DOI:
10.1039/C8RA03882A
(Paper)
RSC Adv., 2018,
8, 28637-28641
Transition-metal-free base catalyzed intramolecular cyclization of 2-ynylphenols for efficient and facile synthesis of 2-substituted benzo[b]furans†
Received
7th May 2018
, Accepted 6th August 2018
First published on 10th August 2018
Abstract
A transition-metal-free base catalyzed intramolecular cyclization of 2-ynylphenols was developed for the facile synthesis of 2-substituted benzo[b]furans. Various 2-aryl and 2-alkyl substituted benzo[b]furans can be obtained with good to excellent yields using readily available Cs2CO3 as the catalyst under mild reaction conditions. The broad substrates scope and the typical maintenance of vigorous efficiency on gram scale make this protocol a potentially practical method to synthesize 2-substituted benzo[b]furans derivatives.
Introduction
2-Substituted benzo[b]furans are a significant structural scaffold especially in the pharmaceutical industry, both for natural and synthesized products, and have exhibited a broad range of biological and pharmacological activities.1–3 Typical pharmaceutical application of 2-substituted benzo[b]furan moiety bearing compounds (Fig. 1) that have been developed include those of drugs or agents used for anti-hyperlipemia,4 antimicrobial,5 anti-inflammatory,6 antifungal2 and so on. Therefore, considerable efforts have been directed toward the development of efficient methods for the synthesis of 2-substituted benzo[b]furan derivatives. Among the various synthetic approaches, transition metal catalyzed intramolecular cyclization of 2-ynylphenols (Scheme 1a) represents one type of important strategy leading to 2-substituted benzo[b]furan skeletons. Various precious metals such as Pt,7 Pd,8 Au/Ag,9 Au,10 Rh11 and Ir12 as well as other non-precious metals such as Zn13 and Cu14 have been developed as catalysts in the presence of stoichiometric base in most cases.
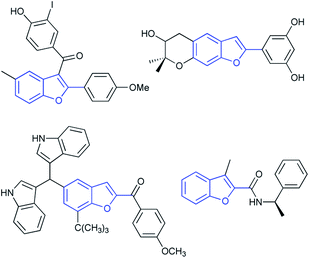 |
| Fig. 1 Pharmaceutically and biologically active molecules bearing 2-substituted benzo[b]furans skeletons. | |
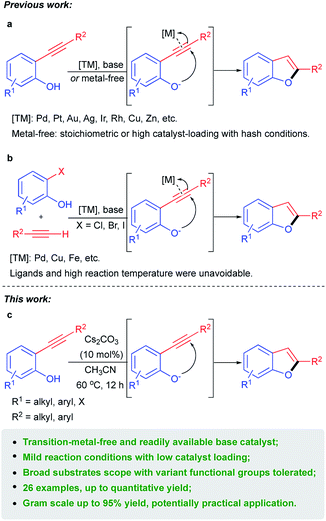 |
| Scheme 1 Previous synthetic methods for 2-substituted benzo[b]furans and the present metal-free catalytic system. | |
On the other hand, another representative pathway to 2-substituted benzo[b]furans was transition metal promoted15 or catalyzed16–18 one-pot synthesis from 2-halophenols and terminal alkynes through Sonogashira coupling followed by intramolecular cyclization of the corresponding 2-ynylphenol intermediates generated in situ (Scheme 1b). In this context, ligands and high reaction temperatures were usually unavoidable to satisfy the incipient Sonogashira coupling reaction which might bring some restrictions to their application. Competitively, one-pot synthesis of 2-substituted benzo[b]furans directly from phenols19 was also developed yet still could not get rid of transition metals catalysts. Taking the higher price of transition-metals into consideration, recently, transition-metal-free synthesis of 2-substituted benzo[b]furans was developed in the presence of stoichiometric20 or catalytic amount21 of base (Scheme 1a) and by photochemical22 or oxidative23 routes. Nevertheless, high catalyst-loading, harsh reaction conditions such as high reaction temperature and indispensable microwave, limited substrates compatibility and low chemselectivity for the desired 2-substituted benzo[b]furans products still bring some restrictions to their practical applications. Therefore, transition-metal-free catalytic systems with easily accessible and inexpensive catalysts and gentle reaction conditions for efficient and facile synthesis of 2-substituted benzo[b]furans are still highly desirable. Herein, we report a facile method for the synthesis of 2-substituted benzo[b]furans catalyzed by readily available base at the absence of transition metals (Scheme 1c). In the present work, a broad array of 2-aryl and 2-alkyl substituted benzo[b]furans can be obtained with excellent yields from the efficacious intramolecular cyclization of the corresponding 2-ynylphenols catalyzed by easily accessible Cs2CO3 at mild reaction conditions. And the semi-gram and gram scale transformations could also be realized with excellent isolated yields which represents this protocol a potentially practical method for the synthesis of 2-substituted benzo[b]furans derivatives.
