DOI:
10.1039/C8RA04823A
(Paper)
RSC Adv., 2018,
8, 30616-30623
Metabolomics analysis based on a UPLC-Q-TOF-MS metabolomics approach to compare Lin-Xia-Shan-Shen and garden ginseng†
Received
6th June 2018
, Accepted 8th August 2018
First published on 30th August 2018
Abstract
Background: Panax ginseng Meyer which has been cultivated and grown naturally in mountainous forests is formally called “Lin-Xia-Shan-Shen” (LXSS), but when cultivated it is called garden ginseng (GG), according to the Chinese Pharmacopoeia (2015 edition). The medicinal value of LXSS is significantly higher than that of GG based on the clinical experience of TCM. This study aimed to evaluate the variety of chemical constituents in LXSS and GG. Methods: 18 LXSS and 6 GG samples were investigated using a UPLC-Q-TOF-MS technique. Results: the contents of 16 metabolites, mainly involved in the biosynthesis of rare ginsenosides (ginsenosides Rg3, -Rh1, -Rh2), galactose metabolism (myo-inositol), the citric cycle (citric acid and succinic acid), GABA shunt (GABA) and amino metabolism (alanine and aspartic acid), were higher in LXSS than in GG; while 14 metabolites, mainly involved in starch and sucrose metabolism (fructose and sucrose), amino metabolism (tryptophan, proline, dencichine and pyroglutamic acid) and campesterol biosynthesis (campesterol), were lower in LXSS than in GG. For LXSS with different growing years, 5 metabolites showed a tendency to increase dependent on the number of years, and these were related mainly to galactose metabolism (melibiose), the citric cycle (malic acid), fatty acid metabolism (2-hydroxy stearic acid); while 5 metabolites showed a tendency to decrease on ascending the grades, and these were related to sucrose metabolism (fructose and sucrose), fatty acid metabolism (2-hydroxy hexadecanoic acid) and campesterol biosynthesis (campesterol). Conclusion: this proposed analytical method coupled with multivariate analysis is fast, accurate, and reliable for discriminating GG and high cultivation ages of LXSS samples.
1. Introduction
With its restorative and tonic effects, ginseng is traditionally used as a panacea in Chinese medicine.1,2 Various chemical constituents in ginseng include ginsenosides, polysaccharides, amino acids and microelements,3 which are responsible for its multiple bioactivities and restorative functions, such as strengthening of the immune response and cardiovascular protection, as well as antidiabetes, anti-cancer, anti-obesity and anti-oxidation activities.4,5 These active components in ginseng accumulate gradually over time and show a certain regularity.6–8 In addition, ginsenosides are influenced by the growth environment, including hours of sunshine9 and soil.10,11 “Lin-Xia-Shan-Shen” (LXSS) is derived from plants grown naturally in mountainous forests after artificial seeding, whereas garden ginseng (GG) is manually cultivated ginseng, according to the Chinese Pharmacopoeia (2015 edition). The similar appearance of LXSS to wild ginseng grown for a longer number of years is also one of the characteristics that make it attractive in the culture of traditional Chinese medicine and its medicinal value is significantly higher than that of GG according to clinical experience in TCM. At present, the price of LXSS in the market is about ten times that of GG.12 Owing to its lower number of cultivation years, convenient administration and higher production, GG is still the main source of ginseng products. It is also one of the main causes of adulteration in the market. Therefore, a reliable method to discriminate the cultivation ages of ginseng is required for the quality control of this medicinal herb and the prevention of its adulteration in the market.
Previous studies indicated that the contents of the chief constituents (ginsenosides and polysaccharides) in LXSS were higher than those in GG.6,13 The change in ginsenosides is related to numbers of years of cultivation and the origin of the ginseng.14,15 And the contents of ginsenosides are also different in different parts of the ginseng.16
However, these studies were mostly limited to the difference between GG and lower cultivation years of LXSS (<10 years), and paid little attention to the difference between GG and higher cultivation years of LXSS (>10 years). Meanwhile, few studies attempted to reveal the alterations in constituent metabolites between GG and LXSS based on an LC-MS platform. None of these works searched for a correlation between primary metabolites and the ginsenoside network within GG and LXSS to posit key views in the regulation of ginsenoside biosynthesis.
Recently, the new ‘omics’ approaches have been widely used in modern biology. Metabolomics, a fine combination of analytical and statistical techniques, is one of the latest additions to the ‘omics’ wave, and has been used to unravel the complex of primary and secondary metabolites fluctuating in the whole plant and the comprehensive metabolite network flux associated with various plant species and with specific tissues.17 Metabolomics approaches involving liquid chromatography mass spectrometry (LC-MS), gas chromatography mass spectrometry (GC-MS), and nuclear magnetic resonance (NMR) have been used to discriminate and analyze the metabolites of ginseng.18–22 The cultivation age of GG is usually about 6 years, but the cultivation age of LXSS is generally greater than 10 years in the market. But because of diseases and pests, the cultivation age of LXSS is usually no more than 20 years.23 In this paper, therefore, 6 GG samples and 18 LXSS samples (of three different subgroups: 13 years, 15 years and 20 years) were selected and used to compare the various chemical constituents of LXSS (high cultivation years) and GG by an UPLC-Q-TOF-MS metabolomics approach. On the one hand, this proposed analytical method coupled with multivariate analysis is fast, accurate, and reliable for discriminating LXSS and GG and is a potential tool for standardizing quality control in the ginseng industry. On the other hand, we followed an interest in the interactive links between alterations in ginsenosides and primary metabolites between LXSS and GG, which offered a new train of thought about the mechanisms of ginsenoside biosynthesis at the metabolite level.
