DOI:
10.1039/C8RA05280H
(Paper)
RSC Adv., 2018,
8, 31889-31894
A novel off-on fluorescent chemosensor for Al3+ derived from a 4,5-diazafluorene Schiff base derivative†
Received
20th June 2018
, Accepted 21st August 2018
First published on 12th September 2018
Abstract
The performance of a chemosensor is closely related to its structure. A new Schiff bass (DFSB) based on 4,5-diazafluorene units has been synthesized in this work. The interaction of DFSB with different metal ions has been studied using UV-vis absorption spectra and fluorescent spectra. The results show that DFSB is a highly selective and sensitive probe for Al3+ ions over other commonly coexisting metal ions in ethanol. A very obvious fluorescence enhancement effect was observed, and a turn-on ratio over 1312-fold was triggered with the addition of 10 equiv. of Al3+ ions. What is more, such fluorescent responses could be detected by the naked eye under a UV-lamp. The lowest detection limit for Al3+ was determined as 3.7 × 10−8 M. The complex solution (DFSB–Al3+) exhibited reversibility with EDTA. These results may be caused by the unique molecular structure.
1 Introduction
Al3+ ions existing in natural waters and most plants can enter the human body through foods and water.1 The normal concentration range for aluminum ions in biological systems is narrow, with both deficiency and excess causing many pathological states, such as Alzheimer's disease, Parkinson's disease, chronic renal failure, bone softening and smoking related diseases.2–6 Since there is a close association between Al3+ and human health, developing fluorescent chemosensors with high selectivity and sensitivity for detecting trace amounts of Al3+ has attracted increasing attention.
In recent years, chemosensors have attracted significant interest because of their high sensitivity, selectivity, rapid response7,8 and simplicity9 and have been widely used in many fields such as public health,10–12 metal ion detection,13–16 environmental monitoring17–19 and disease diagnosis. Incorporation of specific functional structure unit into the fluorescent chemosensors leads to advanced functional materials that exhibit certain advantageous properties, such as highly selective and sensitive sensor for analysis.20 In addition, fluorescent sensors based on a large group of structurally different fluorophores, including coumarin,21,22 8-hydroxyquinoline,23 naphthalene,24 rhodamine,25–27 pyrrolidine,28 calixarene,29 hydroxyflavone,30,31 thiazole,32 oxazoline and imidazoline33 have been developed. These sensors have sufficiently high selectivity. However, for many of them, the fluorescent response and detection limit of a determined cation is not sufficient. For example, the sensor based on julolidine shows only 7.8-fold fluorescence buildup by reaction with Zn2+ ions.34 Therefore, the aim of determining simple structure and obtaining fluorescent chelating ligands capable of acting both as a selective chemical reagent and an “off–on” fluorescent cation sensor is very relevant.
4,5-Diazafluorene is one of the most powerful building blocks for creating multifunctional structures. These compounds have similarities to 2,2′-bipyridyl and 1,10-phenanthroline derivatives.35 Furthermore, these compounds can form various complexes by coordination to metal ions such as Fe3+,36 Cu2+,37,38 Cd2+,39 Eu3+,40 and Ru2+.41,42 The 4,5-diazafluorene has been chosen as an ideal structure of a fluorescent chemosensor due to its large π-system and bidentate coordination ability with various metal ions. Some Ru(II) polypyridyl complexes containing 4,5-diazafluorene units has been employed as fluorescent probes for metal ions.43–45 However, the fluorescent chemosensors based on the binding ability of 4,5-diazafluorene units to metal ions is rarely.46,47
Herein, we report the synthesis and characterization of a Schiff base ligand (DFSB) obtained by condensing 9,9-bis(4-aminophenyl)-4,5-diazafluorene with salicylaldehyde. The Al3+ recognition ability were investigated. The free chemosensor DFSB shows weak fluorescence emission. Upon binding of Al3+, a significant fluorescence enhancement over 1314-fold is achieved in ethanol.
2 Experiment
2.1 Materials and instrumentation
The reactants 4,5-diazafluoren-9-one (1)48 was prepared according to the literature. Aniline (Tianjin Guangfu) was purified by distillation prior to use. All organic solvents were of analytical reagent grade. Nitrate and chloride salt of all cations used were of high purity and used without any purification.
The stock solution for DFSB (3 mM) were prepared in DMSO, stock solution for nitrate and chloride salt of different metals of Ag+, Al3+, Ba2+, Ca2+, Cd2+, Co2+, Cr3+, Cu2+, Fe2+, Fe3+, Hg2+, K+, Li+, Mg2+, Mn2+, Na+, Ni2+, Sr2+, Pb2+ and Zn2+ were prepared by dissolving the salts in deionized water to the final concentrations of 1.0 mM. The absorption and fluorescence titrations were performed on 10.0 μM solutions of DFSB in ethanol and the aliquots of freshly prepared standard aqueous solutions of metal ions were added to record the absorption and fluorescence spectra. Each spectrum was recorded 40 min after Al3+ addition.
The 1H NMR and 13C NMR spectra were measured on a JEOL EX-400 spectrometer using DMSO-d6 as solvent and tetramethylsilane as the internal reference. UV-vis spectra were examined on a Lambda 35 spectrophotometer and fluorescent recorded on a LS-55 spectrofluorophotometer with excitation slit at 15.0 nm and emission at 7.0 nm.
2.1.2 Determination of detection limit. The detection limits (DLs) of DFSB for Al3+ were calculated using the following equation:
where σ is the standard deviation of the blank measurement, which is 0.077 here, and S is the slope between the emission intensity vs. the concentration of aluminium ion.
2.2 Monomer synthesis
2.2.1 Synthesis of 9,9-bis(4-aminophenyl)-4,5-diazafluorene (2). 9,9-bis(4-aminophenyl)-4,5-diazafluorene was synthesized by accordingly to describe the method in Scheme 1. Methanesulfonic acid (4.04 g, 41 mmol) was slowly added to the aniline (19.56 g, 210 mmol) was placed in a 100 ml three-necked flask with magnetic stirring and a nitrogen inlet. Then, 4,5-diazafluoren-9-one (5.45 g, 30 mmol) was added. The mixture was the heated to 155 °C for 24 h under an atmosphere of nitrogen with continuous stirring. The solution was cooled below 80 °C followed by neutralization with a solution of 5% sodium hydroxide. The precipitate was filtered and washed with water and ethanol. The crude product was purified by recrystallization to give a white powder (4.04 g, yield: 59%), mp: >300 °C.
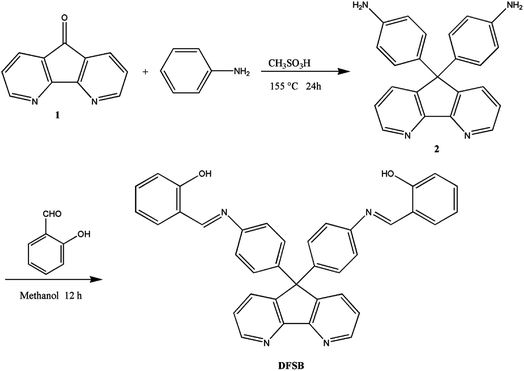 |
| Scheme 1 Synthesis of the Schiff base DFSB. | |
IR (KBr, cm−1): 3454 (NH), 3331 (NH), 3066–3004 cm−1 (C–N), 1510 cm−1 (C
N); 1H NMR (400 MHz, DMSO-d6, δ, ppm, TMS, Fig. S1†): 5.03 (s, 2H, –NH2), 6.42 (d, H2, J = 8.4 Hz, 2H), 6.75 (d, H3, J = 8.4 Hz, 2H), 7.35 (dd, H8, J = 4.8, 4.8 Hz), 7.84 (dd, H7, J = 1.6, 1.6 Hz, 2H), 8.62 (dd, H9, J = 1.6, 1.6 Hz, 2H); 13C NMR (100 MHz, DMSO-d6, δ, ppm, TMS, Fig. S1†): 59.8 (C5), 114.1 (C2), 123.9 (C8), 128.5 (C3), 131.0 (C6), 134.2 (C7), 147.5 (C4), 148.0 (C1), 149.7 (C9), 157.1 (C10).