Results and discussion
Our studies commenced with the intramolecular cyclization of 2-(phenylethynyl)phenol 1a as the model reaction and a variety of bases were evaluated in view of their easy accessibility and key role based on previous catalytic systems using acetonitrile as solvent at 60 °C. Firstly, a serious of readily available inorganic bases were examined for the titled reaction. As can be seen from Table 1, commonly used hydroxides of alkalis especially KOH exhibited good catalytic activity toward the formation of 2a with 89% isolated yield (Table 1, entries 1 and 2). To our delight, it was found that a quantitative yield of 2a could be obtained when cesium carbonate was used as the catalyst (Table 1, entry 5), although other carbonates of alkalis such as Na2CO3 and K2CO3 displayed poor even none efficiency toward the cyclization of 1a (Table 1, entries 3 and 4). The excellent catalytic activity of Cs2CO3 could be attributed to its stronger basicity and better solubility in acetonitrile compared with other inorganic bases,24 which endowed it with a powerful ability to activate 2-(phenylethynyl)phenol 1a. To the contrary, other commonly used strong bases such as t-BuOK, NaOMe and NaOEt exhibited poor even none activity for the transformation of 1a to 2a (Table 1, entries 6–8). Consequently, it was understandable that weaker base like NaOAc displayed no activity at all (Table 1, entry 9). In addition, several commonly used organic bases were also tested under the identical reaction conditions. As a result, only a low yield was given when the relatively strong base DBU was used as the catalyst (Table 1, entry 13) while other organic bases tested here were nearly completely negative for the formation of 2a (Table 1, entries 10–12), which could be ascribable to their weak basicity for the efficient activation of 1a. It was worth noting that there was no corresponding 2-phenylbenzo[b]furan 2a product detected at all in the absence of the base catalyst which could further prove the activation role of Cs2CO3 to 1a and thus its catalytic characteristic for this transformation (Table 1, entry 5 vs. entry 14). Therefore, Cs2CO3 was chosen as the optimal catalyst and then different solvents were further screened. It was found that the cyclization of 1a to 2a was quite inefficient both in nonpolar solvent such as toluene (Table 1, entry 15) and other medium polar aprotic solvents like THF, 1,4-dioxane, 1,2-dichloroethane, ethyl acetate and acetone (Table 1, entries 16–20), which probably ascribe to the poor solubility of Cs2CO3 in them. When it comes to the polar protic solvents such as H2O and ethanol, moderate yields could be obtained (Table 1, entries 21 and 22) with the increase of the solubility of both substrate and catalyst. Thus, acetonitrile could be proved to be the best solvent for the cyclization of 2-ynylphenol catalyzed by Cs2CO3. As comparison, it was found that acetonitrile was also the best solvent for KOH, which also exhibited good catalytic activity toward the cyclization of 2-ynylphenol, through control experiments (entries 2 vs. 23–26). At the same time, it was observed that either lower catalyst loading or lower reaction temperature was unfavourable for the transformation of 1a to 2a (entry 5 vs. entries 27 and 28). On the other hand, 12 h was necessary for the complete conversion of 1a to 2a (entry 5 vs. entries 29–31) with a satisfactory yield.
