DOI:
10.1039/C8RA04550J
(Paper)
RSC Adv., 2018,
8, 26523-26527
Direct N-heterocyclization of hydrazines to access styrylated pyrazoles: synthesis of 1,3,5-trisubstituted pyrazoles and dihydropyrazoles†
Received
28th May 2018
, Accepted 2nd July 2018
First published on 24th July 2018
Abstract
A microwave-assisted method has been developed for the synthesis of tri-substituted pyrazoles via direct N-heterocyclization of hydrazines with metal-acetylacetonate and -dibenzylideneacetonate without using any base or additives. Most importantly, the synthesis of 1-aryl-5-phenyl-3-styryl-1H-pyrazoles was achieved in a single step using hydrochloride salt of various phenylhydrazines and this is the first report for direct construction of these molecules. The reaction medium and microwave conditions play a critical role for their selective product formation during the reaction. The present reaction explored the usage of metal-diketonic complexes as reaction substrates providing acetylacetone and dibenzylideneacetone moieties to directly participate in cyclization with hydrazines to form the corresponding pyrazoles in excellent yields. The present protocol introduces the important N-heterocyclic moieties in the final structures, giving the reaction great applications from a medicinal chemistry perspective, particularly in the late stage modification strategies in drug discovery.
1. Introduction
Pyrazoles are very important functional moieties having extensive applications in pharmaceuticals,1 agriculture,2 supramolecular chemistry,3 polymers,4 liquid crystals,5 ligands in catalysis6 and many others. Despite the intricacy in N–N bond formations, these are widely present in nature7 such as in withasomnine, pyrazofurin, formycin A and B, nostocine A, fluviol A–E, and pyrazole-3(5)-carboxylic acid and show remarkable biological potential. 1,3,5-Trisubstituted pyrazoles constitute a number of drugs,1i,8 including sildenafil (inhibits phosphodiesterase), celecoxib (selective COX-2 inhibitor used for inflammation and arthritis), rimonabant (inverse agonist for cannabinoid receptor CB1 and anti-obesity drug) and difenamizole (analgesic). In recent times, these are one of the privileged scaffolds in drug discovery and are extensively targeted by synthetic chemists. A number of methods have been developed to construct pyrazole scaffolds.9 Traditionally, 1,3,5-trisubstituted pyrazoles are synthesized by cyclocondensation of hydrazines with diketones under acidic conditions,10 aromatization of pyrazolines11 and intramolecular coupling of hydrazones.12
Recently, pyrazoles have been synthesized from imine hydrazine precursors (I, II) by cyclization using metal catalyst and oxidants or base13 and from addition of hydrazones by α-bromo ketones using light irradiation.14
Among 1,3,5-trisubstituted pyrazoles, the synthesis of 1-aryl-5-phenyl-3-styryl-1H-pyrazoles require pre-installed pyrazolines (III) as a substrate15 for further oxidation to pyrazoles (Scheme 1). To date, we could not come across any report in our thorough literature search for the direct synthesis of 1-aryl-5-phenyl-3-styryl-1H-pyrazoles (IV) in one step. The electrocyclization of dibenzylideneacetones with hydrazines leads to the synthesis of pyrazolines (III) in the presence of a catalyst (Scheme 1).16 Metal-acetylacetonates [M(acac)2] and metal-dibenzylideneacetones [M2(dba)3 or M(dba)2] are very powerful reagents in organic synthesis and catalysis. However, these metal complexes have not been used till date as a partner substrate for the construction of pyrazoles. As envisioned, herein, we disclose a general method for N-heterocyclization of different arylhydrazines with metal-diketonic complexes to access 1,3,5-trisubstituted pyrazoles, namely, 1-aryl-5-phenyl-3-styryl-1H-pyrazoles (IV), 3,5-dimethyl-N-aryl-1H-pyrazoles (V), 3,5-dimethyl-N-sulfonyl aryl-1H-pyrazoles (VI) and 1,3-diphenyl-5-styryl-4,5-dihydro-1H-pyrazole (III), under neutral conditions using microwave irradiations (Scheme 1).
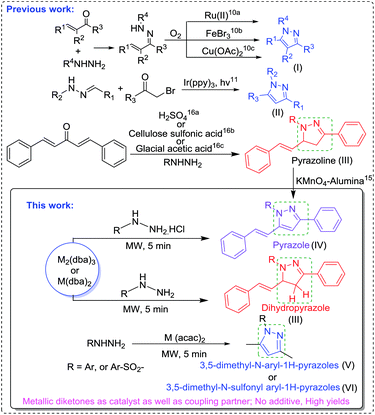 |
| Scheme 1 Synthesis of pyrazoles and dihydropyrazoles. | |
2. Results and discussion
To investigate the optimized conditions for the synthesis of styryl substituted pyrazoles (A), we choose phenyl hydrazine hydrochloride as a model substrate. The reaction of model substrate with Pd2(dba)3 was performed in different solvents under microwave irradiation at 100 °C and 50 watt for 5 min (Table 1). Reaction with phenylhydrazine hydrochloride in H2O gave both pyrazole as well as dihydropyrazole in approximately equal amounts (Table 1, entry 1). However, interestingly, in the case of phenyl hydrazine substrate (without hydrochloride salt), the reaction gave styryl-substituted dihydropyrazole (B) as the major product (Table 1, entry 9). DMSO afforded the desired styryl-substituted pyrazole in high yield (Table 1, entry 2). In case of DMF, the reaction did not give pyrazole (A) product, but only 10% of dihydropyrazole (B) was observed (Table 1, entry 3).
Table 1 Optimization of conditions for formation of styryl pyrazolesa

|
Entry |
Solvent |
MW (watts) |
Yieldb [%] |
A |
B |
Reaction conditions: phenyl hydrazine hydrochloride (1.0 mmol), Pd2(dba)3 (0.5 equiv.), solvent (1 mL), MW (watts), 100°C, for 5 min. Isolated yields. Reaction with phenyl hydrazine (no HCl salt). |
1 |
H2O |
50 |
30 |
25 |
2 |
DMSO |
50 |
80 |
5 |
3 |
DMF |
50 |
0 |
10 |
4 |
MeOH |
50 |
20 |
10 |
5 |
PEG-200 |
50 |
5 |
8 |
6 |
CHCl3 |
50 |
15 |
0 |
7 |
THF |
50 |
20 |
6 |
8 |
Toluene |
50 |
10 |
0 |
9c |
H2O |
50 |
5 |
89 |
Reaction in CHCl3 and toluene selectively provided the pyrazole (A) product. However, very less conversion was observed (Table 1, entries 6 and 8). Other solvents such as MeOH, PEG-200 and THF also did not provide promising yields and selectivity of the desired pyrazole (A) product (Table 1, entries 4, 5 and 7).
Thereafter, we investigated the scope for an important finding in the present report, i.e. direct synthesis of 1-aryl-5-phenyl-3-styryl-1H-pyrazoles from the reaction of phenyl hydrazine hydrochlorides (Table 2). The reaction of trifluoromethoxy, nitrile, methoxy and trifluoro methyl-substituted hydrazines afforded 1-aryl-5-phenyl-3-styryl-1H-pyrazoles in good yields (Table 2, 2a–2d) with Pd2(dba)3 using DMSO as a solvent instead of H2O. Halogenated (Cl, F, Br) phenylhydrazines also gave the desired product in high yields (Table 2, 2e–2j). The reaction of phenylhydrazine hydrochloride proceeded well under optimised conditions (Table 2, 2k).
Table 2 Synthesis of pyrazoles and dihydropyrazolesabcd
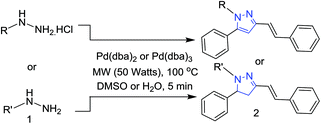
|
Reaction conditions: hydrazine (1.0 mmol), Pd(dba)3 (0.5 equiv.), MW (50 watts), 100 °C, for 5 min. Isolated yields. DMSO (1 mL) was used as solvent for synthesis of pyrazoles (5a–5k). H2O (1 mL) was used as solvent for synthesis of dihydropyrazoles (5l–5t). Yields in parenthesis are with Pd(dba)2 (0.5 equiv.). |
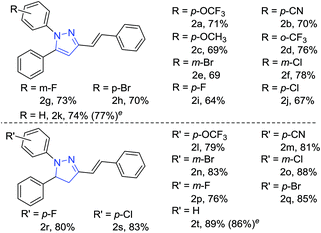 |
To obtain the dihydropyrazole product, reaction of Pd2(dba)3 was carried out with various substituted phenylhydrazines in H2O. The reaction of trifluoromethoxy, nitrile and halides (F, Cl, Br) substituted phenyl hydrazines afforded the corresponding dihydropyrazoles in good yields (Table 2, 2l–2s). Also, phenylhydrazine gave the desired product in high yield (Table 2, 2t). Following this, we thought to explore the scope of the current methodology for other metal complexes that could be used as coupling partners. Initially, the reaction of phenyl hydrazine with Cu(acac)2 in DMSO was performed to optimize the conditions under microwave irradiation (Scheme 2).