2. Materials and methods
2.1 Materials
Eighteen samples of LXSS (three samples each growing for 13 years, 15 years or 20 years) and six samples of GG were used in the study. The GG samples were purchased from Anhui Monteggia Chinese Herbal Medicine Co., Ltd. (no: 131009; Anhui, China) and the LXSS samples were purchased from Benxi, Liaoning Province and were identified by Bing Wang (Professor of Liaoning University of Traditional Chinese Medicine).
2.2 Chemicals and reagents
HPLC-grade acetonitrile, methanol and MS-grade formic acid were purchased from Merck (Merck, Germany). Ultra-pure water was treated with a Milli-Q water purification system (Millipore, France) and other reagents were analytical grade. The standards of ginsenosides Ra3, -Rb1, -Rc, -Rd, -Re, -Rf, -Rg1, -20(S)Rg3, -Rh1, -20(S)F1, -Rh2, -F3 and pseudo-ginsenoside F11 were purchased from the Chinese Medical and Biological Products Institute (Beijing, China). Malic acid, malt sugar, citric acid, malonic acid, adenosine and amino acid mixed standards were purchased from Sigma (USA). GABA, dencichine, inositol and glucuronic acid and pyruvic acid were purchased from the Aladdin Industrial Corporation (Shanghai, China). Fumaric acid was purchased from TCI (Tokyo Industrial Co., Ltd, Japan).
2.3 Liquid chromatography
Chromatographic separation was performed on an Acquity UPLC HSS T3 column (2.1 mm × 100 mm, 1.7 μm, Waters Corp., Milford, USA) and an BEH Amide column (2.1 mm × 100 mm, 1.7 μm, Waters Corp., Milford, USA) using a Waters Acquity UPLC system, equipped with a binary solvent delivery system. The column was maintained at 45 °C and eluted at a flow rate of 0.3 ml min−1, using separate mobile phases of (A) 0.1% (by volume) formic acid in water and (B) methanol, and (A) water and (B) acetonitrile. The gradient programs were optimized as follows: T3 column, 0 → 1 min: 0% B; 1 → 3 min: 0% → 20% B; 3 → 13 min: 20%→50% B; 13 → 15 min: 100% B; 15 → 16 min: 100% B; 16 → 16.1 min: 100% → 0% B; 16.1 → 18 min: 0% B, BEH Amide column, 0 → 1.5 min: 0% B; 1.5 → 8 min: 0% → 50% B; 8 → 8.1 min: 50% → 0% B; 8.1 → 10.5 min; curve: 6. The column eluent was directed to the mass spectrometer without splitting.
2.4 Mass spectrometry
Mass spectrometry was performed on a Waters Xevo G2 QTOF quadrupole accelerated time-of-flight mass spectrometer (Waters Corp., Manchester, USA) with an electrospray ionization source (ESI) operating separately in positive and negative ion modes (T3 column) and positive ion mode (amide column). The MS condition is described in ESI Table 1.†
2.5 Sample preparation
Reference compounds were dissolved in 60% aqueous methanol to obtain stock solutions at approximately 1.0 mg ml−1, and they were stored at 4 °C.
For the UPLC-Q-TOF MS analyses, ginseng samples were extracted by an optimized method for detecting diverse ginseng metabolites.24,25 Briefly, the roots of the ginseng samples were pulverized, and approximately 30 mg of the ground sample was accurately weighed and ultrasonically-extracted three times with 0.6 ml of 60% aqueous methanol for 30 min at room temperature, and centrifuged at 1200 rpm for 10 min. The supernatants were merged and transferred to a 2 ml volumetric flask, 60% aqueous methanol added to volume, and mixed. The mixed solutions were filtered through a 0.22 μm PTFE syringe filter, and 3 μl of each filtrate was subjected to UPLC-Q-TOF-MS/MS analysis. To validate the stability of the established method, an in-house QC sample was prepared by mixing an equal volume (100 μl) from each sample, which was injected after every 6 samples during the whole sample analysis.26
2.6 Multivariate statistical analysis
To gain a comprehensive assessment of the quality and to explore the discriminating variables of LXSS and GG, all the UPLC-Q-TOF-MS data were recorded by Markerlynx 4.1 software. All processed data of the ginseng were analyzed by multivariate statistical methods, such as PLS-DA and hierarchical clustering analysis (HCA). PLS-DA analysis was employed to determine the specific variation between LXSS (growing for 13 years, 15 years or 20 years) and GG groups. The potentially interesting metabolites were identified and selected by using the cutoff of the PLS-DA Variable Importance in Projection (VIP) score, using the criteria that the VIP should be larger than 1 and p should be less than 0.05, using the Statistical Package for Social Science program (SPSS 20.0, Chicago, IL, USA).