Table 1 Optimization of reaction conditions
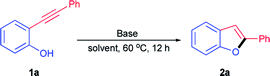
|
Entrya |
Base |
Solvent |
Yieldb (%) |
Reaction conditions: 2-(phenylethynyl)phenol 1a (0.3 mmol), base (10 mol%), solvent (3 mL), 60 °C, 12 h. Isolated yields. ND, not detected. DBU, 1,8-diazabicyclo[5.4.0]undec-7-ene. DCE, 1,2-dichloro ethane. 5 mol% Cs2CO3 was used. 40 °C. 6 h. 8 h. 10 h. |
1 |
NaOH |
CH3CN |
28 |
2 |
KOH |
CH3CN |
89 |
3 |
Na2CO3 |
CH3CN |
Trace |
4 |
K2CO3 |
CH3CN |
21 |
5 |
Cs2CO3 |
CH3CN |
>99 |
6 |
t-BuOK |
CH3CN |
21 |
7 |
NaOMe |
CH3CN |
7 |
8 |
NaOEt |
CH3CN |
NDc |
9 |
NaOAc |
CH3CN |
ND |
10 |
Et3N |
CH3CN |
Trace |
11 |
Pyridine |
CH3CN |
ND |
12 |
Piperidine |
CH3CN |
5 |
13d |
DBU |
CH3CN |
41 |
14 |
— |
CH3CN |
ND |
15 |
Cs2CO3 |
Toluene |
18 |
16 |
Cs2CO3 |
THF |
14 |
17 |
Cs2CO3 |
1,4-Dioxane |
31 |
18e |
Cs2CO3 |
DCE |
20 |
19 |
Cs2CO3 |
EtOAc |
31 |
20 |
Cs2CO3 |
Acetone |
10 |
21 |
Cs2CO3 |
H2O |
54 |
22 |
Cs2CO3 |
EtOH |
78 |
23 |
KOH |
THF |
ND |
24 |
KOH |
Acetone |
9 |
25 |
KOH |
H2O |
23 |
26 |
KOH |
EtOH |
80 |
27f |
Cs2CO3 |
CH3CN |
20 |
28g |
Cs2CO3 |
CH3CN |
9 |
29h |
Cs2CO3 |
CH3CN |
21 |
30i |
Cs2CO3 |
CH3CN |
89 |
31j |
Cs2CO3 |
CH3CN |
91 |
With the optimal reaction conditions in hand (Table 1, entry 5), we began to explore the substrates scope of the intramolecular cyclization of different 2-ynylphenols. Inspiringly, a wide array of 2-arylynyl and 2-alkylynyl substituted phenols were compatible to the present protocol and a variety of functional groups were tolerated (Table 2). Generally, almost all of the 2-arylynyl phenols bearing either electron-donating or electron-withdrawing substitutes on the ynyl-moiety could react smoothly to afford the corresponding 2-aryl-substituted benzo[b]furans products with good to quantitative yields (Table 2, 2a–2i, 2k–2l). However, with a –CF3 group on the ynyl-moiety, only 26% yield was achieved for the related benzo[b]furan product under the optimal conditions which might ascribe to the passivation caused by the –CF3 moiety (Table 2, 2j). Additionally, moderate to good yields could be obtained for 2-alkyl substituted benzo[b]furans and either ester group or hydroxyl could tolerate this basic reaction system (Table 2, 2m–2o). On the other hand, substrates bearing either electron-donating groups or electron-withdrawing groups such as halogens, methyl, phenyl and acetyl that located at different positions of the phenol-ring-moiety could also readily be transformed into the desired 2-substituted benzo[b]furans products with good to excellent yields (Table 2, 2p–2v). Besides that, it was worth noting that the present protocol was also highly active for 2-ynylphenols bearing substitutes both on the ynyl-moiety and on the phenol-ring-moiety. As a result, several polysubstituted benzo[b]furans derivatives could be approached with excellent to quantitative yields (Table 2, 2w–2z).
Table 2 Substrates scopea,b
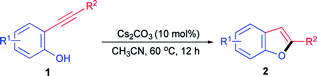
|
Reaction conditions: 2-ynylphenol 1, (0.3 mmol), Cs2CO3 (10 mol%), CH3CN (3 mL), 60 °C, 12 h. Isolated yields. |
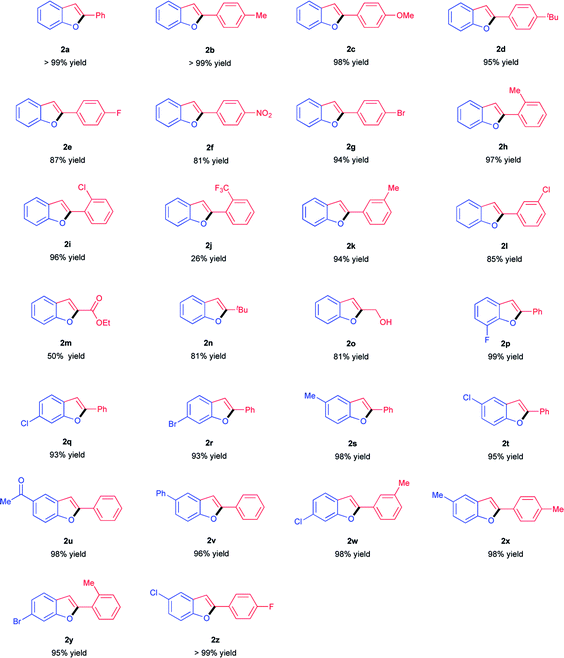 |
In order to evaluate the practical application of the present metal-free catalytic system, both the semi-gram and gram scale patterns were conducted using several 2-ynylphenols bearing different substitutes. As can be seen from Scheme 2, excellent yields could be obtained for both semi-gram and gram scale patterns under the optimal reaction conditions for the tested 2-ynylphenols, which indicated ideal maintenances of the vigorous efficiency of this catalytic system and enabled the present methodology potentially practical application for facile synthesis of 2-substituted benzo[b]furans derivatives.
