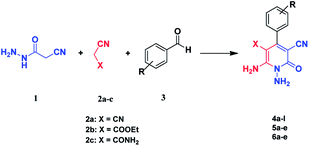 |
| Scheme 3 Synthetic scheme for generation of products 4, 5, 6. | |
For the better study of reactions and procedures and more detailed examination of structures, we divide the reactions into three class based on malononitrile 2a, ethyl cyanoacetate 2b and cyanoacetamide 2c.
As the first group of reactions, we used cyanoacetohydrazide 1, malononitrile 2a and aromatic aldehydes 3a–l in different conditions. The best results were obtained in water in the presence of piperidine as catalyst at room temperature. We could synthesize 12 pyridone derivatives with various aldehydes. In all of these reactions, 1,6-diamino-4-aryl-3,5-dicyano-2-pyridone 4a–l were obtained with high efficiency and relatively short time (Table 1). The structures of compounds 4a–l were derived from their IR, 1H NMR, 13C NMR spectroscopic and mass spectrometric data (see the ESI†).
Table 1 Synthesis of 1,6-diamino-4-aryl-2-oxo-1,2-dihydropyridine-3,5-dicarbonitrile 4a–la
By comparing the reaction rates in the table above, it is found that for aldehydes with an electron withdrawing group on the ring (nitro and halogens), the reaction rate is the highest and with electron donating groups (methoxy), the speed is the lowest.
Here we investigate the 1H and 13C NMR spectra of product 4a. As shown in Fig. 1, the 1H NMR spectrum of 4a showed two signals at δ 5.65 and 8.51 ppm identified as N–NH2 and NH2 groups respectively. These peaks were exchangeable with D2O. The four protons of aromatic ring appeared at δ 7.50 and 7.62 ppm as two doublets. The 1H-decoupled 13C NMR spectrum of 4a indicated 11 distinct resonances in accordance to desired structure. Two signals of C-5 and C-3 of pyridone ring were observed at δ 74.7 and 86.8 ppm respectively. The signals at δ 115.8 and 116.6 ppm were related to two nitrile groups. The signals at δ 129.2, 130.4, 133.8 and 135.5 ppm were assigned to carbons of aryl ring. The carbons of C-4 and C-6 appeared at δ 157.0 and 158.8 ppm. The carbonyl group (C-2) was observed at δ 159.5 ppm (Fig. 1).
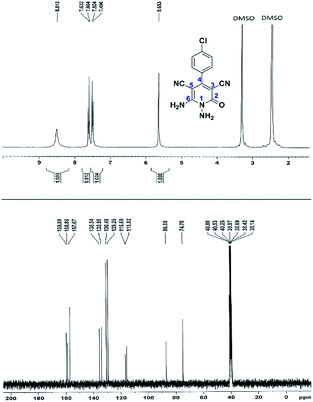 |
| Fig. 1 The 1H and 13C NMR spectrums of 4a. | |
The mass spectrum of 4a displayed the molecular-ion peak at m/z 285 (100%) in agreement with the proposed structure. The IR spectrum of this compound showed absorption bands at 3390, 3283 due to the NH2 groups, stretching vibration of nitrile groups at 2216, strong absorption of carbonyl group at 1623, N–H bending band at 1525, stretching vibration of C
C of aromatic ring at 1465 and C–N band at 1227 cm−1.
According to the above findings, we used ethyl cyanoacetate instead of malononitrile. As a result, ethyl 1,6-diamino-4-aryl-3-cyano-2-pyridone-5-carboxylate derivatives 5a–e were synthesized via the reaction of cyanoacetohydrazide 1, ethyl cyanoacetate 2b and the five aldehydes 3 (Table 2). The best results in this type of reactions were obtained in the mixture of water and ethanol (1
:
1, v/v) in the presence of piperidine at reflux conditions (these reactions in water led to the hydrazone products without participation of ethyl cyanoacetate).
Table 2 Synthesis of ethyl 1,6-diamino-4-aryl-3-cyano-2-oxo-1,2-dihydropyridine-5-carboxylate 5a–ea
The reaction with other aromatic aldehydes was carried out similar to the high work, but did not result in the expected products and the reaction mixture showed several spots in TLC (the desired product was not isolable).
It is interesting to note that when methyl cyanoacetate was used instead of ethyl cyanoacetate in the above reaction, the same ethyl carboxylate products 5a–e were obtained.
The spectral analysis of IR, 1H NMR, 13C NMR and mass spectrums of 5a–e confirmed the formation of expected structures (see the ESI†). For example the 1H NMR spectrum of 5a showed a triplet at δ 0.57 and a quartet at δ 3.73 ppm related to the CH3 and CH2 groups. Protons of N–NH2 group was observed at δ 5.56 ppm. The signals of aromatic ring specified at δ 7.25 and 7.48 ppm as two doublets. Two broad signals related to the NH2 group (position 6) appeared at δ 8.42 and 8.72 ppm separately as a result of intramolecular hydrogen bonding. The 1H-decoupled 13C NMR spectrum of 5a displayed 13 distinct signals in accordance with expected structure. The carbons of methyl and methylene groups appeared at δ 13.2 and 60.5 ppm respectively. The signals of C-3 and C-5 of pyridone ring were observed at δ 88.1 and 91.4 ppm. The nitrile group was assigned at δ 117.0 The signals at δ 129.2, 130.4, 133.8 and 135.5 ppm were related to carbons of aryl ring. The carbons of C-4 and C-6 appeared at δ 156.6 and 158.6 ppm. Two carbonyl groups (C-2 and CO2Et) were observed at δ 159.2 and 166.16 ppm respectively (Fig. 2).
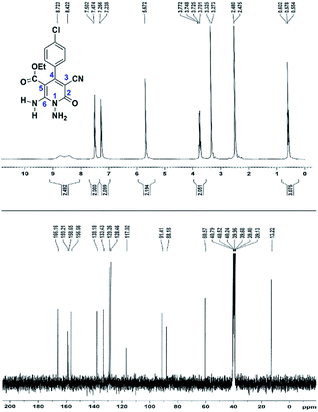 |
| Fig. 2 The 1H and 13C NMR spectrums of 5a. | |
The mass spectrum of 5a afforded a molecular-ion peak at m/z 332 (100%) in conformity with the proposed product. The IR spectrum of this compound indicated absorption bands due to NH2 groups broadly at 3387 and 3266, stretching vibrations of aliphatic C–H bands at 2984, 2923, one vibration of nitrile group at 2215, strong absorptions of carbonyl groups at 1686 and 1651, N–H bending band at 1536, stretching vibration of C
C of aromatic ring at 1473 and C–N stretching band at 1207 cm−1.