2.7 Metabolite identification and metabolic annotations
Potential biomarkers were identified with available reference standards by matching their retention time and accurate mass measurement with the databases METLIN (http://metlin.scripps.edu/), HMDB (http://www.hmdb.ca/) and KEGG (http://www.genome.jp/kegg/). The database MetPA (http://metpa.metabolomics.ca./MetPA/faces/Home.jsp), based on database sources including the METLIN, HMDB, KEGG and SMPD (http://www.smpdb.ca/), was used to perform the ingenuity pathway analysis (IPA).
3. Results and discussion
3.1 Identifying and analyzing potential biomarkers
The PLS-DA analysis was used to distinguish the difference between GG and LXSS and to look for potential marker compounds in GG and LXSS. The data from the positive ion mode (T3 column), the negative ion mode (T3 column), the positive ion mode (amide column) and for all three models were analyzed by PLS-DA, and are shown in ESI Fig. 1–3† and 1. In the PLS-DA score plots, a clear separation was observed between GG and LXSS (ESI Fig. 1–3†). The R2Y and Q2 (cum) of the newly established PLS-DA model were 0.997 and 0.987 in the positive ion mode (T3 column), 0.998 and 0.993 in the negative ion mode (T3 column), and 0.759 and 0.690 in the positive ion mode (amide column), respectively, and the values of R2 and Q2 are shown in ESI Fig. 4–6,† indicating that the model showed great fitness and prediction. In order to comprehensively analyze the constituents in ginseng, all the data from the three models were analyzed further by PLS-DA in this study (Fig. 1). The reproducibility of the UPLC-Q-TOF-MS was determined from four replicated analyses of the same quality control sample (QC) interspersed throughout the analysis.
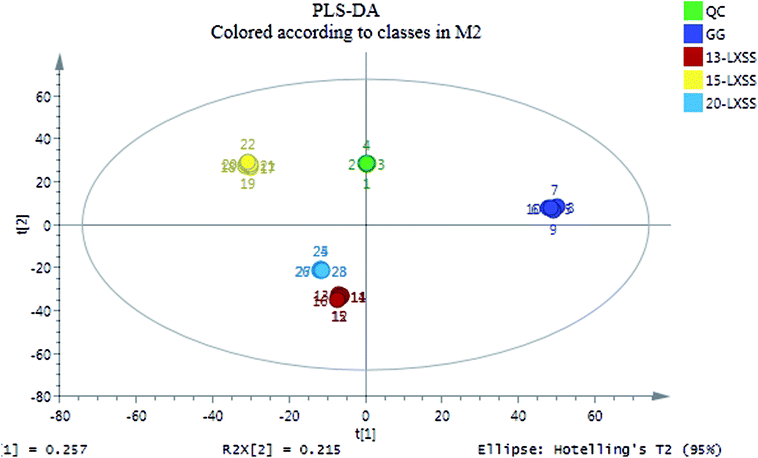 |
| Fig. 1 PLS-DA scores plot of different ginseng groups. | |
QC samples play an important role in untargeted metabolomic studies.27 Generally, the platform-dependent acceptance criterion of a QC sample is as follow: RSD < 20% for UPLC-MS.28–30 In this paper, the RSDs of the peak area of all the metabolites in the QC samples are below 20%, demonstrating good stability and reproducibility throughout the entire sequence of sample runs. Metabolic profiling was acquired in the positive and negative ion modes of the different columns. The QC samples clustered together tightly in the score plot of PLS-DA, which indicated that system stability was suitable for this metabolomics study (Fig. 1).
In the study, 46 significantly different biomarkers were identified on the basis of accurate elemental compositions and in the context of their retention times compared with available reference standards and the available database (ESI Table 2†). 34 biomarkers (numbers: 1–34, shown in ESI Table 3†) were confirmed with available reference standards by matching their retention times and accurate mass measurements. To further understand the metabolic differences between GG and LXSS, a clustering heatmap was generated for all identified biomarkers, demonstrating their relative increase (red) or decrease (green). In Fig. 2, the distance between GG and LXSS was related to the degree of similarity between them and the results indicated that GG was close to 13-LXSS, followed by 15-LXSS and 20-LXSS.
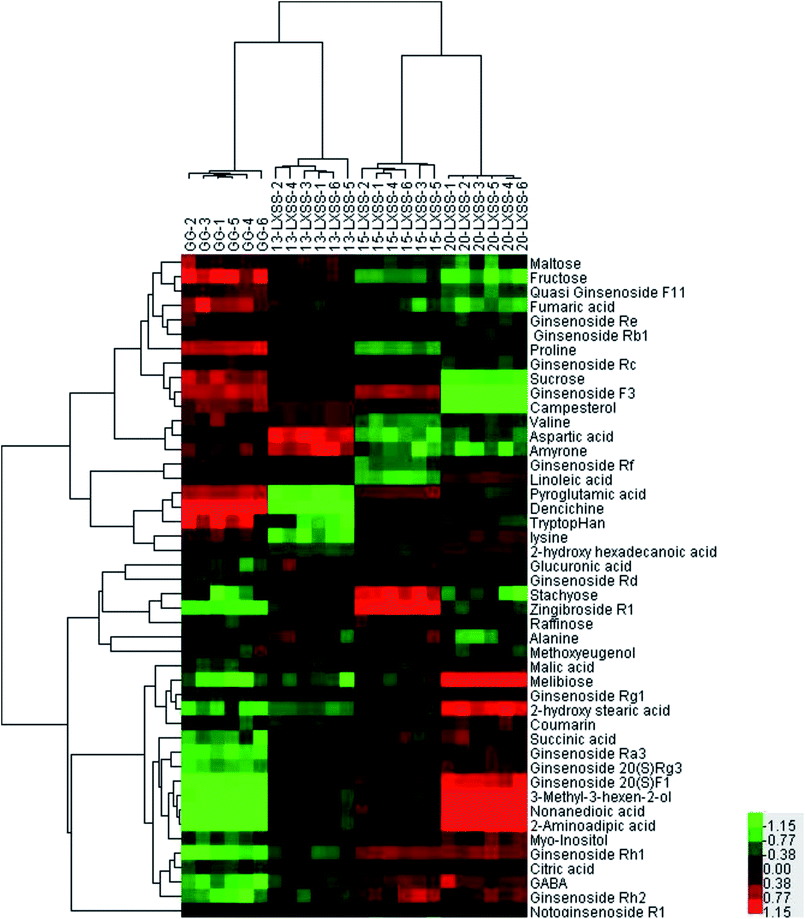 |
| Fig. 2 Heatmap of identified metabolites in LXSS and GG groups a clustering heatmap was generated for all identified biomarkers, demonstrating the relative increase (red) or decrease (green). | |
3.2 An integrative analysis of potential metabolites
Based on database sources, including MetPA, METLIN, HMDB, KEGG and SMPD and the molecular structures of ginsenosides (ESI Table 4†), a metabolic network in light of the identified primary metabolites and ginsenosides was drawn up to reveal their tight interlinking (Fig. 3), which made clear the internal differences between LXSS and GG. Fig. 3 shows that 12 primary metabolites, three sugars, seven amino acids and two acids differed significantly between GG and LXSS. Thus, these compounds act as the best discriminating factors between LXSS and GG. Since the primary compounds have different roles in different tissues,31 this may be the main reason why ginsenosides show different significant accumulations in a tissue-specific way. In addition, there was a significant accumulation of rare ginsenosides in LXSS, including ginsenosides Rg3, -Rh1, -Rh2, and a significant accumulation of campesterol and β-amyrone in GG, which explained the phenomenon of ginsenosides being lower in GG than in LXSS.
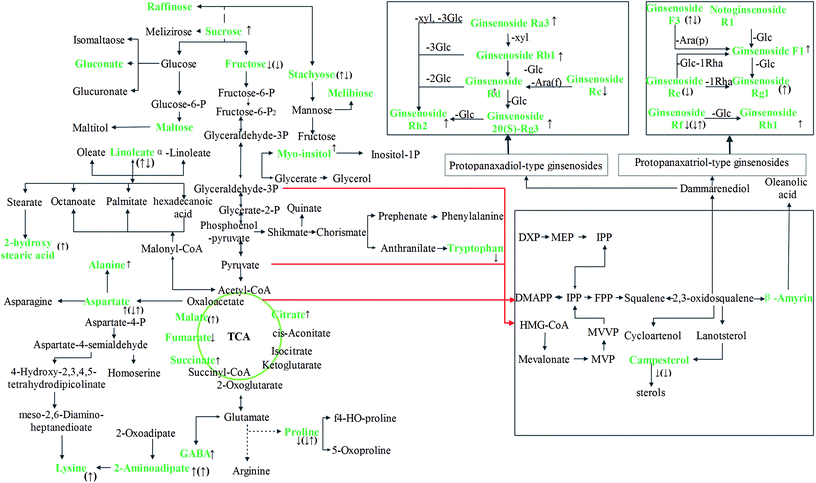 |
| Fig. 3 Visualization of primary and secondary metabolite dynamics in biochemical pathway mapcompounds with green font are the metabolites. Metabolites with ↑ present significant increase in LXSS compared to GG. Metabolites with ↓ present significant decrease in LXSS compared to GG. Metabolite with (↑) present significantly increase as the rise of year in LXSS with different growing years. Metabolite with (↓) present significantly decrease as the rise of year in LXSS with different growing years. Metabolite with (↑↓) present significantly first increase then decrease as the rise of year in LXSS with different growing years. Metabolites with (↓↑) present significantly first decrease then increase as the rise of year in LXSS with different growing year. | |
3.2.1 Ginsenosides. GG is cultivated ginseng and its optimal harvesting year is approximately year six. However, LXSS is cultivated for at least 10 years and 15 years is very common. In the study, therefore, LXSS samples were selected from 13 years, 15 years and 20 years. Previous research indicated an UHPLC-Q-TOF-MS based metabolomic approach could be used to discriminate different cultivation ages of P. ginseng (from 1 to 6 years).24 In this study, the results indicated that there was no distinction between the types of ginsenosides in GG and LXSS; however, their contents were different. 15 ginsenosides were obtained and the data for molecular structure are exhibited in ESI Table 3.† The contents of 7 metabolites in LXSS, ginsenosides Ra3, -Rd, -20(S)Rg3, -Rh1, -Rh2, -20(S)F1 and notoginsenoside R1, were higher than those in GG; while the contents of 6 metabolites in LXSS, ginsenosides Rc, -Rb1, -Re, -Rf, -F3 and pseudo-ginsenoside F11, were lower than those in GG; of these, ginsenosides Rg3, -Rh1 and -Rh2 are rare. As for LXSS with different growing years, ginsenoside Rg1 showed a tendency to increase dependent on the number of years; while pseudo-ginsenoside F11 and campesterol showed a tendency to decrease as the grades were ascended. Meanwhile, the results indicated that the contents of ginsenoside Rb1, ginsenoside Rh2, notoginsenoside R1, ginsenoside Re, ginsenoside Rc, and ginsenoside F3 were the highest and the content of ginsenoside Rf was the lowest in LXSS grown for 15 years than for the other years; thus, 15 years was a turning point for the accumulation of these components in LXSS. Among these, the results of the change in ginsenoside Rb1 and ginsenoside Re in LXSS were the same as in a previous study.23 Considering the difference in ginsenosides for different growing years of LXSS, LXSS should be rationally planted and harvested, to obtain the maximum benefits of industrialization.Further, analysis of the molecular structure and biosynthetic pathway of ginsenosides was performed and the results indicated that rare ginsenosides, such as ginsenosides 20(S)Rg3, -Rh1 and Rh2, showed a significant accumulation in LXSS, which is a result of geographic and environmental factors and the duration of cultivation. The glucosidic bond of the primary ginsenoside was hydrolyzed by microorganisms or the respective enzyme, and gradually transformed into the secondary ginsenoside or rare ginsenoside (Fig. 3). Now, it is known that 2,3-oxidized squalene in ginseng has four metabolic pathways: two ginsenoside biosynthesis pathways (dammarane ginsenoside and β-amyrin), sterol biosynthesis and lupeol biosynthesis. The biosynthesis of campesterol and β-amyrone belongs to the ginsenoside biosynthesis type of pathway, so it can compete with dammarane ginsenoside biosynthesis (Fig. 3). Therefore, the accumulation of campesterol and β-amyrone in GG could explain this phenomenon from the point of view that the content of ginsenoside is lower in GG than in LXSS.
3.2.2 Sugars, amino acids and other organic metabolites. Carbon metabolism, such as sugar metabolism, glycolysis, the TCA cycle and shikimate-phenylpropanoid metabolism, are responsible for the production of accessible energy, resistant metabolites and carbon skeletons for plant life during growth and development.32 Carbon metabolism is closely related to plant growth and senescence, and it can include carbon assimilation, carbon transformation and accumulation among various plant physiological processes.33 Eight metabolites of the respective sugars were obtained in the study. The contents of fructose and sucrose were significantly higher in GG than in LXSS, but myo-inositol was significantly lower in LXSS; and this could be used to distinguish between GG and LXSS. The contents of fructose, maltose and sucrose in LXSS grown for different years showed a significant tendency to increase as the grades were ascended, whereas the contents of myo-inositol and melibiose showed a decrease; and the level of stachyose was highest in the 15 year LXSS than in the other years. Sucrose is used as a transport sugar for entry into glycolysis and the TCA cycle for the production of ATP and NADH.34 Fructose is a monosaccharide and a degradation product of sucrose. Significant accumulations of fructose and sucrose were detected in GG, indicating that the shift from C assimilation to accumulation occurred more in GG than in LXSS. This may be due to environmental factors such as hours of sunshine.9 The former experiences more hours of sunshine than the latter. And the accumulation of ginsenosides in LXSS requires more sugar. Significant accumulation of myo-inositol was detected in LXSS; myo-inositol is a substrate of raffinose, playing an important role in the metabolism of plants and having multiple functions. In addition, myo-inositol is the precursor of cell wall polysaccharides, such as glucuronic acid and pentose units, which play an important role in external and non-biological stress.35 LXSS was planted in the wild and suffered more external and non-biological stress and the cell wall became gradually incrassated. Therefore, the level of myo-inositol in ginseng was higher than in GG and increased as the number of growing years increased. Raffinose and stachyose are mainly soluble sugars in the seeds, roots and rhizomes and they effect the transport of sugar in plant phloem.36 To meet sugar metabolism, raffinose and stachyose represented a significant change in LXSS with different growth years in the study. Succinic acid, citric acid and fumaric acid are important intermediate products of the TCA cycle. The results indicated that succinic acid and citric acid were substantially more abundant in LXSS compared with GG, but fumaric acid was significantly decreased in LXSS. On the one hand, the TCA cycle is the main energy source and LXSS needed more energy than GG to respond to external and non-biological stress. Previous studies indicated that the increase in the synthesis, accumulation, transport and secretion of organic acids in plants is a response by plants to environmental stress.37,38 Therefore, environmental stress may lead to the production of abundant organic acid in LXSS. And, the content of citric acid in LXSS was higher than that of other organic acids, which may be related to its ability to inactivate pathogenic microorganisms.39Nitrogen metabolisms (e.g., amino acid metabolism) are basic physiological mechanisms for the synthesis and decomposition of nitrogenous compounds in plants.40 Amino acids, as important energy metabolites and synthetic precursors of various bioactive molecules, are divided into aromatic, aliphatic and heterocyclic amino acids based on their chemical structures. Compared to GG, 8 biomarkers were obtained and could be used to distinguish GG and LXSS. Among them, the content of GABA, alanine and aspartic acid were higher in LXSS; however, tryptophan, arginine, pyroglutamic acid, dencichine and proline were lower in LXSS. As for LXSS with different growing years, the contents of lysine and GABA showed tendencies to increase as the grades were ascended, whereas the contents of pyroglutamic, decichine and valine showed a trend of rising first then falling or falling first then rising in LXSS grown for 15 years. As a response to environmental stresses, GABA production often increases a lot.41 An adverse growing environment is the main reason for the GABA increase in LXSS.