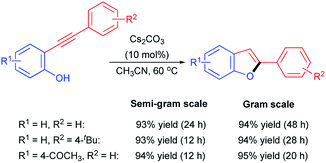 |
| Scheme 2 Semi-gram and gram scale reactions for Cs2CO3 catalyzed cyclization of 2-ynylphenols toward 2-substituted benzo[b]furans. | |
A plausible reaction mechanism is proposed as shown in Scheme 3. Firstly, 2-(phenylethynyl)phenol 1a can be activated through a deprotonation by Cs2CO3 with the formation of the nucleophilic phenolate intermediate I. Then an intramolecular nucleophilic cyclization of phenolate I to acetenyl, which might be activated by cesium ion through a metal–π activation, could afford the benzo[b]furan anion intermediate II. And the subsequent protonation of intermediated II by 2-(phenylethynyl)phenol could give the final product 2-phenylbenzo[b]furan 2a accompanied with the generation of phenolate intermediate I for further cyclization to afford benzo[b]furan product.
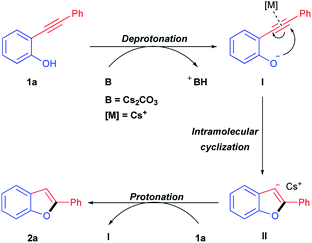 |
| Scheme 3 Plausible reaction mechanism. | |
Conclusions
In conclusion, we have developed an efficacious protocol for the synthesis of 2-substituted benzo[b]furans through a transition-metal-free Cs2CO3 catalyzed intramolecular cyclization of 2-ynylphenols under mild reaction conditions. Notably, the wide substrates scope and the typical maintenance of the excellent catalytic efficiency for gram scale enable the present method to be a potentially practical protocol for facile synthesis of 2-substituted benzo[b]furan derivatives.
Experimental
General procedure for Cs2CO3 catalyzed intramolecular annulation of 2-ynylphenols to 2-substituted benzo[b]furans
To a sealed tube with a magnetic bar were added 2-ynylphenols 1 (0.3 mmol) and acetonitrile (3 mL). After stirring at r.t. for 5 min, Cs2CO3 (10 mol%) was added and the resulting mixture was stirred at 60 °C for 12 hours. After cooling, the obtained mixture was filtered and the residue was washed twice with acetonitrile. Then the filtrate was evaporated under reduced pressure to remove the solvent and the residue was purified by flash column chromatography to obtain the corresponding 2-substituted benzo[b]furans 2.
Conflicts of interest
There are no conflicts to declare.
Acknowledgements
We are grateful to the National Natural Science Foundation of China (No. 21502231 and 21572273) and the Fundamental Research Funds for the Central Universities (No. 2015PY004) for financial supports. And we also thank Prof. Liang-Nian He from Nankai University for the help on EI-HRMS test.
Notes and references
- B. A. Keay, J. M. Hopkins and P. W. Dibble, in Comprehensive Heterocyclic Chemistry III, ed. A. R.Katrizky, Pergamon Press, New York, 1984, vol. 3, p. 571 Search PubMed.
- H. K. Shamsuzzaman, Eur. J. Med. Chem., 2015, 97, 483 CrossRef PubMed.
- R. Naik, D. S. Harmalkar, X.-Z. Xu, K. Jang and K. Lee, Eur. J. Med. Chem., 2015, 90, 379 CrossRef PubMed.
- K. V. Sashidhara, R. K. Modukuri, R. Sonkar, K. B. Rao and G. Bhatia, Eur. J. Med. Chem., 2013, 68, 38 CrossRef PubMed.
- X.-Z. Jiang, W.-L. Liu, W. Zhang, F.-Q. Jiang, Z. Gao, H. Zhuang and L. Fu, Eur. J. Med. Chem., 2011, 46, 3526 CrossRef PubMed.