In the third section, we used cyanoacetamide as activated nitrile compound. As a result, the reaction of cyanoacetohydrazide 1, cyanoacetamide 2c and some aromatic aldehydes 3 led to 1,6-diamino-4-aryl-3-cyano-2-pyridone-5-carboxamide derivatives 6a–e (Table 3). It was investigated that the best results were obtained in the mixture of water and ethanol (1
:
2, v/v) in the presence of piperidine at reflux conditions (similar to the previous reactions, when water was used alone, the hydrazone compounds were formed as only products).
Table 3 Synthesis of 1,6-diamino-4-aryl-3-cyano-2-oxo-1,2-dihydropyridine-5-carboxamide 6a–ea
This reaction with other aromatic aldehydes was carried out similar to the first work, but did not led to the pure products and the reaction mixture contained several spots in TLC (the expected product was not separable).
The structures of products 6a–e were deduced from their IR, 1H NMR, 13C NMR spectroscopic and mass spectrometric data (see the ESI†). Here we study the 1H and 13C NMR spectra of 6a. As seen in Fig. 3, the 1H NMR spectrum of 6a indicated a singlet at δ 5.67 ppm identified as the N–NH2 group. Two singlet broad signals at δ 6.85 and 7.22 ppm were assigned to the amino group (on C-6). Two doublets at δ 7.28 and 7.65 ppm were related to aryl ring. The amidic NH2 group appeared at δ 7.78 ppm. The 1H-decoupled 13C NMR spectrum of 6a showed 11 resonances which confirmed the assumed structure. The signals of C-3 and C-5 were observed at δ 83.7 and 99.4 ppm respectively. The nitrile group appeared at δ 118.1 ppm. The signals at δ 123.0, 130.8, 131.6 and 136.1 were related to aryl ring. The carbons of C-4 and C-6 were observed at δ 153.8 and 154.0 ppm. Two carbonyl groups (C-2 and CONH2) were assigned at δ 159.6 and 167.3 ppm respectively (Fig. 3).
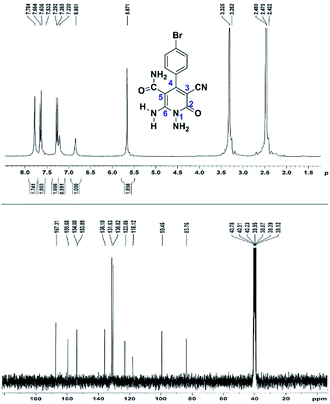 |
| Fig. 3 The 1H and 13C NMR spectrums of 6a. | |
The mass spectrum of 6a showed the molecular-ion peak at m/z 347 (100%) that was in accordance with the desired structure. The IR spectrum of compound 6a showed absorption broad bands at 3394, 3290 related to the NH2 groups, stretching vibrations of nitrile group at 2205, strong absorption of carbonyl groups at 1662 and 1612, N–H bending band at 1571, stretching vibration of C
C of aromatic ring at 1463 and C–N stretching band at 1213 cm−1.
A plausible mechanism for the formation of 2-pyridones 4, 5 and 6 is depicted in Scheme 4. Initial condensation of activated nitriles 2 with aromatic aldehydes 3 in the presence of piperidine, leads to the Knoevenagel products 7. The methylene group of cyanoacetohydrazide loses proton with the catalyst so subsequent Michael addition of cyanoacetohydrazide 1 to adduct 7 affords intermediate 8 which undergoes intramolecular cyclisation via nucleophilic addition of –NH to nitrile group to give the corresponding 1-amino-6-imino-2-piperidinone 9. Successive imine–enamine tautomerization followed by dehydrogenation affords desired products (Scheme 4).
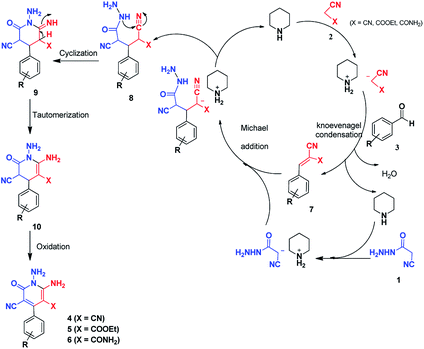 |
| Scheme 4 Proposed mechanism for the formation of products 4, 5, 6. | |
It is necessary to mention this point that cyanoacetohydrazide could conceivably lead to condensation at the hydrazide group with aldehydes, but even when the hydrazone structures were synthesized and added to compounds 2, the same pyridone products were obtained. In fact, the most important part of these reactions was the prevention of hydrazone formation, which was controlled with the mentioned conditions.
Experimental
Materials
All commercially available reagents and other solvents were purchased and used without further purification. The NMR spectra were recorded with a Bruker DRX-300 Avance instrument (300 MHz for 1H and 75.4 MHz for 13C) with DMSO-d6 as solvent. Chemical shifts are given in ppm (δ), and coupling constant (J) are reported in hertz (Hz). Melting points were measured with an electrotherma1 9100 apparatus. Mass spectra were recorded with an Agilent 5975C VL MSD with Triple-Axis Detector operating at an ionization potential of 70 eV. IR spectra were measured with, Bruker Tensor 27 spectrometer (
in cm−1). Elemental analyses for C, H and N were performed using a PerkinElmer 2004 series [II] CHN elemental analyzer.
General procedure for the synthesis of 1,6-diamino-4-aryl-2-oxo-1,2-dihydropyridine-3,5-dicarbonitrile (4a–l)
The stoichiometric mixture of cyanoacetohydrazide 1 (1 mmol, 0.99 g), malononitrile 2a (1 mmol, 0.66 g), aromatic aldehyde 3a–l (1 mmol) and piperidine (20% mol, 0.019 ml) in H2O (10 ml) was stirred at room temperature. The progress of the reaction was monitored by TLC using ethyl acetate/n-hexane (1
:
1). After completion of the reaction, the precipitated product was collected by filtration and washed with warm ethanol to give the pure products 4a–l.