Fatty acid metabolism is an important plant metabolism. Fatty acids are the constituent part of membrane lipids, the source of energy and the precursor of some signal molecules in plants.42 Previous research indicated that the desaturation of fatty acids in ginseng containing 20% saturated fatty acid and 80% unsaturated fatty acid had effects on cold and disease resistance, which was an important component of the plant's defense reaction. Linoleic acid shows the highest level of fatty acids in ginseng, and the highest level of linoleic acid was detected in LXSS grown for 20 years compared to the others in the study. Compared to GG, 3-methyl-3-hexen-2-ol, nonanedioic acid and 2-aminoadipate were up-regulated in LXSS. The cells of the phellem layer were arranged flatter and more tightly in LXSS than in GG, which limited moisture loss and resisted the invasion of microorganisms. As for LXSS grown for different numbers of years, 2-hydroxy stearic acid showed a tendency to increase dependent of the number of years; while 2-hydroxy hexadecanoic acid showed a tendency to decrease as the grades were ascended. Volatile oil of ginseng is the signal conduction substance for phytoalexins and florescence, and it has a significant influence on ginseng output. The results indicated that 3-methyl-3-hexen-2-ol showed significant accumulation in LXSS.
4. Conclusion
Metabolomic approaches are now widely used in evaluating and identifying ginseng. In this study, a UPLC-Q-TOF-MS metabolomics approach was used to compare the various chemical constituents of LXSS and GG. 30 metabolites can be used to identify GG and LXSS and 10 metabolites can be used to identify LXSS growing for different numbers of years. Further, a metabolic network in light of the identified primary metabolites and ginsenosides was drawn up to reveal their tight interlinking. We found that metabolites mainly involved in sugar metabolism, amino acid metabolism, fatty acid metabolism and energy metabolism existed in GG and LXSS, which may be due to environmental stress and the duration of cultivation, which explained the differences in metabolites between LXSS and GG at the metabolite level and can provide a reference for the reasonable planting and quality control of ginseng.
Conflicts of interest
None of the authors declare any conflicts of interests in this study.
Acknowledgements
This project was supported by the funding of the National Natural Science Foundation of China, Grant 81773858 and 81370095, the Fundamental Research Funds for the Central Public Welfare Research Institutes (Project number zz2017004) and also Beijing Key Laboratory of Research of Chinese Medicine on Prevention and Treatment for Major Diseases.
References
- C. P. Commission, Chinese Pharmacopoeia (2015 edition), Chinese Medical Science Press, 2015 Search PubMed.
- L. Jia, Y. Zhao and X. J. Liang, Current Evaluation of the Millennium Phytomedicine-Ginseng (II): Collected Chemical Entities, Modern Pharmacology, and Clinical Applications Emanated from Traditional Chinese Medicine, Curr. Med. Chem., 2009, 16, 2924 CrossRef PubMed.
- V. A. Assinewe, B. R. Baum, D. Gagnon and J. T. Arnason, Phytochemistry of Wild Populations of Panax quinquefolius L. (North American Ginseng), J. Agric. Food Chem., 2003, 53, 4549–4553 CrossRef PubMed.
- L. Eunjeong, R. Shaykhutdinov, A. M. Weljie, H. J. Vogel and P. J. Facchini, Quality assessment of ginseng by 1H NMR metabolite fingerprinting and profiling analysis, J. Agric. Food Chem., 2009, 57, 7513–7522 CrossRef PubMed.
- M. Rotblatt, Herbal Medicine: Expanded Commission E Monographs, Ann. Intern. Med., 2000, 133, 487 CrossRef.
- J. K. Zhang, R. Gao, D. Q. Dou and T. G. Kang, The ginsenosides and carbohydrate profiles of ginseng cultivated under mountainous forest, Pharmacogn. Mag., 2013, 9, 38–43 CrossRef PubMed.
- L. Zhang, W. Gao, S. Zhou and N. Cai, Application of UPLC to Separation and Analysis of Ginsenosides from Cultivated Ginseng and Forest-grown Wild Ginseng, Lat. Am. J. Pharm., 2011, 30, 991–995 Search PubMed.
- R. Ma, L. Sun, X. Chen, R. Jiang, H. Sun and D. Zhao, Proteomic changes in different growth periods of ginseng roots, Plant Physiol. Biochem., 2013, 67, 20–32 CrossRef PubMed.
- J. H. Argyroudi-Akoyunoglou, Z. Feleki and G. Akoyunoglou, Formation of two chlorophyll-protein complexes during greening of etiolated bean leaves, Biochem. Biophys. Res. Commun., 1971, 45, 606–614 CrossRef PubMed.
- Y. Zhao, X. Gu, L. Wu and W. You, Researches on categories, characteristics, and utilization value of cultivated ginseng germplasm resources, Chin. Tradit. Herb. Drugs, 2007, 38, 294–296 Search PubMed.
- Y. Jin, Study on the growth and development of ginseng and the environmental conditions of saponin accumulation, Jilin Agricultural University, 2003 Search PubMed.
- S. Xu, K. Li, D. Shi and Q. Sun, Study on characteristics and microscopic characteristics of wild ginseng, wild ginseng under forest, unimplanted ginseng, and cultivated ginseng, Chin. Tradit. Herb. Drugs, 2013, 44, 2304–2307 Search PubMed.
- Z. X. Zhou, Y. Qu, D. Q. Dou, F. Y. Liu, Y. S. Huo and F. B. Jiao, The ginsenoside profile of ginseng cultivated under mountainous forest, J. Med. Plants Res., 2011, 5, 410–419 Search PubMed.
- A. Wang, C. Z. Wang, J. A. Wu, J. Osinski and C. S. Yuan, Determination of major ginsenosides in Panax quinquefolius (American ginseng) using high-performance liquid chromatography, Phytochem. Anal., 2005, 16, 272–277 CrossRef PubMed.
- M. Mizuno, J. Yamada, H. Terai, N. Kozukue, Y. S. Lee and H. Tsuchida, Differences in immunomodulating effects between wild and cultured Panax ginseng, Biochem. Biophys. Res. Commun., 1994, 200, 1672–1678 CrossRef PubMed.
- W. Shi, Y. Wang, J. Li, H. Zhang and L. Ding, Investigation of ginsenosides in different parts and ages of Panax ginseng, Food Chem., 2007, 102, 664–668 CrossRef.
- W. Weckwerth, Metabolomics in systems biology, Annu. Rev. Plant Biol., 2003, 54, 669 CrossRef PubMed.
- E. C. Chan, S. L. Yap, A. J. Lau, P. C. Leow, D. F. Toh and H. L. Koh, Ultra-performance liquid chromatography/time-of-flight mass spectrometry based metabolomics of raw and steamed Panax notoginseng, Rapid Commun. Mass Spectrom., 2007, 21, 519–528 CrossRef PubMed.
- J. Kang, S. Lee, S. Kang, H. N. Kwon, J. H. Park, S. W. Kwon and S. Park, NMR-based metabolomics approach for the differentiation of ginseng (Panax ginseng) roots from different origins, Arch. Pharmacal Res., 2008, 31, 330–336 CrossRef PubMed.
- L. Eunjeong, R. Shaykhutdinov, A. M. Weljie, H. J. Vogel and P. J. Facchini, Quality assessment of ginseng by 1H NMR metabolite fingerprinting and profiling analysis, J. Agric. Food Chem., 2009, 57, 7513–7522 CrossRef PubMed.
- Y. Wang, J. Y. Pan, X. Y. Xiao, R. C. Lin and Y. Y. Cheng, Simultaneous determination of ginsenosides in Panax ginseng with different growth ages using high-performance liquid chromatography-mass spectrometry, Phytochem. Anal., 2010, 17, 424–430 CrossRef.
- G. Xie, R. Plumb, M. Su, Z. Xu, A. Zhao, M. Qiu, X. Long, Z. Liu and W. Jia, Ultra-performance LC/TOF MS analysis of medicinal Panax herbs for metabolomic research, J. Sep. Sci., 2008, 31, 1015–1026 CrossRef PubMed.
- Z. You, Quality evaluation of Linxia shen, Jilin Agricultural University, 2007 Search PubMed.
- N. Kim, K. Kim, B. Y. Choi, D. Lee, Y. S. Shin, K. H. Bang, S. W. Cha, J. W. Lee, H. K. Choi and D. S. Jang, Metabolomic approach for age discrimination of Panax ginseng using UPLC-Q-Tof MS, J. Agric. Food Chem., 2011, 59, 10435–10441 CrossRef PubMed.
- J. Liu, Y. Liu, Y. Wang, A. Abozeid, Y. G. Zu and Z. H. Tang, The integration of GC-MS and LC-MS to assay the metabolomics profiling in Panax ginseng and Panax quinquefolius reveals a tissue- and species-specific connectivity of primary metabolites and ginsenosides accumulation, J. Pharm. Biomed. Anal., 2017, 135, 176–185 CrossRef PubMed.
- T. Sangster, H. Major, R. Plumb, A. J. Wilson and I. D. Wilson, A pragmatic and readily implemented quality control strategy for HPLC-MS and GC-MS-based metabonomic analysis, Analyst, 2006, 131, 1075–1078 RSC.
- W. B. Dunn, I. D. Wilson, A. W. Nicholls and D. Broadhurst, The importance of experimental design and QC samples in large-scale and MS-driven untargeted metabolomic studies of humans, Bioanalysis, 2012, 4, 2249 CrossRef PubMed.
- W. B. Dunn, D. Broadhurst, P. Begley, E. Zelena, S. Francis-Mcintyre, N. Anderson, M. Brown, J. D. Knowles, A. Halsall and J. N. Haselden, Procedures for large-scale metabolic profiling of serum and plasma using gas chromatography and liquid chromatography coupled to mass spectrometry, Nat. Protoc., 2011, 6, 1060–1083 CrossRef PubMed.
- E. Zelena, W. B. Dunn, D. Broadhurst, S. Francis-Mcintyre, K. M. Carroll, P. Begley, S. O'Hagan, J. D. Knowles, A. Halsall and H. Consortium, Development of a robust and repeatable UPLC-MS method for the long-term metabolomic study of human serum, Anal. Chem., 2009, 81, 1357–1364 CrossRef PubMed.
- P. Begley, S. Francismcintyre, W. B. Dunn, D. I. Broadhurst, A. Halsall, A. Tseng, J. Knowles, H. Consortium, R. Goodacre and D. B. Kell, Development and Performance of a Gas Chromatography–Time-of-Flight Mass Spectrometry Analysis for Large-Scale Nontargeted Metabolomic Studies of Human Serum, Anal. Chem., 2009, 81, 7038 CrossRef PubMed.
- H. Tan, Q. Xie, X. Xiang, J. Li, S. Zheng, X. Xu, H. Guo and W. Ye, Dynamic Metabolic Profiles and Tissue-Specific Source Effects on the Metabolome of Developing Seeds of Brassica napus, PLoS One, 2015, 10, e0124794 CrossRef PubMed.
- A. Nunes-Nesi, A. R. Fernie and M. Stitt, Metabolic and Signaling Aspects Underpinning the Regulation of Plant Carbon Nitrogen Interactions, Mol. Plant, 2010, 3, 973–996 CrossRef PubMed.
- Y. Zhao, J. Zhao, C. Zhao, H. Zhou, Y. Li, J. Zhang, L. Li, C. Hu, W. Li and X. Peng, A metabolomics study delineating geographical location-associated primary metabolic changes in the leaves of growing tobacco plants by GC-MS and CE-MS, Sci. Rep., 2015, 5, 16346 CrossRef PubMed.
- A. Sturm, Invertases. Primary Structures, Functions, and Roles in Plant Development and Sucrose Partitioning, Plant Physiol., 1999, 121, 1–7 CrossRef PubMed.
- H. Yin, W. Wang and X. Zhao, Research Progress in Plant Glycobiology, Chinese Bulletin of Botany, 2010 Search PubMed.
- L. I. Fang and X. F. Wang, Advance in Raffinose Family Oligosaccharides Metabolism and the Key Enzymes in Plants, Acta Bot. Boreali-Occident. Sin., 2008, 28, 852–859 Search PubMed.
- F. Zhang, V. Römheld and H. Marschner, Effect of zinc deficiency in wheat on the release of zinc and iron mobilizing root exudates, J. Plant Nutr. Soil Sci., 1989, 152, 205–210 Search PubMed.
- C. Huang, M. J. Webb and R. D. Graham, Pot size affects expression of Mn efficiency in barley, Plant Soil, 1996, 178, 205–208 CrossRef.
- H. E. Park, S. Y. Lee, S. H. Hyun, d. Y. Kim, P. J. Marriott and H. K. Choi, Gas chromatography/mass spectrometry-based metabolic profiling and differentiation of ginseng roots according to cultivation age using variable selection, J. AOAC Int., 2013, 96, 1266–1272 CrossRef PubMed.
- H. Tschoep, Y. Gibon, P. Carillo, P. Armengaud, M. Szecowka, A. Nunes-Nesi, A. R. Fernie, K. Koehl and M. Stitt, Adjustment of growth and central metabolism to a mild but sustained nitrogen-limitation in Arabidopsis, Plant, Cell Environ., 2009, 32, 300 CrossRef PubMed.
- A. M. Kinnersley and F. J. Turano, Gamma aminobutyric acid (GABA) and plant responses to stress, Crit. Rev. Plant Sci., 2000, 19, 479–509 CrossRef.
- L. U. Shan_Fa,Biosynthesis and Gene Engineering of Plant Fatty Acids, Chinese Bulletin of Botany, 2000 Search PubMed.
Footnote |
† Electronic supplementary information (ESI) available. See DOI: 10.1039/c8ra04823a |
|
This journal is © The Royal Society of Chemistry 2018 |
Click here to see how this site uses Cookies. View our privacy policy here.