- Y.-S. Xie, D. Kumar, V. D. V. Bodduri, P. S. Tarani, B.-X. Zhao, J.-Y. Miao, K. Jang and D.-S. Shin, Tetrahedron Lett., 2014, 55, 2796 CrossRef.
- For Pt catalyzed examples, see:
(a) A. Fürstner and P. W. Davies, J. Am. Chem. Soc., 2005, 127, 15024 CrossRef PubMed;
(b) C. A. Witham, W.-Y. Huang, C. K. Tsung, J. N. Kuhn, G. A. Somorjai and F. D. Toste, Nat. Chem., 2010, 2, 36 CrossRef PubMed;
(c) Y.-M. Li, J. H. Liu, C. A. Witham, W. Y. Huang, M. A. Marcus, S. C. Fakra, P. Alayoglu, Z.-W. Zhu, C. M. Thompson, A. Arjun, K. Lee, E. Gross, F. D. Toste and G. A. Somorjai, J. Am. Chem. Soc., 2011, 133, 13527 CrossRef PubMed.
- For Pd catalyzed examples, see:
(a) Y. Liang, S. Tang, X.-D. Zhang, L.-Q. Mao, Y.-X. Xie and J.-H. Li, Org. Lett., 2006, 8, 3017 CrossRef PubMed;
(b) W.-Y. Huang, J.-H. Liu, P. Alayoglu, Y.-M. Li, C. A. Witham, C.-K. Tsung, F. D. Toste and G. A. Somorjai, J. Am. Chem. Soc., 2010, 132, 16771 CrossRef PubMed;
(c) M. Kim, S. Lee, K. Kim, D. Shin, H. Kim and H. Song, Chem. Commun., 2014, 50, 14938 RSC;
(d) P. Rubio-Marques, M. A. Rivero-Crespo, A. Leyva-Perez and A. Corma, J. Am. Chem. Soc., 2015, 137, 11832 CrossRef PubMed.
- Y. Zhang, Z.-J. Xin, J.-J. Xue and Y. Li, Chin. J. Chem., 2008, 26, 1461 CrossRef.
- For Au catalyzed examples, see:
(a) V. Belting and N. Krause, Org. Lett., 2006, 8, 4489 CrossRef PubMed;
(b) A. S. K. Hashmi, T. D. Ramamurthi and F. Rominger, Adv. Synth. Catal., 2010, 352, 971 CrossRef;
(c) O. S. Morozov, A. V. Lunchev, A. A. Bush, A. A. Tukov, A. F. Asachenko, V. N. Khrustalev, S. S. Zalesskiy, V. P. Ananikov and M. S. Nechaev, Chem.–Eur. J., 2014, 20, 6162 CrossRef PubMed;
(d) L.-B. Kong, R. Ganguly, Y.-X. Li and R. Kinjo, Chem. Sci., 2015, 6, 2893 RSC;
(e) Z.-H. Xia, O. Khaled, V. Mouriès-Mansuy, C. Ollivier and L. Fensterbank, J. Org. Chem., 2016, 81, 7182 CrossRef PubMed;
(f) J. Zhao, S. C. Nguyen, R. Ye, B.-H. Ye, H. Weller, G. A. Somorjai, A. P. Alivisatos and F. D. Toste, ACS Cent. Sci., 2017, 3, 482 CrossRef PubMed.
- For Rh catalyzed examples, see:
(a) B. M. Trost and A. McClory, Angew. Chem., Int. Ed., 2007, 46, 2074 CrossRef PubMed;
(b) N. Isono and M. Lautens, Org. Lett., 2009, 11, 1329 CrossRef PubMed;
(c) A. Boyer, N. Isono, S. Lackner and M. Lautens, Tetrahedron, 2010, 66, 6468 CrossRef;
(d) A. Sarbajna, P. Pandey, S. M. W. Rahaman, K. Singh, A. Tyagi, P. H. Dixneuf and J. K. Bera, ChemCatChem, 2017, 9, 1397 CrossRef.
- X.-W. Li, A. R. Chianese, T. Vogel and R. T. Carbtree, Org. Lett., 2005, 7, 5437 CrossRef PubMed.
- M. Nakamura, L. Ilies, S. Otsubo and E. Nakamura, Angew. Chem., Int. Ed., 2006, 45, 944 CrossRef PubMed.
- For Cu catalyzed examples, see:
(a) K. Hiroya, S. Itoh and T. Sakamoto, Tetrahedron, 2005, 61, 10958 CrossRef;
(b) M. Nakamura, L. Ilies, S. Otsubo and E. Nakamura, Org. