DOI:
10.1039/C8RA04325F
(Paper)
RSC Adv., 2018,
8, 34000-34003
Organocatalytic sulfa-Michael/aldol cascade: constructing functionalized 2,5-dihydrothiophenes bearing a quaternary carbon stereocenter†
Received
21st May 2018
, Accepted 24th September 2018
First published on 3rd October 2018
Abstract
A practical sulfa-Michael/aldol cascade reaction of 1,4-dithiane-2,5-diol and α-aryl-β-nitroacrylates has been developed, which allows efficient access to functionalized 2,5-dihydrothiophenes bearing a quaternary carbon stereocenter in moderate to good yields with high enantioselectivities.
Among the various classes of heterocycles, members of the thiophene family have received particular attention from the chemical community because of their widespread occurrence as ubiquitous motifs in natural products, pharmaceuticals, agrochemicals as well as materials.1 In this context, the 2,5-dihydrothiophene ring is a common structural feature of many bioactive compounds and a potential intermediate for various synthetic applications.2 Over the decades, only a few examples of the assembly of optically active 2,5-dihydrothiophenes have been documented.3 For instance, the Spino3b group successfully prepared non-racemic dihydrothiophenes using an efficient chiral auxiliary. The first gold-catalyzed cycloisomerization of α-hydroxyallenes to 2,5-dihydrothiophenes was reported by Krause3c and co-workers. Then in 2010, the Xu3e group developed a highly stereoselective domino thia-Michael/aldol reaction between 1,4-dithiane-2,5-diol and α,β-unsaturated aldehyde catalyzed by a chiral diphenylprolino TMS ether, which provided a new avenue for the synthesis of functionalized 2,5-dihydrothiophenes.
Quaternary carbon stereocenters are often contained in natural products and pharmaceuticals.4 Compared with the chiral pool synthesis,5 the procedure of chiral materials or catalysts to construct such sterically congested stereogenic centers is more challenging because of the difficulty of orbital overlap.6 To date, a lot of progresses have been made in the construction of chiral quaternary carbon centers in cyclic compounds,7 which are greatly accelerated by the advancement of transition metal catalysis,8 and organocatalysis,9 including methods beyond radical initiation.10 However, only few examples are about the construction of a quaternary carbon in 2,5-dihydrothiophene ring.11 Inspired by the previous work of the Xu group,3e we describe herein an elegant organocatalytic asymmetric cascade sulfa-Michael/aldol reaction, providing a convenient way for the synthesis of 2,5-dihydrothiophenes bearing a chiral quaternary cabon center.
Results and discussion
At the outset of our pursuit, a series of organocatalysts I–VII were examined in the cascade reaction of 1,4-dithiane-2,5-diol 1a and α-phenyl-β-nitroacrylate 2a in chloroform at room temperature (Fig. 1). The desired 2,5-dihyrothiophene 3a could be successfully obtained in the presence of cinchona alkaloid I within 24 hours in 40% yield with only 12% ee (Table 1, entry 1). A substantial increase of yield and enantioselectivity were observed when thiourea-tertiary amine catalysts II–V were employed (Table 1, entries 2–5). Further optimization indicated that cinchona-based squaramide VI and VII with stronger H-bonding donors gave the products with comparable results (Table 1, entries 6 and 7), then we selected VII as the optimal catalyst. Next, changing chloroform to other solvents resulted in an obvious decrease both in yield and stereoselectivity (Table 1, entries 8–13). To our delight, the addition of MgSO4 or molecular sieve (MS) into the reaction mixture could bring out a slight increase of the enantioselectivity, while the 5 Å MS gave the best results (Table 1, entry 16). We also tried to set up the reaction under a lower temperature, the cycloadduct 3a could maintain the yield and enantioselectivity within a longer time at 15 °C (Table 1, entry 17). When the temperature was further decreased to 0 °C, the reaction became very slow, and we only observed trace amount of product after 120 hours (Table 1, entry 18).
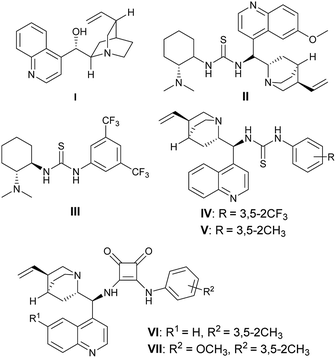 |
| Fig. 1 The organocatalysts tested in this study. | |
Table 1 Optimization of the reaction conditionsa
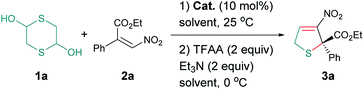
|
Entry |
Catalyst |
Solvent |
Time (h) |
Yieldb (%) |
eec (%) |
Reaction conditions (unless otherwise stated): 1a (0.18 mmol), 2a (0.3 mmol), catalyst (10 mol%), solvent (2 mL), T1 = 25 °C; then trifluoroacetic anhydride (TFAA, 0.6 mmol), Et3N (0.6 mmol), T2 = 0 °C. Isolated yield based on 2a. Determined by chiral HPLC analysis. MgSO4 (90 mg) was added. 4 Å MS (90 mg) was added. 5 Å MS (90 mg) was added. T1 = 15 °C. T1 = 0 °C, ND = not determined. |
1 |
I |
CHCl3 |
24 |
40 |
12 |
2 |
II |
CHCl3 |
24 |
55 |
33 |
3 |
III |
CHCl3 |
24 |
62 |
−56 |
4 |
IV |
CHCl3 |
24 |
65 |
45 |
5 |
V |
CHCl3 |
24 |
50 |
66 |
6 |
VI |
CHCl3 |
24 |
66 |
70 |
7 |
VII |
CHCl3 |
24 |
69 |
71 |
8 |
VII |
CH2Cl2 |
24 |
64 |
60 |
9 |
VII |
Toluene |
24 |
56 |
62 |
10 |
VII |
THF |
24 |
35 |
48 |
11 |
VII |
Et2O |
24 |
68 |
66 |
12 |
VII |
CH3OH |
24 |
44 |
3 |
13 |
VII |
CH3CN |
24 |
43 |
12 |
14d |
VII |
CHCl3 |
24 |
63 |
73 |
15e |
VII |
CHCl3 |
24 |
68 |
76 |
16f |
VII |
CHCl3 |
24 |
70 |
77 |
17f,g |
VII |
CHCl3 |
55 |
68 |
73 |
18f,h |
VII |
CHCl3 |
120 |
Trace |
ND |
With the optimal conditions in hand, the cascade reaction of 1,4-dithiane-2,5-diol and diverse α-aryl-β-nitroacrylates was investigated. The results were summarized in Table 2. We found that α-phenyl-β-nitroacrylates with different ester groups, including benzyl, isopropyl, tert-butyl, could react smoothly to give the desired chiral dihydrothiophenes in 67–73% yields with 66–83% ee (entries 1–3), while bulky tert-butyl group gave the best results (entry 3). The position and nature of substituents on the α-phenyl ring had slight influence on the stereocontrol, 65–75% yields and 82–86% ee were observed (entries 4–9). The reaction was also applicable to α-naphthyl and furanyl nitroacrylates, producing the desired adducts with 83% and 85% ee, respectively, the yields were also satisfactory (entries 10 and 11). Gratifyingly, branched as well as aliphatic nitroacrylate is equally suitable substrate (entry 12). In addition, we tried the reaction of 2,5-dihydroxy-1,4-dithiane-2,5-dicarboxylate with α-aryl-β-nitroacrylates 2n and 2o, which exhibited promising results, leading to 64–65% yields and 87–88% ee at −40 °C within 36 hours (entries 13 and 14).
Table 2 Scope for the synthesis of 2,5-dihydrothiophenes bearing a quaternary carbon stereocentera
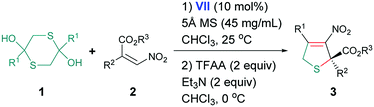
|
Entry |
Product |
R1 |
R2 |
R3 |
t (h) |
Yieldb (%) |
eec (%) |
Reaction conditions (unless otherwise stated): 1 (0.18 mmol), 2 (0.3 mmol), VII (10 mol%), 5 Å MS (90 mg), CHCl3 (2 mL), T1 = 25 °C; then TFAA (0.6 mmol), Et3N (0.6 mmol), T2 = 0 °C. Isolated yield based on 2. Determined by chiral HPLC analysis. T1 = −40 °C. |
1 |
3b |
H |
Ph |
Bn |
24 |
68 |
66 |
2 |
3c |
H |
Ph |
i-Pr |
24 |
67 |
80 |
3 |
3d |
H |
Ph |
t-Bu |
24 |
73 |
83 |
4 |
3e |
H |
4-CH3C6H4 |
t-Bu |
36 |
65 |
86 |
5 |
3f |
H |
4-OCH3C6H4 |
t-Bu |
24 |
71 |
82 |
6 |
3g |
H |
4-FC6H4 |
t-Bu |
24 |
68 |
85 |
7 |
3h |
H |
4-ClC6H4 |
t-Bu |
24 |
75 |
86 |
8 |
3i |
H |
3-OCH3C6H4 |
t-Bu |
24 |
74 |
84 |
9 |
3j |
H |
3,5-2CH3C6H3 |
t-Bu |
30 |
67 |
83 |
10 |
3k |
H |
2-Naphthyl |
t-Bu |
24 |
72 |
86 |
11 |
3l |
H |
2-Furanyl |
t-Bu |
24 |
62 |
83 |
12 |
3m |
H |
i-Pr |
t-Bu |
24 |
69 |
85 |
13d |
3n |
CO2Et |
Ph |
t-Bu |
36 |
65 |
87 |
14d |
3o |
CO2Et |
3,5-2CH3C6H3 |
t-Bu |
36 |
64 |
88 |
To determine the absolute configuration of the products, we carried the cascade reaction in a large amount, and a single crystal of the optically pure product 3a was obtained after crystallization,12 which was then determined to be S by X-ray crystallographic analysis.13 Based on the experimental result and previous studies,14 the transition state is shown in Scheme 1. Mechanistically, the β-nitroacrylate was activated by the hydrogen bonds of the squaramide, while the tertiary amine of the catalyst would enhance the nucleophilicity of mercaptoacetaldehyde based on its basicity, the nucleophile attacked the Michael acceptor from the Si face, which favoured the formation of the C2 (S) stereogenic center. It is worth noting that the prediction of possible interaction in the transition state was in agreement with the DFT calculation (Fig. 2).15
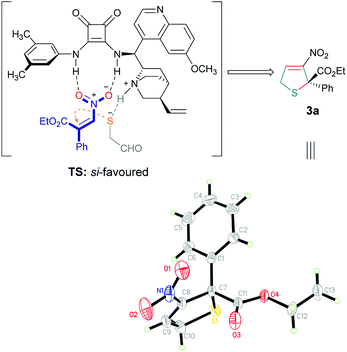 |
| Scheme 1 Proposed transition state and the X-ray structure of 3a. | |
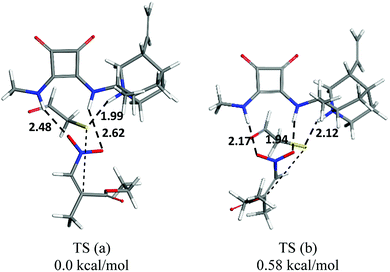 |
| Fig. 2 Optimized structures and free energy gap of the transition states. | |
Because of the highly functionalization of the product, we also tried the synthetic transformation of 2,5-dihydrothiophene. Taking the reaction of 1a and 2d as example, when the addition and dehydration steps completed at the optimal conditions, the benzotriazole was added directly to the mixture as a nucleophile. Interestingly, the adduct 4 was obtained with moderate yield and high stereoselectivities (eqn (1), 58% yield, >19
:
1 dr, 85% ee).16
|
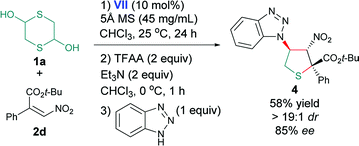 | (1) |
Conclusions
In summary, we have developed a practical sulfa-Michael/aldol cascade reaction of 1,4-dithiane-2,5-diol and α-aryl-β-nitroacrylates, which allows an efficient access to functionalized 2,5-dihydrothiophenes bearing a quaternary carbon center in moderate to good yields with high enantioselectivities. Moreover, the products can be easily derivated to more complex compound. Further application of this strategy to the enantioselective synthesis of other heterocyclic systems is underway in our laboratory.
Conflicts of interest
There are no conflicts to declare.
Acknowledgements
We gratefully acknowledge the National Science Foundation of China (21502118) for financial support.
Notes and references
-
(a) A. Acharya, G. Parameshwarappa, B. Saraiah and H. Ila, J. Org. Chem., 2015, 80, 414 CrossRef CAS PubMed;
(b) A. Acharya, V. Gautam and H. Ila, J. Org. Chem., 2017, 82, 7920 CrossRef CAS PubMed.
-
(a) E. Rogers, H. Araki, L. A. Batory, C. E. Mclnnis and J. T. Njardarson, J. Am. Chem. Soc., 2007, 129, 2768 CrossRef CAS PubMed;
(b) P. G. McGarraugh and S. E. Brenner, Org. Lett., 2009, 11, 5654 CrossRef CAS PubMed;
(c) S. Benetti, C. De Risi, G. P. Pollini and V. Zanirato, Chem. Rev., 2012, 112, 2129 CrossRef CAS PubMed;
(d) C. De Risi, S. Benetti, M. Fogagnolo and V. Bertolasi, Tetrahedron Lett., 2013, 54, 283 CrossRef CAS;
(e) A. Zaghi, T. Bernardi, V. Bertolasi, O. Bortolini, A. Massi and C. De Risi, J. Org. Chem., 2015, 80, 9176 CrossRef CAS PubMed.
-
(a) G. Spagnol, M.-P. Heck, S. P. Nolan and C. Mioskowski, Org. Lett., 2002, 4, 1767 CrossRef CAS PubMed;
(b) J. Minville, M. Girardin and C. Spino, Can. J. Chem., 2007, 85, 603 CrossRef CAS;
(c) N. Morita and N. Krause, Angew. Chem., Int. Ed., 2006, 45, 1897 CrossRef CAS PubMed;
(d) N. Krause, V. Belting, C. Deutsch, J. Erdsack, H.-T. Fan, H. B. Gockel, A. Hoffmann-Röder, N. Morita and F. Volz, Pure Appl. Chem., 2008, 80, 1063 CAS;
(e) J. Tang, D.-Q. Xu, A.-B. Xia, Y.-F. Wang, J.-R. Jiang, S.-P. Luo and X.-Y. Xu, Adv. Synth. Catal., 2010, 352, 2121 CrossRef CAS;
(f) Y. Xiang, J. Song, Y. Zhang, Y. D.-C. Yang, Z. Guan and Y.-H. He, J. Org. Chem., 2016, 81, 6042 CrossRef CAS PubMed.
- D. J. Newman and G. M. Cragg, J. Nat. Prod., 2016, 79, 629 CrossRef CAS PubMed.
-
(a) H. X. Ding, C. A. Leverett, R. E. Kyne, K.-C. K. Liu, S. J. Fink, A. C. Flick and C. J. O'Donnell, Bioorg. Med. Chem., 2015, 23, 1895 CrossRef CAS PubMed;
(b) T. P. Stockdale and C. M. Williams, Chem. Soc. Rev., 2015, 44, 7737 RSC.
- J.-J. Feng, M. Holmes and M. J. Krische, Chem. Rev., 2017, 117, 12564 CrossRef CAS PubMed.
- For selected reviews, see:
(a) L. M. Repka and S. E. Reisman, J. Org. Chem., 2013, 78, 12314 CrossRef CAS PubMed;
(b) K. W. Quasdorf and L. E. Overman, Nature, 2014, 516, 181 CrossRef CAS PubMed;
(c) T. Ling and F. Rivas, Tetrahedron, 2016, 72, 6729 CrossRef CAS;
(d) M. Büschleb, S. Dorich, S. Hanessian, D. Tao, K. B. Schenthal and L. E. Overman, Angew. Chem., Int. Ed., 2016, 55, 4156 CrossRef PubMed;
(e) H.-L. Li and Y.-X. Lu, Asian J. Org. Chem., 2017, 6, 1130 CrossRef CAS.
- For selected recent examples, see:
(a) J.-W. Park, Z.-W. Chen and V. M. Dong, J. Am. Chem. Soc., 2016, 138, 3310 CrossRef CAS PubMed;
(b) Z.-S. Chen, X.-Y. Huang, J.-M. Gao and K.-G. Ji, Org. Lett., 2016, 18, 5876 CrossRef CAS PubMed;
(c) K. Balaraman and C. Wolf, Angew. Chem., Int. Ed., 2017, 56, 1390 CrossRef CAS PubMed;
(d) J.-W. Liu, Z.-B. Han, X.-M. Wang, F. Meng, Z. Wang and K.-L. Ding, Angew. Chem., Int. Ed., 2017, 56, 5050 CrossRef CAS PubMed;
(e) S.-Q. Xu, C. Wang, M. Komiyama, Y. Tomonari and E. Negishi, Angew. Chem., Int. Ed., 2017, 56, 11502 CrossRef CAS PubMed;
(f) J.-F. Zheng, L.-L. Lin, L. Dai, Q. Tang, X.-H. Liu and X.-M. Feng, Angew. Chem., Int. Ed., 2017, 56, 13107 CrossRef CAS PubMed;
(g) X.-W. Wu, B. Wang, Y. Zhou and H. Liu, Org. Lett., 2017, 19, 1294 CrossRef CAS PubMed;
(h) Y. Ogiwara, K. Sato and N. Sakai, Org. Lett., 2017, 19, 5296 CrossRef CAS PubMed;
(i) M. A. Abozeid, S. Sairenji, S. Taizawa, M. Fujita and H. Sasai, Chem. Commun., 2017, 53, 6887 RSC;
(j) L.-L. Hou, Y.-J. Yuan and X.-F. Tong, Org. Biomol. Chem., 2017, 15, 4803 RSC;
(k) K. Li, F.-L. Zhu, Z.-T. Liu, J. Tong and X.-P. Hu, Org. Biomol. Chem., 2018, 16, 742 RSC.
- For selected recent examples, see:
(a) B. M. Trost, T. Saget and C.-I. Hung, J. Am. Chem. Soc., 2016, 138, 3659 CrossRef CAS PubMed;
(b) C.-C. Zou, C.-K. Zeng, Z. Liu, M. Lu, X.-H. Sun and J.-X. Ye, Angew. Chem., Int. Ed., 2016, 55, 14257 CrossRef CAS PubMed;
(c) S. Bera, C. G. Daniliuc and A. Studer, Angew. Chem., Int. Ed., 2017, 56, 7402 CrossRef CAS PubMed;
(d) J.-Y. Liu, J. Zhao, J.-L. Zhang and P.-F. Xu, Org. Lett., 2017, 19, 1846 CrossRef CAS PubMed;
(e) S.-M. Yang, G. M. Reddy, T.-P. Wang, Y.-S. Yeh, M. Wang and W.-W. Lin, Chem. Commun., 2017, 53, 7649 RSC;
(f) L. Wang, A. Rahman and X.-F. Lin, Org. Biomol. Chem., 2017, 15, 6033 RSC;
(g) M. Zhao, J. Chen, H. Yang and L. Zhou, Chem.–Eur. J., 2017, 23, 2783 CrossRef CAS PubMed;
(h) J. Luo, Q.-X. Cao, X.-H. Cao and X.-D. Zhao, Nat. Commun., 2018, 9, 527 CrossRef PubMed;
(i) Q. Li, L. Zhou, X.-D. Shen, K.-C. Yang, X. Zhang, Q.-S. Dai, H.-J. Leng, Q.-Z. Li and J.-L. Li, Angew. Chem., Int. Ed., 2018, 57, 1913 CrossRef CAS PubMed;
(j) G. Pandey, J. Khamrai and A. Mishra, Org. Lett., 2018, 20, 166 CrossRef CAS PubMed;
(k) A. Rahman, E. Xie and X.-F. Lin, Org. Biomol. Chem., 2018, 16, 1367 RSC.
- For recent examples, see:
(a) N. S. Dange, F. Robert and Y. Landais, Org. Lett., 2016, 18, 6156 CrossRef CAS PubMed;
(b) S. Li, P.-P. Zhang, Y.-H. Li, S.-M. Lu, J.-X. Gong and Z. Yang, Org. Lett., 2017, 19, 4416 Search PubMed;
(c) H.-M. Huang, P. Bonilla and D. J. Procter, Org. Biomol. Chem., 2017, 15, 4159 RSC.
- H. Kumamoto, T. Nakai, K. Haraguchi, K. T. Nakamura, H. Tanaka, M. Baba and Y.-C. Cheng, J. Med. Chem., 2006, 49, 7861 CrossRef CAS PubMed.
- See the ESI† for more details.
- CCDC 869512 (for 3a) contains the supplementary crystallographic data for this paper.
- H.-H. Lu, F.-G. Zhang, S.-W. Duan and W.-J. Xiao, Org. Lett., 2009, 11, 3946 CrossRef CAS PubMed.
- See the ESI† for details.
- See the ESI† for more details.
Footnote |
† Electronic supplementary information (ESI) available. CCDC 869512. For ESI and crystallographic data in CIF or other electronic format see DOI: 10.1039/c8ra04325f |
|
This journal is © The Royal Society of Chemistry 2018 |