 |
| Scheme 2 Formation of pyrazole and/or other products (azobenzene and biphenyl) using phenylhydrazine and M(acac)2 in DMSO. | |
To extend the scope of various substituted pyrazoles, we screened different solvents with the Cu(acac)2 on model substrates (Table 3, entries 1–8) and among them, H2O (96%), DMSO (89%) and THF (90%) provided the desired 3,5-dimethyl-1-phenyl-1H-pyrazole (C) in high yields. In case of DMF and toluene, azobenzene (D) was observed as the major (>90%) product (Table 3, entries 3 and 8). Undoubtedly, an ideal solvent H2O was selected for further study. Furthermore, the reaction with different metal (Cu, Co, Pd, Ni, Cr, Mn)-diketones were also explored; the results showed the formation of the desired pyrazole in good to excellent yields (63–96%). To our delight, Cu(acac)2 showed the best results (Table 3, entries 1 and 9–13). The required minimum amount of Cu(acac)2 for the reaction was also optimized (Table 3, entries 1 and 14–16). On decreasing the amount to 0.5 equiv., yield of the product was not affected (Table 3, entry 17). However, on further decreasing the amount drastically, the yield of the product greatly reduced (Table 3, entries 14 and 15). The increase in microwave (MW) power did not influence the yields per time of the present reaction (Table 3, entries 17 and 18).
Table 3 Optimization of conditions for 3,5-dimethyl substituted pyrazolea

|
Entry |
M(acac)2 (equiv.) |
Solvent |
MW (watts) |
Yieldb [%] |
Reaction conditions: phenyl hydrazine (1.0 mmol), M(acac)2 (equiv.), solvent (1 mL), MW (watts), 100 °C, for 5 min. Isolated yields. Azobenzene (40%) and biphenyl (60%) were formed as major products. Azobenzene was formed as major product (96%). |
1 |
Cu(acac)2 (1) |
H2O |
50 |
96 |
2 |
Cu(acac)2 (1) |
DMSO |
50 |
86 |
3c |
Cu(acac)2 (1) |
DMF |
50 |
0 |
4 |
Cu(acac)2 (1) |
MeOH |
50 |
35 |
5 |
Cu(acac)2 (1) |
PEG-200 |
50 |
63 |
6 |
Cu(acac)2 (1) |
CHCl3 |
50 |
52 |
7 |
Cu(acac)2 (1) |
THF |
50 |
90 |
8d |
Cu(acac)2 (1) |
Toluene |
50 |
6 |
9 |
Co(acac)2 (1) |
H2O |
50 |
89 |
10 |
Pd(acac)2 (1) |
H2O |
50 |
88 |
11 |
Ni(acac)2 (1) |
H2O |
50 |
72 |
12 |
Cr(acac)2 (1) |
H2O |
50 |
65 |
13 |
Mn(acac)2 (1) |
H2O |
50 |
63 |
14 |
Cu(acac)2 (0.05) |
H2O |
50 |
9 |
15 |
Cu(acac)2 (0.25) |
H2O |
50 |
45 |
16 |
Cu(acac)2 (0.5) |
H2O |
50 |
96 |
17 |
Cu(acac)2 (0.05) |
H2O |
100 |
95 |
18 |
Cu(acac)2 (1) |
H2O |
100 |
96 |
The present reaction was explored for the synthesis of various 1,3,5-trisubstituted pyrazoles. Different types of functional groups were tolerated under the present reaction conditions. The reaction of phenyl hydrazine gave the desired pyrazole in 96% yield (Table 4, 3a). Halogen bearing phenyl hydrazines proceeded well and afforded the corresponding 1,3,5-trisubstituted pyrazoles in high yields (Table 4, 3b–3j). Nitrile, nitro, methoxy, methyl and methylenedioxy accommodated phenyl hydrazines also gave the corresponding pyrazoles in excellent yields (Table 4, 3k–3p). The labile ester group remained intact in the final product during the reaction (Table 4, 3q). The reaction of hydrazine having the heterocyclic ring system, i.e., 2-hydrazinopyridine, afforded the corresponding pyrazole product in high yield (Table 4, 3r).
Table 4 Synthesis of 1,3,5-trisubstituted pyrazolesab

|
Reaction conditions: hydrazine (1.0 mmol), Cu(acac)2 (0.5 equiv.), H2O (1 mL), MW (50 watts), 100 °C, for 5 min. Isolated yields. |
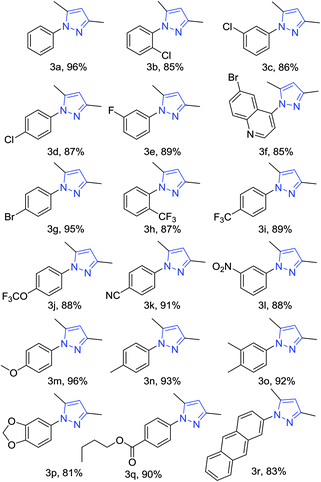 |
Next, with the thought of further expanding the scope of the reaction, we moved our attention towards applying the methodology on sulfonyl hydrazines. The reaction with various substituted aryl sulfonylhydrazines such as methyl, methoxyl, halides, trifluoromethyl, trifluoromethoxyl, nitrile, and naphthalene substituted sulfonyl hydrazines gave the corresponding arylsulfonyl pyrazoles in excellent yields (Table 5, 5a–5m).
Table 5 Synthesis of 3,5-dimethyl-N-sulfonyl aryl-1H-pyrazolesab

|
Reaction conditions: sulfonylhydrazine (1.0 mmol), Cu(acac)2 (0.5 equiv.), H2O (1 mL), MW (50 watts), 100 °C, for 5 min. Isolated yields. |
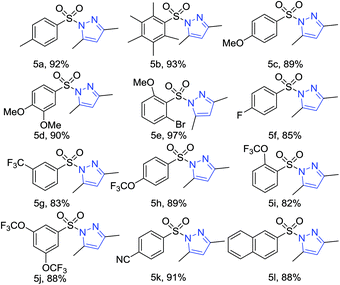 |
As an extension of the present method, a typical example of alkyl-hydrazine, i.e., phenethylhydrazine hydrochloride, was reacted under the optimized conditions, in which the desired product 3,5-dimethyl-1-phenethyl-1H-pyrazole (see ESI†) was obtained in trace amount.
The present protocol was applied in some complex molecules to elaborate the commercial importance in late stage modifications. In continuation to our ongoing project for the synthesis of selective PDE5 inhibitors,17 the pyrazole-bearing analogue with different heterocyclic moiety in the native structure of sildenafil (Fig. 1) was prepared only to show the utility of method in late stage modifications of important drug scaffolds.
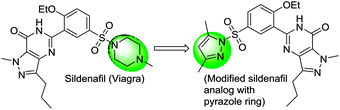 |
| Fig. 1 Direct application of method in preparation of pyrazole analogue of sildenafil. | |
3. Conclusions
In conclusion, we have developed a new method for direct N-heterocyclization of hydrazines with metal-diketones for the synthesis of various trisubstituted pyrazoles under microwave irradiation offering excellent yields. The synthesis of 1-aryl-5-phenyl-3-styryl-1H-pyrazoles was achieved in a single step, which is the most important finding in the present method. In addition to the various substituted phenyl hydrazines, this method was also applicable to various sulfonyl hydrazines, which are very important functional moieties in drug discovery. To ascribe the late stage modification importance of the present methodology, pyrazole analogue of sildenafil was also prepared. Bringing N-heterocyclic moieties, pyrazoles, in the final structure in a single step, the present method could have great importance in medicinal chemistry or drug discovery.
Conflicts of interest
There are no conflicts to declare.
Acknowledgements
The funding support from CSIR-India funded project HCP0008 and BSC0108 is gratefully acknowledged. SG thanks UGC for award of Senior Research Fellowship, PKV is thankful to DST-SERB for the Fast-track Young Scientist Grant. SDS thanks CSIR for the award of Raman Research Fellowship. IIIM communication No. IIIM/2148/2018.
Notes and references
-
(a) C. E. Mowbray, C. Burt, R. Corbau, S. Gayton, M. Hawes, M. Perros, I. Tran, D. A. Price, F. J. Quinton, M. D. Selby, P. A. Stupple, R. Webster and A. Wood, Bioorg. Med. Chem. Lett., 2009, 19, 5857 CrossRef PubMed;
(b) P. G. Wyatt, A. J. Woodhead, V. Berdini, J. Boulstridge, M. G. Carr, D. M. Cross, D. J. Davis, L. A. Devine, T. R. Early, R. E. Feltell, E. J. Lewis, R. L. MsMenamin, E. F. Navarro, M. A. O'Brien, M. O'Reilly, M. Reule, G. Saxty, L. A. Seavers, D. M. Smith, M. Squires, G. Trewartha, M. T. Walker and A. J. A. Woolford, J. Med. Chem., 2008, 51, 4986 CrossRef PubMed;
(c) P. Wickens, H. Kluender, J. Dixon, C. Brennan, F. Achebe, A. Bacchiocchi, D. Bankston, D. Bierer, M. Brands, D. Braun, M. S. Brown, C. Y. Chuang, J. Dumas, I. Enyedy, G. Hofilena, Z. Hong, T. Housley, B. Jones, U. Khire, C. Kreiman, E. Kumarasinghe, T. Lowinger, R. Ott-Morgan, L. Perkins, B. Phillips, R. Schoenleber, W. J. Scott, R. Sheeler, A. Redman, X. Sun, I. Taylor, L. Wang, S. Wilhelm, X. Zhang, M. Zhang, E. Sullivan, C. Carter, M. Miglarese and J. Levy, Bioorg. Med. Chem. Lett., 2007, 17, 4378 CrossRef PubMed;
(d) T. de Paulis, K. Hemstapat, Y. Chen, Y. Zhang, S. Saleh, D. Alagille, R. M. Baldwin, G. D. Tamagnan and P. J. Conn, J. Med. Chem., 2006, 49, 3332 CrossRef PubMed;
(e) A. A. Bekhit and T. Abdel-Aziem, Bioorg. Med. Chem., 2004, 12, 1935 CrossRef PubMed;
(f) A. Tanitame, Y. Oyamada, K. Ofuji, M. Fujimoto, K. Suzuki, T. Ueda, H. Terauchi, M. Kawasaki, K. Nagai, M. Wachi and J. I. Yamagishi, Bioorg. Med. Chem., 2004, 12, 5515 CrossRef PubMed;
(g) K. M. Kasiotis, E. N. Tzanetou and S. A. Haroutounian, Front. Chem., 2014, 2, 78 Search PubMed;
(h) A. Balbi, M. Anzaldi, C. Maccio, C. Aiello, M. Mazzei, R. Gangemi, P. Castagnola, M. Miele, C. Rosano and M. Viale, Eur. J. Med. Chem., 2011, 46, 5293 CrossRef PubMed;
(i) M. F. Khan, M. M. Alam, G. Verma, W. Akhtar, M. Akhter and M. Shaquiquzzaman, Eur. J. Med. Chem., 2016, 120, 170 CrossRef PubMed.
-
(a) F. Giornal, S. Pazenok, L. Rodefeld, N. Lui, J. P. Vors and F. R. Leroux, J. Fluorine Chem., 2013, 152, 2 CrossRef;
(b) J. Jaunzems and M. Braun, Org. Process Res. Dev., 2014, 18, 1055 CrossRef;
(c) P. Lei, X. Zhang, Y. Xu, G. Xu, X. Liu, X. Yang, X. Zhang and Y. Ling, Chem. Cent. J., 2016, 10, 40 CrossRef PubMed.
-
(a) M. A. Halcrow, Dalton Trans., 2009, 2059 RSC;
(b) J. Perez and L. Riera, Eur. J. Inorg. Chem., 2009, 2009, 4913 CrossRef;
(c) I. R. Fernando, S. Jianrattanasawat, N. Daskalakis, K. D. Demadis and G. Mezei, CrystEngComm, 2012, 14, 908 RSC;
(d) M. Ishida, H. Fujimoto, T. Morimoto, S. Mori, M. Toganoh, S. Shimizu and H. Furuta, Supramol. Chem., 2017, 29, 8 CrossRef.
-
(a) V. Mukundam, A. Kumar, K. Dhanunjayarao, A. Ravi, S. Peruncheralathan and K. Venkatasubbaiah, Polym. Chem., 2015, 6, 7764 RSC;
(b) S. Carlotto, M. Casarin, A. Lanza, F. Nestola, L. Pandolfo, C. Pettinari and R. Scatena, Cryst. Growth Des., 2015, 15, 5910 CrossRef.
-
(a) M. Viciano-Chumillas, S. Tanase, L. Jos de Jongh and J. Reedijk, Eur. J. Inorg. Chem., 2010, 2010, 3403 CrossRef;
(b) J. Olguin and S. Brooker, Coord. Chem. Rev., 2011, 255, 203 CrossRef.
-
(a) B. H. Pearce, H. F. Ogutu and R. C. Luckay, Eur. J. Inorg. Chem., 2017, 1189 CrossRef;
(b) F. Estevan, P. Hirva, A. Ofori, M. Sanau, T. Spec and M. Ubeda, Inorg. Chem., 2016, 55, 2101 CrossRef PubMed;
(c) W. D. Bailey, L. Luconi, A. Rossin, D. Yakhvarov, S. E. Flowers, W. Kaminsky, R. A. Kemp, G. Giambastiani and K. I. Goldberg, Organometallics, 2015, 34, 3998 CrossRef.
- V. Kumar, K. Kaur, G. K. Gupta and A. K. Sharma, Eur. J. Med. Chem., 2013, 69, 735 CrossRef PubMed.
- A. Ansari, A. Ali, M. Asif and Shamsuzzaman, New J. Chem., 2017, 41, 16 RSC.
-
(a) M. Suri, T. Jousseaume, J. J. Neumann and F. Glorius, Green Chem., 2012, 14, 2193 RSC;
(b) P. Prasanna, S. Perumal and J. C. Menéndez, Green Chem., 2013, 15, 1292 RSC;
(c) Q. Wang, Z. Zhang, Z. Du, H. Hua and S. Chen, Green Chem., 2013, 15, 1048 RSC;
(d) F. Li, J. Nie, L. Sun, Y. Zheng and J. A. Ma, Angew. Chem., Int. Ed., 2013, 52, 6255 CrossRef PubMed;
(e) R. Harigae, K. Moriyama and H. Togo, J. Org. Chem., 2014, 79, 2049 CrossRef PubMed;
(f) S. Dadiboyena and A. Nefzi, Eur. J. Med. Chem., 2011, 46, 5258 CrossRef PubMed;
(g) S. Fustero, M. Sánchez-Roselló, P. Barrio and A. Simón-Fuentes, Chem. Rev., 2011, 111, 6984 CrossRef PubMed;
(h) J. L. Tang, L. Ming and X. M. Zhao, J. Heterocyclic Chem., 2016, 53, 1367–1370 CrossRef.
-
(a) L. Knorr, Ber. Dtsch. Chem. Ges, 1883, 16, 2587 CrossRef;
(b) V. L. M. Silva, A. M. S. Silva, D. C. G. A. Pinto, J. A. S. Cavaleiro and J. Elguero, Eur. J. Org. Chem., 2004, 117, 4348 CrossRef;
(c) S. T. Heller and S. R. Natarajan, Org. Lett., 2006, 8, 2675 CrossRef PubMed;
(d) E. Vickerstaffe, B. H. Warrington, M. Ladlow and S. V. J. Ley, Comb. Chem., 2004, 6, 332 CrossRef PubMed;
(e) S. K. Singh, M. Srinivasa Reddy, S. Shivaramakrishna, D. Kavitha, R. Vasudev, J. M. Babu, A. Sivalakshmidevi and Y. Rao, Tetrahedron Lett., 2004, 45, 7679 CrossRef;
(f) V. Polshettiwar and R. S. Varma, Tetrahedron, 2010, 66, 1091 CrossRef;
(g) A. DeAngelis, D. H. Wang and S. L. Buchwald, Angew. Chem., Int. Ed., 2013, 52, 3434 CrossRef PubMed;
(h) W. Jin, H. F. Yu and Z. K. Yu, Tetrahedron Lett., 2011, 52, 5884 CrossRef.
-
(a) M. Zolfigol, D. Azarifar and B. Maleki, Tetrahedron Lett., 2004, 45, 2181 CrossRef;
(b) L. Chai, Y. Zhao, Q. Sheng and Z. Q. Liu, Tetrahedron Lett., 2006, 47, 9283 CrossRef;
(c) G. Su, W. T. Wu, J. T. Wang and L. M. Wu, Chin. Chem. Lett., 2008, 19, 1013 CrossRef;
(d) N. Nakamichi, Y. Kawashita and M. Hayashi, Org. Lett., 2002, 4, 3955 CrossRef PubMed;
(e) Y. Kawashita and M. Hayashi, Molecules, 2009, 14, 3073 CrossRef PubMed;
(f) G. S. Ananthnag, A. Adhikari and M. S. Balakrishna, Catal. Commun., 2014, 43, 240 CrossRef.
-
(a) T. H. Zhang and W. L. Bao, J. Org. Chem., 2013, 78, 1317 CrossRef PubMed;
(b) J. T. Hu, S. Chen, Y. H. Sun, J. Yang and Y. Rao, Org. Lett., 2012, 14, 5030 CrossRef PubMed;
(c) X. W. Li, L. He, H. J. Chen, W. Q. Wu and H. F. Jiang, J. Org. Chem., 2013, 78, 3636 CrossRef PubMed;
(d) G. W. Zhang, Y. Zhao and H. B. Ge, Angew. Chem., Int. Ed., 2013, 52, 2559 CrossRef PubMed;
(e) M. Zora and A. Kivrak, J. Org. Chem., 2011, 76, 9379 CrossRef PubMed;
(f) P. Liu, Y. M. Pan, Y. L. Xu and H. S. Wang, Org. Biomol. Chem., 2012, 10, 4696 RSC;
(g) Z. L. Li, J. H. Liu, Z. W. Huang, Y. Yang, C. G. Xia and F. W. Li, ACS Catal., 2013, 3, 839 CrossRef;
(h) P. Rai, M. Srivastava, J. Singh and J. Singh, RSC Adv., 2014, 4, 779 RSC.
-
(a) J. Hu, S. Chen, Y. Sun, J. Yang and R. Yu, Org. Lett., 2012, 14, 5030 CrossRef PubMed;
(b) T. Zhang and W. Bao, J. Org. Chem., 2013, 78, 1317 CrossRef PubMed;
(c) X. Li, H. Li, H. Chen, W. Wu and H. Jiang, J. Org. Chem., 2013, 78, 3636 CrossRef PubMed.
- X. W. Fan, T. Lei, C. Zhou, Q. Y. Meng, B. Chen, C. H. Tung and L. Z. Wu, J. Org. Chem., 2016, 81, 7127 CrossRef PubMed.
- S. Naik and V. Desai, Green Sustainable Chem., 2013, 3, 1 CrossRef.
-
(a) K. N. V. C. Lakshmi, M. Venkatesh, J. Hareesh, B. S. Raju and B. Anupama, Pharma Chem., 2016, 8, 122 Search PubMed;
(b) Z. Daneshfar and A. Rostami, RSC Adv., 2015, 5, 104695 RSC;
(c) V. N. Pathak, R. Joshi, J. Sharma, N. Gupta and V. M. Rao, Phosphorus, Sulfur Silicon, 2009, 184, 1854 CrossRef.
-
(a) S. D. Sawant, G. L. Reddy, M. I. Dar, M. Srinivas, G. Gupta, P. K. Sahu, P. Mahajan, A. Nargotra, S. Singh, S. C. Sharma, M. Tikoo, G. Singh, R. A. Vishwakarma and S. H. Syed, Bioorg. Med. Chem., 2015, 23, 2121 CrossRef PubMed;
(b) S. D. Sawant, G. L. Reddy, M. Srinivas, S. S. Hussain, M. I. Dar, A. Nargotra, P. Mahajan and R. A. Vishwakarma, WO 2015/114647 A1, 2015.
Footnotes |
† Electronic supplementary information (ESI) available. See DOI: 10.1039/c8ra04550j |
‡ Equal contribution as first author. |
|
This journal is © The Royal Society of Chemistry 2018 |
Click here to see how this site uses Cookies. View our privacy policy here.