1,6-Diamino-4-(4-chlorophenyl)-2-oxo-1,2-dihydropyridine-3,5-dicarbonitrile (4a). White solid; yield: 0.265 g (93%); mp: 340 °C (dec.); IR (KBr) (νmax/cm−1): 3390, 3283, 3197, 2216, 1623, 1525, 1465, 1227, 841, 766; 1H NMR (300 MHz, DMSO): δ 5.65 (s, 2H, N–NH2), 7.51 (d, J = 8.4 Hz, 2H, ArH), 7.62 (d, J = 8.4 Hz, 2H, ArH), 8.51 (brs, 2H, NH2); 13C{1H} NMR (75.4 MHz, DMSO): δ 74.7 (C–CN), 86.8 (C–CN), 115.8 (CN), 116.6 (CN), 129.2, 130.4, 133.8, 135.5 (Ar), 157 (C-4), 158.8 (C–NH2), 159.5 (C
O); MS (EI, 70 eV): m/z (%) = 287 (35) [M + 2]+, 286 (18) [M + 1]+, 285 (100) [M]+, 256 (19), 241 (20), 205 (13), 193 (7), 161 (10), 138 (5), 111 (4), 88 (3), 75 (6), 58 (3), 43 (2); anal. calcd for C13H8ClN5O: C, 54.65; H, 2.82; N, 24.51. Found: C, 54.65; H, 2.71; N, 24.39.
1,6-Diamino-4-(4-bromophenyl)-2-oxo-1,2-dihydropyridine-3,5-dicarbonitrile (4b). White solid; yield: 0.289 g (88%); mp: 355 °C (dec.); IR (KBr) (νmax/cm−1): 3450, 3396, 3258, 2212, 1600, 1522, 1466, 1297, 829, 613; 1H NMR (300 MHz, DMSO): δ 5.64 (s, 2H, N–NH2), 7.43 (d, J = 8.1 Hz, 2H, ArH), 7.59 (d, J = 8.1 Hz, 2H, ArH), 8.50 (brs, 2H, NH2); 13C{1H} NMR (75.4 MHz, DMSO): δ 74.7 (C–CN), 86.7 (C–CN), 115.8 (CN), 116.6 (CN), 124.3, 130.6, 132.1, 134.2 (Ar), 157 (C-4), 158.8 (C–NH2), 159.5 (C
O); MS (EI, 70 eV): m/z (%) = 331 (98) [M + 2]+, 330 (21) [M + 1]+, 329 (100) [M]+, 300 (12), 285 (17), 258 (6), 222 (14), 205 (26), 193 (14), 165 (12), 152 (13), 126 (14), 100 (9), 88 (7), 75 (10), 58 (6), 43 (3); anal. calcd for C13H8BrN5O: C, 47.29; H, 2.44; N, 21.21. Found: C, 47.26; H, 2.28; N, 21.07.
1,6-Diamino-4-(4-nitrophenyl)-2-oxo-1,2-dihydropyridine-3,5-dicarbonitrile (4c). Yellow solid; yield: 0.269 g (91%); mp: 360 °C (dec.); 1H NMR (300 MHz, DMSO): δ 5.50 (s, 2H, N–NH2), 7.84 (d, J = 9 Hz, 2H, ArH), 8.06 (brs, 2H, NH2); 8.44 (d, J = 9 Hz, 2H, ArH), 13C{1H} NMR (75.4 MHz, DMSO): δ 74.6 (C–CN), 86.7 (C–CN), 115.5 (CN), 116.4 (CN), 124.3, 130.2, 141.3, 148.9 (Ar), 157.0 (C-4), 158 (C–NH2), 159.4 (C
O); MS (EI, 70 eV): m/z (%) = 297 (17) [M + 1]+, 296 (100) [M]+, 267 (11), 252 (14), 230 (3), 205 (4), 193 (5), 165 (8), 152 (7), 126 (5), 100 (4), 88 (4), 58 (3), 43 (2); anal. calcd for C13H8N6O3: C, 52.71; H, 2.72; N, 28.37. Found: C, 52.64; H, 2.53; N, 28.19.
1,6-Diamino-4-(4-methoxyphenyl)-2-oxo-1,2-dihydropyridine-3,5-dicarbonitrile (4d). Pale yellow solid; yield: 0.233 g (83%); mp: 321–323 °C; IR (KBr) (νmax/cm−1): 3393, 3316, 3264, 2211, 1640, 1513, 1419, 1256, 1023, 837; 1H NMR (300 MHz, DMSO): δ 3.81 (s, 3H, OCH3), 5.62 (s, 2H, N–NH2), 7.07 (d, J = 8.7 Hz, 2H, ArH), 7.43 (d, J = 8.7 Hz, 2H, ArH), 8.40 (brs, 2H, NH2); 13C{1H} NMR (75.4 MHz, DMSO): δ 55.79 (OCH3), 74.7 (C–CN), 86.7 (C–CN), 114.4 (CN), 116.2 (CN), 117, 126.9, 130.3, 157.7 (Ar), 159.7 (C-4), 159.8 (C–NH2), 161.1 (C
O); MS (EI, 70 eV): m/z (%) = 282 (18) [M + 1]+, 281 (100) [M]+, 266 (4), 252 (18), 237 (27), 210 (13), 195 (8), 180 (6), 167 (5), 140 (3), 114 (9), 88 (6), 58 (4), 43 (7); anal. calcd for C14H11N5O2: C, 59.78; H, 3.94; N, 24.90. Found: C, 59.39; H, 3.79; N, 24.85.
1,6-Diamino-4-(3-methoxyphenyl)-2-oxo-1,2-dihydropyridine-3,5-dicarbonitrile (4e). White solid; yield: 0.238 g (85%); mp: 265–267 °C; IR (KBr) (νmax/cm−1): 3425, 3314, 3194, 2954, 2205, 1682, 1617, 1526, 1464, 1292, 1159, 884; 1H NMR (300 MHz, DMSO): δ 3.78 (s, 3H, OCH3), 5.57 (s, 2H, N–NH2), 6.99–7.10 (m, 3H, ArH), 7.44 (t, J = 8.1 Hz, 1H, ArH), 8.44 (brs, 2H, NH2); 13C{1H} NMR (75.4 MHz, DMSO): δ 55.78 (OCH3), 74.7 (C–CN), 86.8 (C–CN), 114.1 (CN), 115.8 (CN), 116, 116.7, 120.5, 130.3, 136.2, 157 (Ar), 159.4 (C-4), 159.6 (C–NH2), 159.7 (C
O); MS (EI, 70 eV): m/z (%) = 282 (18) [M + 1]+, 281 (100) [M]+, 280 (22), 265 (5), 252 (18), 237 (16), 210 (3), 195 (7), 180 (6), 167 (5), 140 (6), 114 (6), 88 (5), 63 (5), 43 (2); anal. calcd for C14H11N5O2: C, 59.78; H, 3.94; N, 24.90. Found: C, 59.60; H, 3.84; N, 24.85.
1,6-Diamino-4-phenyl-2-oxo-1,2-dihydropyridine-3,5-dicarbonitrile (4f). White solid; yield: 0.225 g (90%); mp: 332–334 °C; IR (KBr) (νmax/cm−1): 3444, 3397, 3240, 2212, 1630, 1525, 1469, 1219, 858, 739; 1H NMR (300 MHz, DMSO): δ 5.64 (s, 2H, N–NH2), 7.36–7.52 (m, 5H, ArH), 8.45 (brs, 2H, NH2); 13C{1H} NMR (75.4 MHz, DMSO): δ 74.7 (C–CN), 86.8 (C–CN), 115.9 (CN), 116.8 (CN), 128.4, 129, 130.6, 135 (Ar), 157.1 (C-4), 159.7 (C–NH2), 160 (C
O); MS (EI, 70 eV): m/z (%) = 252 (18) [M + 1]+, 251 (100) [M]+, 236 (2), 222 (21), 207 (26), 194 (7), 180 (17), 165 (8), 127 (14), 88 (5), 77 (12), 51 (10), 43 (4); anal. calcd for C13H9N5O: C, 62.15; H, 3.61; N, 27.87. Found: C, 61.79; H, 3.56; N, 27.62.
1,6-Diamino-4-(2-chlorophenyl)-2-oxo-1,2-dihydropyridine-3,5-dicarbonitrile (4g). White solid; yield: 0.247 g (87%); mp: 305–307 °C; IR (KBr) (νmax/cm−1): 3411, 3305, 3205, 2213, 1678, 1561, 1466, 1228, 880, 757; 1H NMR (300 MHz, DMSO): δ 5.66 (s, 2H, N–NH2), 7.41–7.66 (m, 4H, ArH), 8.59 (brs, 2H, NH2); 13C{1H} NMR (75.4 MHz, DMSO): δ 75.3 (C–CN), 87.5 (C–CN), 115.1 (CN), 116 (CN), 128.2, 130.1, 130.2, 131, 132.1, 134.2 (Ar), 157 (C-4), 157.7 (C–NH2), 159.5 (C
O); MS (EI, 70 eV): m/z (%) = 287 (37) [M + 2]+, 286 (30) [M + 1]+, 285 (100) [M]+, 276 (3), 250 (23), 222 (4), 205 (13), 193 (10), 161 (12), 138 (10), 111 (5), 88 (6), 67 (3), 58 (4), 43 (2); anal. calcd for C13H8ClN5O: C, 54.65; H, 2.82; N, 24.51. Found: C, 55.14; H, 2.45; N, 24.31.
1,6-Diamino-4-(3-chlorophenyl)-2-oxo-1,2-dihydropyridine-3,5-dicarbonitrile (4h). White solid; yield: 0.242 g (85%); mp: 292–294 °C; 1H NMR (300 MHz, DMSO): δ 5.66 (s, 2H, N–NH2), 7.42–7.62 (m, 4H, ArH), 8.52 (brs, 2H, NH2); 13C{1H} NMR (75.4 MHz, DMSO): δ 74.8 (C–CN), 86.9 (C–CN), 115.7 (CN), 116.6 (CN), 127.2, 128.2, 130.5, 131.1, 133.6, 137 (Ar), 157 (C-4), 158.4 (C–NH2), 159.5 (C
O); anal. calcd for C13H8ClN5O: C, 54.65; H, 2.82; N, 24.51. Found: C, 55.53; H, 2.67; N, 24.50.
1,6-Diamino-2-oxo-4-(4-(trifluoromethyl)phenyl)-1,2-dihydropyridine-3,5-dicarbonitrile (4i). Pale brown solid; yield: 0.255 g (80%); mp: 290–293 °C; 1H NMR (300 MHz, DMSO): δ 5.61 (s, 2H, N–NH2), 7.72 (d, J = 8.1 Hz, 2H, ArH), 7.93 (d, J = 8.1 Hz, 2H, ArH), 8.57 (brs, 2H, NH2); 13C{1H} NMR (75.4 MHz, DMSO): δ 74.7 (C–CN), 86.8 (C–CN), 115.6 (CN), 116.5 (CN), 122.5 (CF3), 126.1, 129.5, 131.03, 139.1, 135.5 (Ar), 157.1 (C-4), 158.6 (C–NH2), 159.5 (C
O); MS (EI, 70 eV): m/z (%) = 320 (17) [M + 1]+, 319 (100) [M]+, 300 (12), 275 (24), 248 (10), 195 (11), 176 (10), 145 (9), 111 (4), 99 (6), 88 (5), 69 (15), 57 (8), 43 (11); anal. calcd for C14H8F3N5O: C, 52.67; H, 2.53; N, 21.94. Found: C, 52.35; H, 2.81; N, 21.67.
1,6-Diamino-4-(3,4-dimethoxyphenyl)-2-oxo-1,2-dihydropyridine-3,5-dicarbonitrile (4j). Pale yellow solid; yield: 0.233 g (75%); mp: 290–292 °C; IR (KBr) (νmax/cm−1): 3402, 3291, 2966, 2845, 2215, 1673, 1613, 152, 1464, 1269, 1153, 1019, 866, 762; 1H NMR (300 MHz, DMSO): δ 3.77 (s, 3H, OCH3), 3.81 (s, 3H, OCH3), 5.64 (s, 2H, N–NH2), 7.02–7.10 (m, 3H, ArH), 8.40 (brs, 2H, NH2); 13C{1H} NMR (75.4 MHz, DMSO): δ 56 (OCH3), 56.1 (OCH3), 74.7 (C–CN), 86.7 (C–CN), 111.84, 112.4 (Ar), 116.2 (CN), 117.1 (CN), 121.6, 126.9, 148.6, 150.7 (Ar), 157.1 (C-4), 159.7 (C–NH2), 159.8 (C
O); MS (EI, 70 eV): m/z (%) = 312 (24) [M + 1]+, 311 (100) [M]+, 296 (7), 268 (13), 237 (5), 209 (4), 181 (4), 151 (10), 127 (3), 101 (3), 88 (2), 77 (3), 58 (2), 43 (3); anal. calcd for C15H13N5O3: C, 57.87; H, 4.21; N, 22.50. Found: C, 57.60; H, 4.20; N, 22.22.
1,6-Diamino-4-(4-fluorophenyl)-2-oxo-1,2-dihydropyridine-3,5-dicarbonitrile (4k). White solid; yield: 0.223 g (83%); mp: 338–340 °C; IR (KBr) (νmax/cm−1):3390, 3287, 3196, 2211, 1627, 1516, 1463, 1224, 1157, 945, 848, 616; 1H NMR (300 MHz, DMSO): δ 5.65 (s, 2H, N–NH2), 7.35–7.57 (m, 4H, ArH), 8.49 (brs, 2H, NH2); 13C{1H} NMR (75.4 MHz, DMSO): δ 74.9 (C–CN), 87 (C–CN), 115.9 (CN), 116 (CN), 116.5, 131.1, 131.4, 163.4 (Ar), 157 (C-4), 159 (C–NH2), 159.6 (C
O); anal. calcd for C13H8FN5O: C, 57.99; H, 2.99; N, 26.01. Found: C, 57.95, 2.89; 25.87.
1,6-Diamino-4-(3-fluorophenyl)-2-oxo-1,2-dihydropyridine-3,5-dicarbonitrile (4l). White solid; yield: 0.209 g (78%); mp: 340 °C (dec.); IR (KBr) (νmax/cm−1): 3382, 3298, 3206, 2215, 1619, 1527, 1465, 1244, 1019, 965, 884, 761; 1H NMR (300 MHz, DMSO): δ 5.66 (s, 2H, N–NH2), 7.29–7.62 (m, 4H, ArH), 8.52 (brs, 2H, NH2); 13C{1H} NMR (75.4 MHz, DMSO): δ 74.8 (C–CN), 86.9 (C–CN), 115.6 (Ar), 115.8 (CN), 116.5 (CN), 117.5, 124.7, 131.4, 137.1, 162.1 (Ar), 157 (C-4), 158.5 (C–NH2), 159.5 (C
O); anal. calcd for C13H8FN5O: C, 57.99; H, 2.99; N, 26.01. Found: C, 56.73; H, 3.83; N, 24.11.
General procedure for the synthesis of ethyl 1,6-diamino-4-aryl-3-cyano-2-oxo-1,2-dihydropyridine-5-carboxylate (5a–e)
The stoichiometric mixture of cyanoacetohydrazide 1 (1 mmol, 0.99 g), ethyl cyanoacetate 2b (1 mmol, 0.113 g), aromatic aldehyde (1 mmol) and piperidine (20% mol, 0.019 ml) in a mixture of H2O and ethanol (5
:
5 ml) was stirred at 80 °C. Upon completion the reaction as monitored by TLC (using ethyl acetate/n-hexane (1
:
1)), the precipitated product was collected by filtration and washed with H2O/EtOH to give the pure products 5a–e.
Ethyl 1,6-diamino-4-(4-chlorophenyl)-3-cyano-2-oxo-1,2-dihydropyridine-5-carboxylate (5a). White solid; yield: 0.288 g (87%); mp: 245–247 °C; IR (KBr) (νmax/cm−1): 3387, 3226, 2984, 2923, 2215, 1686, 1651, 1585, 1473, 1207, 1092, 839, 618; 1H NMR (300 MHz, DMSO): δ 0.57 (t, J = 7.2 Hz, 3H, CH3), 3.73 (q, J = 7.2 Hz, 2H, CH2), 5.67 (s, 2H, N–NH2), 7.25 (d, J = 8.4 Hz, 2H, ArH), 7.49 (d, J = 8.4 Hz, 2H, ArH), 8.42 (brs, 1H, NH2), 8.72 (brs, 1H, NH2); 13C{1H} NMR (75.4 MHz, DMSO): δ 13.2 (CH3), 60.5 (O–CH2), 88.1 (C–CN), 91.4 (C–CO2Et), 117 (CN), 128.4, 129.2, 133.4, 138.1 (Ar), 156.6 (C-4), 158.6 (C–NH2), 159.2 (C
O), 166.1 (CO2Et); MS (EI, 70 eV): m/z (%) = 334 (35) [M + 2]+, 333 (21) [M + 1]+, 332 (100) [M]+, 303 (5), 286 (13), 251 (13), 229 (21), 187 (5), 162 (12), 138 (7), 111 (4), 75 (4), 58 (3), 43 (5); anal. calcd for C15H13ClN4O3: C, 54.14; H, 3.94; N, 16.84. Found: C, 53.86; H, 3.74; N, 16.47.
Ethyl 1,6-diamino-4-(4-bromophenyl)-3-cyano-2-oxo-1,2-dihydropyridine-5-carboxylate (5b). White solid; yield: 0.319 g (85%); mp: 245–247 °C; IR (KBr) (νmax/cm−1): 3391, 3270, 2216, 1686, 1588, 1537, 1471, 1207, 1091, 878, 768, 615; 1H NMR (300 MHz, DMSO): δ 0.57 (t, J = 6.9 Hz, 3H, CH3), 3.73 (q, J = 6.9 Hz, 2H, CH2), 5.66 (s, 2H, N–NH2), 7.19 (d, J = 8.1 Hz, 2H, ArH), 7.62 (d, J = 8.1 Hz, 2H, ArH), 8.42 (brs, 1H, NH2), 8.72 (brs, 1H, NH2); 13C{1H} NMR (75.4 MHz, DMSO): δ 13.1 (CH3), 60.5 (O–CH2), 88 (C–CN), 91.3 (C–CO2Et), 117 (CN), 121.9, 129.5, 131.3, 138.5 (Ar), 156.6 (C-4), 158.6 (C–NH2), 159.2 (C
O), 166.1 (CO2Et); MS (EI, 70 eV): m/z (%) = 379 (19) [M+2]+, 378 (100) [M+1]+, 377 (21) [M]+, 376 (99), 349 (4), 332 (9), 304 (9), 275 (18), 223 (18), 194 (14), 152 (8), 127 (12), 100 (5), 88 (5), 43 (3); anal. calcd for C15H13BrN4O3: C, 47.76; H, 3.47; N, 14.85. Found: C, 47.43; H, 3.75; N, 14.53.
Ethyl 1,6-diamino-4-(2-chlorophenyl)-3-cyano-2-oxo-1,2-dihydropyridine-5-carboxylate (5c). White solid; yield: 0.265 g (80%); mp: 322–324 °C; IR (KBr) (νmax/cm−1): 3386, 3269, 2214, 1653, 1584, 1456, 1312, 1213, 1006, 800, 621; 1H NMR (300 MHz, DMSO): δ 0.55 (t, J = 6.9 Hz, 3H, CH3), 3.73 (q, J = 6.9 Hz, 2H, CH2), 5.69 (s, 2H, N–NH2), 7.24–7.52 (m, 4H, ArH), 8.47 (brs, 1H, NH2), 9.00 (brs, 1H, NH2); 13C{1H} NMR (75.4 MHz, DMSO): δ 13.2 (CH3), 60.5 (O–CH2), 88.5 (C–CN), 91 (C–CO2Et), 116.4 (CN), 127.4, 129, 129.3, 130.2, 130.7, 138.4 (Ar), 157 (C-4), 159.2 (C–NH2), 159.2 (C
O), 165.8 (CO2Et); MS (EI, 70 eV): m/z (%) = 334 (6) [M + 2]+, 333 (3) [M + 1]+, 332 (17) [M]+, 297 (100), 269 (95), 253 (12), 224 (11), 174 (6), 138 (7), 113 (3), 99 (3), 75 (3), 58 (2), 43 (2); anal. calcd for C15H13ClN4O3: C, 54.14; H, 3.94; N, 16.84. Found: C, 56.05; H, 4.04; N, 16.71.
Ethyl 1,6-diamino-4-(3-fluorophenyl)-3-cyano-2-oxo-1,2-dihydropyridine-5-carboxylate (5d). White solid; yield: 0.237 g (75%); mp: 218–220 °C; IR (KBr) (νmax/cm−1): 3390, 3268, 2217, 1687, 1652, 1537, 1456, 1310, 1208, 1096, 884; 1H NMR (300 MHz, DMSO): δ 0.57 (t, J = 7.2 Hz, 3H, CH3), 3.74 (q, J = 7.2 Hz, 2H, CH2), 5.68 (s, 2H, N–NH2), 7.05 (d, J = 7.8 Hz, 1H, ArH), 7.14 (d, J = 9.6 Hz, 1H, ArH), 7.25 (t, J = 8.4 Hz, 1H, ArH), 7.45 (q, J = 8.1 Hz, 1H, ArH), 8.43 (brs, 1H, NH2), 8.78 (brs, 1H, NH2); 13C{1H} NMR (75.4 MHz, DMSO): δ 13.2 (CH3), 60.4 (O–CH2), 88.2 (C–CN), 91.3 (C–CO2Et), 116.9 (CN), 114.5, 115.3, 123.6, 130.5, 141.4, 163.8 (Ar), 156.7 (C-4), 158.3 (C–NH2), 159.1 (C
O), 166.1 (CO2Et); MS (EI, 70 eV): m/z (%) = 317 (19) [M + 1]+, 316 (100) [M]+, 287 (4), 270 (30), 213 (26), 171 (6), 146 (13), 111 (2), 75 (3), 58 (2), 43 (2); anal. calcd for C15H13FN4O3: C, 56.96; H, 4.14; N, 17.71. Found: C, 56.42; H, 4.28; N, 17.34.
Ethyl 1,6-diamino-4-(4-fluorophenyl)-3-cyano-2-oxo-1,2-dihydropyridine-5-carboxylate (5e). White solid; yield: 0.237 g (75%); mp: 237–239 °C; 1H NMR (300 MHz, DMSO): δ 0.59 (t, J = 7.2 Hz, 3H, CH3), 3.73 (q, J = 7.2 Hz, 2H, CH2), 5.66 (s, 2H, N–NH2), 7.26 (d, J = 7.8 Hz, 4H, ArH), 8.55 (br, 2H, NH2); 13C{1H} NMR (75.4 MHz, DMSO): δ 13.2 (CH3), 60.5 (O–CH2), 88.2 (C–CN), 91.7 (C–CO2Et), 117.1 (CN), 115.4, 129.5, 135.5, 163.2 (Ar), 156.5 (C-4), 158.9 (C–NH2), 159.3 (C
O), 166.2 (CO2Et); anal. calcd for C15H13FN4O3: C, 56.96; H, 4.14; N, 17.71. Found: C, 56.14; H, 4.08; N, 17.61.
General procedure for the synthesis of 1,6-diamino-4-aryl-3-cyano-2-oxo-1,2-dihydropyridine-5-carboxamide (6a–e)
The stoichiometric mixture of cyanoacetohydrazide 1 (1 mmol, 0.99 g), cyanoacetamide 2c (1 mmol, 0.084 g), aromatic aldehyde (1 mmol) and piperidine (20% mol, 0.019 ml) in a mixture of H2O and ethanol (3
:
6 ml) was stirred at 80 °C. After completion of the reaction that was monitored by TLC (using ethyl acetate/n-hexane (1
:
1)), the precipitated product was collected by filtration and washed with H2O/EtOH to give the pure products 6a–e.
1,6-Diamino-4-(4-bromophenyl)-3-cyano-2-oxo-1,2-dihydropyridine-5-carboxamide (6a). Brown solid; yield: 0.278 g (80%); mp: 245–247 °C; IR (KBr) (νmax/cm−1): 3394, 3290, 2205, 1662, 1612, 1571, 1463, 1399, 1267, 886, 579; 1H NMR (300 MHz, DMSO): δ 5.67 (s, 2H, N–NH2), 6.85 (brs, 1H, NH2), 7.22 (brs, 1H, NH2), 7.28 (d, J = 8.4 Hz, 2H, ArH), 7.65 (d, J = 8.4 Hz, 2H, ArH), 7.78 (s, 2H, CONH2); 13C{1H} NMR (75.4 MHz, DMSO): δ 83.7 (C–CN), 99.4 (C–CONH2), 118.1 (CN), 123, 130.8, 131.6, 136.1 (Ar), 153.8 (C-4), 154 (C–NH2), 159.6 (C
O), 167.3 (CONH2); MS (EI, 70 eV): m/z (%) = 350 (16) [M + 2]+, 349 (99) [M + 1]+, 348 (64) [M]+, 347 (100), 346 (51), 332 (5), 301 (5), 275 (4), 251 (43), 237 (8), 205 (16), 194 (24), 180 (9), 152 (9), 140 (18), 113 (8), 100 (9), 88 (8), 63 (9), 44 (22); anal. calcd for C13H10BrN5O2: C, 44.85; H, 2.90; N, 20.12. Found: C, 41.97; H, 3.38; N, 17.42.
1,6-Diamino-4-(4-chlorophenyl)-3-cyano-2-oxo-1,2-dihydropyridine-5-carboxamide (6b). Pale brown solid; yield: 0.236 g (78%); mp: 280–282 °C; IR (KBr) (νmax/cm−1): 3386, 3301, 2208, 1635, 1574, 1461, 1398, 1268, 880, 585; 1H NMR (300 MHz, DMSO): δ 5.67 (s, 2H, N–NH2), 6.83 (brs, 1H, NH2), 7.21 (brs, 1H, NH2), 7.35 (d, J = 8.4 Hz, 2H, ArH), 7.51 (d, J = 8.4 Hz, 2H, ArH), 7.78 (brs, 2H, CONH2); 13C{1H} NMR (75.4 MHz, DMSO): δ 83.8 (C–CN), 99.4 (C–CONH2), 118.1 (CN), 128.7, 130.5, 134.3, 135.7 (Ar), 153.8 (C-4), 154 (C–NH2), 159.6 (C
O), 167.3 (CONH2); anal. calcd for C13H10ClN5O2: C, 51.41; H, 3.32; N, 23.06. Found: C, 51.87; H, 3.54; N, 22.57.
1,6-Diamino-4-(3-chlorophenyl)-3-cyano-2-oxo-1,2-dihydropyridine-5-carboxamide (6c). White solid; yield: 0.227 g (75%); mp: 322–324 °C; 1H NMR (300 MHz, DMSO): δ 5.67 (s, 2H, N–NH2), 6.91 (brs, 1H, NH2), 7.26 (brs, 1H, NH2), 7.28–7.48 (m, 4H, ArH), 7.79 (s, 2H, CONH2); 13C{1H} NMR (75.4 MHz, DMSO): δ 83.7 (C–CN), 99.5 (C–CONH2), 118 (CN), 127.4, 128.4, 129.4, 130.5, 133.1, 138.8 (Ar), 153.3 (C-4), 153.9 (C–NH2), 159.6 (C
O), 167.2 (CONH2); anal. calcd for C13H10ClN5O2: C, 51.41; H, 3.32; N, 23.06.
1,6-Diamino-4-(2-chlorophenyl)-3-cyano-2-oxo-1,2-dihydropyridine-5-carboxamide (6d). Pale brown solid; yield: 0.212 g (70%); mp: 218–220 °C; 1H NMR (300 MHz, DMSO): δ 5.69 (s, 2H, N–NH2), 6.37 (br, 1H, NH2), 7.27 (br, 1H, NH2), 7.42–7.54 (m, 4H, ArH), 8.05 (s, 2H, CONH2); 13C{1H} NMR (75.4 MHz, DMSO): δ 84.7 (C–CN), 99.2 (C–CONH2), 117.4 (CN), 127.4, 130, 130.6, 131.1, 131.4, 135.8 (Ar), 152.4 (C-4), 154.6 (C–NH2), 159.4 (C
O), 166.8 (CONH2); anal. calcd for C13H10ClN5O2: C, 51.41; H, 3.32; N, 23.06.
1,6-Diamino-4-(4-fluorophenyl)-3-cyano-2-oxo-1,2-dihydropyridine-5-carboxamide (6e). Pale brown solid; yield: 0.186 g (65%); mp: 237–239 °C; 1H NMR (300 MHz, DMSO): δ 5.66 (s, 2H, N–NH2), 6.77 (brs, 1H, NH2), 7.21 (brs, 1H, NH2), 7.25–7.37 (m, 4H, ArH), 7.77 (s, 2H, CONH2); 13C{1H} NMR (75.4 MHz, DMSO): δ 84.1 (C–CN), 99.5 (C–CONH2), 118.1 (CN), 115.6, 130.9, 131, 133.2 (Ar), 154 (C-4), 154.1 (C–NH2), 159.7 (C
O), 167.4 (CONH2); MS (EI, 70 eV): m/z (%) = 288 (9) [M + 1]+, 287 (51) [M]+, 286 (21), 270 (17), 241 (10), 205 (26), 177 (12), 146 (18), 122 (100), 109 (24), 95 (25), 75 (13), 57 (11), 44 (16); anal. calcd for C13H10FN5O2: C, 54.36; H, 3.51; N, 24.38. Found: C, 56.09; H, 4.15; N, 19.43.
Conclusion
We have reported a novel and green one-pot synthesis of three classes of polysubstituted pyridine systems, 1,6-diamino-4-aryl-3,5-dicyano-2-pyridone, ethyl 1,6-diamino-4-aryl-3-cyano-2-pyridone-5-carboxylate and 1,6-diamino-4-aryl-3-cyano-2-pyridone-5-carboxamide derivatives, via a three-component reaction between cyanoacetohydrazide, activated nitrile compounds and aromatic aldehydes. The present process includes some important advantages like easy operation mild reaction conditions, facile accessibility of reactants, simple workup procedure, the use of water or water/ethanol as green reaction medium, high atom economy and good to high yields.
Conflicts of interest
The authors declare no competing financial interest.
Acknowledgements
Financial support of this research from Imam Khomeini International University, Iran is gratefully acknowledged.
Notes and references
- S. C. Benson, J. L. Gross and J. K. Snyder, J. Org. Chem., 1990, 55, 3257–3269 CrossRef.
- A. Thomass, M. Chakraborty and H. Junjappa, Tetrahedron, 1990, 46, 577–586 CrossRef.
- J. Wolff and M. Taddei, Tetrahedron, 1986, 42, 4267–4272 CrossRef.