2.2.2 Synthesis of 4,5-diazafluorene Schiff bases (DFSB). 9,9-bis(4-aminophenyl)-4,5-diazafluorene (0.175 g, 0.5 mmol) was placed into a 100 ml round-bottom flask which was fitted with condenser, thermometer and magnetic stirrer. Methanol (50 ml) was added into the flask and reaction mixture was heated up to 80 °C. A solution of salicylaldehyde (0.183 g, 1.5 mol) in 20 ml methanol was added into the flask. Reactions were maintained for 12 h under reflux. The precipitated monomers was filtered hot and dried in a vacuum desiccator. (0.24 g, yield: 85.7%). 1H NMR (DMSO-d6, δ, ppm, TMS, Fig. S2†): 12.95 (s, –OH), 8.91 (s, H7, J = 1.2 Hz, 2H), 8.74 (dt, H16, J = 4.8, 1.2 Hz, 2H), 8.12–8.04 (dt, H14, J = 7.8, 1.4 Hz, 2H), 7.63 (dd, H5, J = 7.8, 1.4 Hz, 2H), 7.48 (m, H3, 2H), 7.41 (tt, H15, J = 3.1, 2.4 Hz, 2H), 7.35 (dd, H9, J = 8.6, 1.3 Hz, 4H), 7.25 (dd, H10, J = 8.5, 1.2 Hz, 4H), 7.00–6.92 (m, H2 and H,4 4H). 13C NMR (DMSO-d6, δ, ppm, TMS, Fig. S2†): 164.18 (C1), 160.78 (C7), 157.33 (C17), 150.66 (C8), 147.89 (C16), 145.83 (C11), 142.58 (C14), 134.59 (C3), 133.90 (C13), 133.05 (C5), 129.16 (C10), 124.48 (C9), 122.24 (C4), 119.83 (C15), 119.67 (C6), 117.12 (C2), 60.83 (C12).
3 Result and discussion
3.1 Absorption spectra study of receptor DFSB
As seen in Fig. 1, the absorption spectrum of receptor DFSB exhibited four absorbance peaks. The intense absorption band in high energy region at 233 nm and a small hump at 258 nm was assigned as the absorption overlap of ligand-to-ligand-charge-transfer (LLCT) transitions,49 Also, a broad band at 320 to 350 nm could be assigned to π → π* transition of the C
N group from 4,5-diazafluorene moiety.50 Upon addition of Al3+ ions, a significant change is observed in the UV-vis absorption spectra of DFSB, the absorption band of DFSB at 258 nm decreased with concomitant evolution of a new small hump at 251 nm; meanwhile, the broad band at 320 to 350 nm decreased with concomitant evolution of a new broad peak at 307 nm are assigned as MLCT absorptions. Clear isosbestic points at 241 nm, 263 nm and 380 nm are noticeable, which indicates the formation of only one active DFSB–Al3+ complex (Fig. 2). In order to further validate the stoichiometry of the receptor DFSB and Al3+, Job's plot was carried out. The concentration dependent studies further confirmed the formation of 1
:
1 coordination complex between Al3+ and DFSB (Fig. S3†). Thus, the presumed binding mode of DFSB–Al3+ complex was given in the Fig. 2.
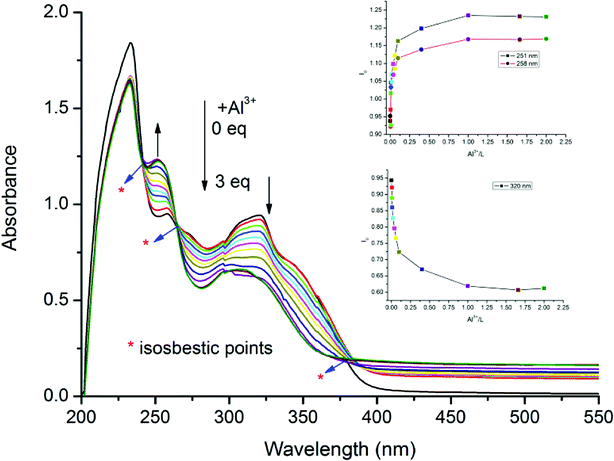 |
| Fig. 1 UV-vis spectra of receptor DFSB (10 μM) in aqueous ethanol upon addition of increasing concentration of Al3+. Inset: a plot of absorbance intensity as estimated by the peak height at 251 nm, 258 nm and 320 nm. | |
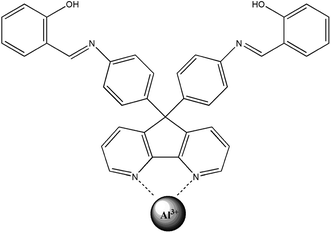 |
| Fig. 2 The presumed binding mode of DFSB-Al3+ complex. | |
3.2 Fluorescence study of Al3+
The fluorescence behavior of DFSB is canvassed upon the addition of various metal ions in ethanol. As shown in Fig. 3a, receptor DFSB alone displayed a very weak single fluorescence emission intensity at 475 nm (about 0.66) with an excitation 392 nm. Upon addition of other cations (Ag+, Ba2+, Ca2+, Cd2+, Co2+, Cr3+, Cu2+, Fe2+, Fe3+, Hg2+, K+, Li+, Mg2+, Mn2+, Na+, Ni2+, Sr2+, Pb2+ and Zn2+) no significant changes were observed except Mg2+ (about 51.5). But on addition of 10 equivalents of Al3+ ions, the fluorescence intensity of DFSB (10 μM) increased rapidly which shows the high selectivity of Al3+ ions. The fluorescence enhancement efficiency observed at 475 nm was 1312-fold greater than the control in the absence of Al3+ (Fig. 3b). In the absence of Al3+, the extent of intramolecular charge transfer (ICT) in DFSB was sufficient enough to enhance its fluorescence. The chelation of DFSB with Al3+ not only enhanced the ICT effect in DFSB but also increased the rigidity of the molecular assembly resulting in a significant enhancement of the fluorescence intensity which is known as chelation-enhanced fluorescence (CHEF).51 Based on the use of a UV lamp, the solution of receptor DFSB showed a dramatic color change from colorless to fluorescent blue in the presence of Al3+ ion, which could easily be detected by the naked-eye (Fig. 3c).
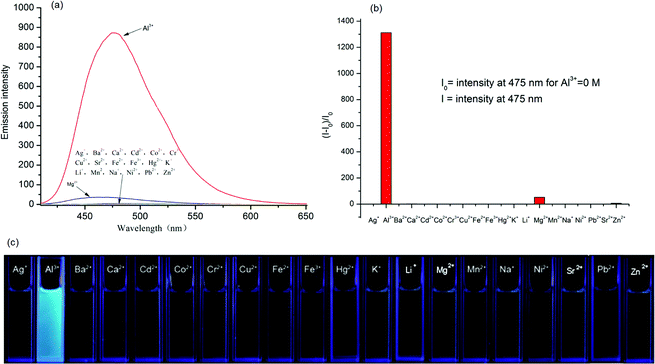 |
| Fig. 3 (a) The fluorescence spectra of the DFSB (10 μM) in the presence of 10 equiv. of various metal ions in ethanol. Excitation wavelength (nm): 392. (b) Fluorescence emission response profiles for DFSB (10 μM) by adding various metal ions in ethanol. (c) Visual fluorescence emissions of sensor DFSB after addition of Ag+, Al3+, Ba2+, Ca2+, Cd2+, Co2+, Cr3+, Cu2+, Fe2+, Fe3+, Hg2+, K+, Li+, Mg2+, Mn2+, Na+, Ni2+, Pb2+, Sr2+ and Zn2+ (10 equiv.) in ethanol on excitation at 365 nm using UV lamp at room temperature. | |
In order to establish the specific selectivity of DFSB to Al3+, we performed the single and dual metal competitive analysis, as shown in Fig. 4. In a single metal system (black bars), all the metal ions (Ag+, Ba2+, Ca2+, Cd2+, Co2+, Cr3+, Cu2+, Fe2+, Fe3+, Hg2+, K+, Li+, Mg2+, Mn2+, Na+, Ni2+, Sr2+, Pb2+ and Zn2+) concentrations were kept as 100 μM towards DFSB. However, for the dual-metal (red bars) studies, two equal amounts of aqueous solutions of Al3+ and other metal ions (100 μM + 100 μM) were combined. From the bar diagram (Fig. 4), the PL intensity of the DFSB shows no obvious changes except for Fe3+. Therefore, it was clear that other ions interference was negligibly small which were attributed to the unique structure of the DFSB. The unique selectivity of DFSB towards Al3+ could be interpreted in terms of the smaller ionic radii (0.5 Å) and higher charge density (r = 4.81) of the Al3+. The smaller radii of the Al3+ permits a suitable coordination geometry of the chelating receptor DFSB and the larger charge density allows a strong coordination ability between DFSB and Al3+.24
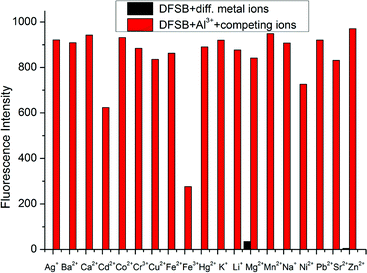 |
| Fig. 4 Fluorescence response of receptor DFSB (10 μM) containing 10 equiv. of Al3+ to the selected metal ions (100 μM). The black bar represents emission intensity after adding 10 μM selected metal ions (Ag+, Al3+, Ba2+, Ca2+, Cd2+, Co2+, Cr3+, Cu2+, Fe2+, Fe3+, Hg2+, K+, Li+, Mg2+, Mn2+, Na+, Ni2+, Pb2+, Sr2+ and Zn2+) in ethanol solution and red bar represent emission intensity after adding 10 μM of Al3+ in each of the above samples. Excitation wavelength (nm): 392. | |
Fluorescence titration experiments were carried out to investigate the complexation of Al3+ with the receptor DFSB. With an increasing concentration of Al3+, the intensity of the maximum emission at 475 nm increased gradually (Fig. 5a). Using the calibration graph (Fig. 5b), it was possible to determine Al3+ in ethanol up to 30 μM. The calibration curve is linear with a correlation coefficient, R2 = 0.956. From the fluorescent titration data, the detection limits was also measured to be 3.7 × 10−8 M (Fig. S4†) in ethanol. Moreover, the dissociation constants of DFSB–Al3+ was calculated to be 1.8 × 109 M (Fig. S4†).
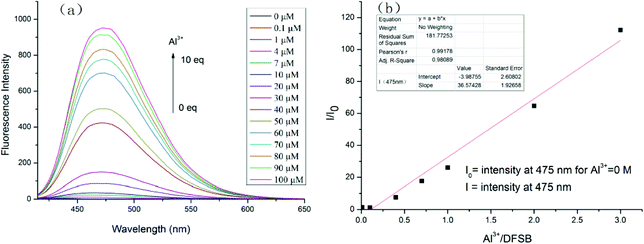 |
| Fig. 5 (a) Fluorescence emission spectra of receptor DFSB (10 μM) in ethanol solution upon addition of increasing concentration of Al3+. From bottom to top: [Al3+] = (0, 0.1, 1, 4, 7, 10, 20, 30, 40, 50, 60, 70, 80, 90, 100 μM); (b) plot of fluorescence intensity at 475 nm versus the number of equivalents of Al3+ added. Excitation wavelength (nm): 392. | |
As shown in Fig. 6, the reversibility of sensor DFSB has been studied by the titration of EDTA with fluorescent probes (DFSB + Al3+). The DFSB has very weak fluorescence emission intensity in ethanol. Upon adding Al3+ ions, the fluorescence intensity increases significantly. The fluorescence emission intensity returned to lower level for sensor DFSB after addition of EDTA. These results show that the addition of EDTA can completely destroyed the DFSB–Al3+ complex. This may be due to the coordination constant between EDTA–Al3+ is greater than DFSB–Al3+.
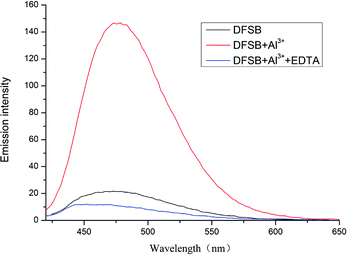 |
| Fig. 6 Fluorescence emission spectra of receptor DFSB (10 μM) in the presence of Al3+ ions (30 μM) or EDTA (10 μM) in ethanol. Excitation wavelength (nm): 392. | |
4 Conclusion
In conclusion, A novel off-on fluorescent chemosensor for Al3+ derivated from 4,5-diazafluorene Schiff base derivative has been developed. The probe shows great fluorescence turn-on upon binding Al3+ and gives fluorescence enhancement by about 1312-fold at 475 nm. Moreover, the sensory system shows bright blue colour with Al3+ under a UV lamp, which can be easily identified by the naked eye. Thus, the reported sensor shows potential for detection of Al3+ using fluorescence spectroscopy.
Conflicts of interest
There are no conflicts to declare.
Acknowledgements
The authors are grateful for the research support from the National Natural Science Foundation of China [No. 21204033], China Postdoctoral Science Foundation (2017M612520), National Science Foundation of Hubei Province of China [2016CFB264, 2018CFB108] and the Program of Hubei Provincial Department of Education, China [Q20171507].
References
- G. Berthon, Coord. Chem. Rev., 1996, 149, 241–280 CrossRef.
- G. R. Legendre and A. C. Alfrey, Clin. Chem., 1976, 22, 53–56 Search PubMed.
- D. P. Perl and A. R. Brody, Science, 1980, 208, 297–299 CrossRef PubMed.
- D. P. Perl, D. C. Gajdusek, R. M. Garruto, R. T. Yanagihara and C. J. Gibbs, Science, 1982, 217, 1053–1055 CrossRef PubMed.
- M. H. Mashhadizadeh and H. Khani, Anal. Methods, 2010, 2, 24–31 RSC.
- L. Fan, J. Qin, T. Li, B. Wang and Z. Yang, J. Lumin., 2014, 155, 84–88 CrossRef.
- V. K. Gupta, B. Sethi, R. A. Sharma, S. Agarwal and A. Bharti, J. Mol. Liq., 2013, 177, 114–118 CrossRef.
- V. K. Gupta, L. P. Singh, R. Singh, N. Upadhyay, S. P. Kaur and B. Sethi, J. Mol. Liq., 2012, 174, 11–16 CrossRef.
- E. V. Antina, N. A. Bumagina, A. I. V'Yugin and A. V. Solomonov, Dyes Pigm., 2017, 136, 368–381 CrossRef.
- H. Karimi-Maleh, F. Tahernejad-Javazmi, N. Atar, M. L. Yola, V. K. Gupta and A. A. Ensafi, Ind. Eng. Chem. Res., 2015, 54, 3634–3639 CrossRef.
- V. K. Gupta, A. Nayak, S. Agarwal and B. Singhal, Comb. Chem. High Throughput Screening, 2011, 14, 284–302 CrossRef PubMed.
- M. L. Yola, V. K. Gupta, T. Eren, A. E. Şen and N. Atar, Electrochim. Acta, 2014, 120, 204–211 CrossRef.
- V. K. Gupta, N. Mergu, L. K. Kumawat and A. K. Singh, Sens. Actuators, B, 2015, 207, 216–223 CrossRef.
- S. K. Srivastava, V. K. Gupta and S. Jain, Anal. Chem., 1996, 68, 1272–1275 CrossRef PubMed.
- S. K. Srivastava, V. K. Gupta and S. Jain, Analyst, 1995, 120, 495–498 RSC.
- S. K. Srivastava, V. K. Gupta, M. K. Dwivedi and S. Jain, Anal. Proc., 1995, 32, 21–23 RSC.
- V. K. Gupta, H. Karimi-Maleh and R. Sadegh, Int. J. Electrochem. Sci., 2015, 10, 303–316 Search PubMed.
- S. Karthikeyan, V. K. Gupta, R. Boopathy, A. Titus and G. Sekaran, J. Mol. Liq., 2012, 173, 153–163 CrossRef.
- M. H. Dehghani, D. Sanaei, I. Ali and A. Bhatnagar, J. Mol. Liq., 2016, 215, 671–679 CrossRef.
- V. K. Gupta, M. R. Ganjali, P. Norouzi, H. Khani, A. Nayak and S. Agarwal, Crit. Rev. Anal. Chem., 2011, 41, 282–313 CrossRef PubMed.
- B. Sen, S. K. Sheet, R. Jamatia, A. K. Pal, R. Thounaojam, K. Aguan and S. Khatua, Spectrochim. Acta, Part A, 2017, 173, 537–543 CrossRef PubMed.
- J. Li, Y. Zeng, Q. Hu, X. Yu, J. Guo and Z. Pan, Dalton Trans., 2012, 41, 3623–3626 RSC.
- N. Lashgari, A. Badiei and G. Mohammadi Ziarani, J. Fluoresc., 2016, 26, 1885–1894 CrossRef PubMed.
- A. Sahana, A. Banerjee, S. Das, S. Lohar, D. Karak, B. Sarkar, S. K. Mukhopadhyay, A. K. Mukherjee and D. Das, Org. Biomol. Chem., 2011, 9, 5523–5529 RSC.
- V. K. Gupta, N. Mergu and L. K. Kumawat, Sens. Actuators, B, 2016, 223, 101–113 CrossRef.
- V. K. Gupta, N. Mergu, L. K. Kumawat and A. K. Singh, Talanta, 2015, 144, 80–89 CrossRef PubMed.
- J. B. Li, H. Q. Hu, Y. Zeng, X. L. Yu and Z. Q. Pan, Prog. Chem., 2012, 24, 823–833 Search PubMed.
- Y. Wang, L. Xiong, F. Geng, F. Zhang and M. Xu, Analyst, 2011, 136, 4809–4814 RSC.
- A. B. Othman, J. W. Lee, Y.-D. Huh, R. Abidi, J. S. Kim and J. Vicens, Tetrahedron, 2007, 63, 10793–10800 CrossRef.
- R. S. Sathish, A. G. Raju, G. N. Rao and C. Janardhana, Spectrochim. Acta, Part A, 2008, 69, 282–285 CrossRef PubMed.
- M. M. Karim, S. H. Lee, Y. S. Kim, H. S. Bae and S. B. Hong, J. Fluoresc., 2006, 16, 17–22 CrossRef PubMed.
- V. K. Gupta, A. K. Singh and L. K. Kumawat, Sens. Actuators, B, 2014, 195, 98–108 CrossRef.
- A. Jeanson and V. Béreau, Inorg. Chem. Commun., 2006, 9, 13–17 CrossRef.
- Y. S. Kim, G. J. Park, J. J. Lee, S. Y. Lee, S. Y. Lee and C. Kim, RSC Adv., 2015, 5, 11229–11239 RSC.
- K. Ono and K. Saito, Heterocycles, 2008, 75, 2381–2413 CrossRef.
- P. Kulkarni, S. Padhye and E. Sinn, Inorg. Chem. Commun., 2003, 6, 1129–1132 CrossRef.
- G. Li, N. Liu, S. Liu and S. Zhang, Electrochim. Acta, 2008, 53, 2870–2876 CrossRef.
- G.-J. Li, N. Liu, P.-K. Ouyang and S.-S. Zhang, Oligonucleotides, 2008, 18, 269–276 CrossRef PubMed.
- B. Machura, I. Nawrot and K. Michalik, Polyhedron, 2012, 31, 548–557 CrossRef.
- Z. Liu, F. Wen and W. Li, Thin Solid Films, 2005, 478, 265–270 CrossRef.
- K. Ocakoglu, C. Zafer, B. Cetinkaya and S. Icli, Dyes Pigm., 2007, 75, 385–394 CrossRef.
- K. Sako, T. Kakehi, S. Nakano, H. Oku, X. F. Shen, T. Iwanaga, M. Yoshikawa, K. Sugahara, S. Toyota and H. Takemura, Tetrahedron Lett., 2014, 55, 749–752 CrossRef.
- F. Cheng, N. Tang and X. Yue, Spectrochim. Acta, Part A, 2009, 71, 1944–1951 CrossRef PubMed.
- F. Cheng, Y. Sun, W. Wu and N. Tang, Inorg. Chem. Commun., 2008, 11, 687–690 CrossRef.
- Y. Zhao, Z. Lin, S. Ou, C. Duan, H. Liao and Z. Bai, Inorg. Chem. Commun., 2006, 9, 802–805 CrossRef.
- H. Li, S. Zhang, C. Gong, J. Wang and F. Wang, J. Fluoresc., 2016, 26, 1555–1561 CrossRef PubMed.
- S. J. Zhang, H. Li, C. L. Gong, J. Z. Wang, Z. Y. Wu and F. Wang, Synth. Met., 2016, 217, 37–42 CrossRef.
- I. Eckhard and L. Summers, Aust. J. Chem., 1973, 26, 2727–2728 CrossRef.
- L. Zhang and B. Li, Inorg. Chim. Acta, 2009, 362, 4857–4861 CrossRef.
- T. Harel, N. Shefer, Y. Hagooly and S. Rozen, Tetrahedron, 2010, 66, 3297–3300 CrossRef.
- B. Liu, B.-S. Yang, P.-F. Wang, J. Chai, X.-Q. Hu, T. Gao, T.-G. Chen and J.-B. Chao, Spectrochim. Acta, Part A, 2016, 168, 98–103 CrossRef PubMed.
Footnote |
† Electronic supplementary information (ESI) available. See DOI: 10.1039/c8ra05280h |
|
This journal is © The Royal Society of Chemistry 2018 |
Click here to see how this site uses Cookies. View our privacy policy here.