- E. C. Taylor, J. Heterocycl. Chem., 1990, 27, 1–12 CrossRef.
- Y. Tominaga, S. Kohra, H. Honkawa and A. Hosomi, Heterocycles, 1989, 28, 1409 CrossRef.
- Y. Tominaga, S. Mdokawa, Y. Shiroshita and A. Hosomi, J. Heterocycl. Chem., 1987, 24, 1365 CrossRef.
- C. J. Shishoo, M. B. Devani, V. S. Bhadti, S. Ananthan and G. V. Ullas, Tetrahedron Lett., 1983, 24, 4611–4612 CrossRef.
- Y. Tominaga, S. Kohra, H. Okuda, A. Ushirogouchi, Y. Matsuda and G. kobayshi, Chem. Pharm. Bull., 1984, 32, 122 CrossRef.
- E. Hahn, in Lectures in Heterocyclic Chemistry IX, ed. R. N. Castle, Heterocorporation, Tampa, FL, 1990, p. 13 Search PubMed.
- F. Sanger, S. Coulson and A. R. Coulson, Proc. Natl. Acad. Sci. U. S. A., 1977, 74, 5463 CrossRef.
- Y. Higashio and T. Shoji, Appl. Catal., A, 2004, 260, 251–259 CrossRef.
- G. A. Youngdale, US Pat. 4288440, 1980Chem. Abstr., 1982, 96, 6596.
- A. H. Todd, UK Pat. 1203,149, 1970Chem. Abstr., 1970, 73, 120509.
- G. Lohaus, W. Dittmar and S. Afric, IN Pat. 6906036, 1968, Chem. Abstr., 1970, 73, 120508.
- C. Gachet, M. Cattanea, P. Ohlmann, B. Lecchi, J. Cassel, P. Mannucci and J. P. Cazenave, Br. J. Haematol., 1995, 91, 434 CrossRef PubMed.
- F. A. Yassin, Chem. Heterocycl. Compd., 2009, 45(1), 35–41 CrossRef.
- J. K. Son, L. X. Zhao, A. Basnet, P. Thapa, R. Karki, T. C. Jeong, B. S. Jeong, C. S. Lee and E. S. Lee, Eur. J. Med. Chem., 2008, 43, 675–682 CrossRef PubMed.
- B. Vacher, B. Bonnaud, P. Funes, N. Jubault and M. Kleven, J. Med. Chem., 1999, 42, 1648–1660 CrossRef PubMed.
- M. T. Cocco, C. Congiu and V. Onnis, Eur. J. Med. Chem., 2003, 38, 37–47 CrossRef PubMed.
- A. Abadi, O. Al-Deeb, A. Al-Afify and H. El-Kashef, Farmaco, 1999, 54, 195–201 CrossRef PubMed.
- G. Ozturk, D. D. Erol, T. Uzbay and M. D. Aytemir, Farmaco, 2001, 56, 251–256 CrossRef PubMed.
- P. Storck, A. Aubertin and D. S. Grierson, Tetrahedron Lett., 2005, 46, 2919–2922 CrossRef.
- P. S. Ghosh, K. Manna, U. Banik, M. Das and P. Sarkar, Int. J. Pharm. Pharm. Sci., 2014, 6, 39–42 Search PubMed.
- F. F. Fleming, L. Yao, P. C. Ravikumar, L. Funk and B. C. Shook, J. Med. Chem., 2010, 53, 7902–7917 CrossRef PubMed.
- J. M. Mirkovi, D. Z. Mijin and S. D. Petrovic, Hem. Ind., 2013, 67, 17–25 CrossRef.
- T. Murata, K. Shimizu, V. C. Manganiello, T. Tagawa and K. Okumura, Anticancer Drugs, 2002, 13(8), 875–880 CrossRef.
- I. W. Cheney, S. Yan, T. Appleby, H. Walker, R. Hamatake and Z. Hong, Bioorg. Med. Chem. Lett., 2007, 17(6), 1679–1683 CrossRef PubMed.
- N. A. Aqui and R. H. Vonderheide, Cancer Biol. Ther., 2008, 7(12), 1888–1889 CrossRef PubMed.
- F. Hernández, A. Sánchez, P. Rendón-Vallejo and F. Delgado, Eur. J. Med. Chem., 2013, 70, 669–676 CrossRef PubMed.
- L. Carles, K. Narkunan, S. Penlou, L. Rousset, D. Bouchu and M. A. Ciufolini, J. Org. Chem., 2002, 67, 4304–4308 CrossRef PubMed.
- Z. Kibou, N. Cheikh, D. Villemin, N. Choukchou-Braham, B. Mostefa-Kara and M. Benabdallah, Int. J. Org. Chem., 2011, 1, 242–249 CrossRef.
- D. Mijin and A. Marinkovic, Synth. Commun., 2006, 36, 193–198 CrossRef.
- S. K. Rai, S. Khanam, R. S. Khanna and A. K. Tewari, RSC Adv., 2014, 4, 44141–44145 RSC.
- M. Seifi and H. Sheibani, Lett. Org. Chem., 2013, 10, 478–481 CrossRef.
- L. Mosti, G. Menozzi, P. Schenone, P. Dorigo, R. M. Gaion and P. Belluco, Farmaco, 1992, 47, 427 Search PubMed.
- I. A. Ibarra, A. Islas-Jácome and E. González-Zamora, Org. Biomol. Chem., 2018, 16, 1402–1418 RSC.
- G. Mari, M. Verboni, L. D. Crescentini, G. Favi, S. Santeusanio and F. Mantellini, Org. Chem. Front., 2018, 5, 2108–2114 RSC.
- X. Chang, X. Zhang and Z. Chen, Org. Biomol. Chem., 2018, 16, 4279–4287 RSC.
- G. L. Wu and Q. P. Wu, Adv. Synth. Catal., 2018, 360, 1949–1953 CrossRef.
- A. H. Mostafa-Hussein, Heteroat. Chem., 1997, 8, 1–6 CrossRef.
- M. R. H. Elmoghayar and A. G. A. El-Agamey, J. Heterocycl. Chem., 1984, 21, 1885 CrossRef.
Footnote |
† Electronic supplementary information (ESI) available. See DOI: 10.1039/c8ra05690k |
|
This journal is © The Royal Society of Chemistry 2018 |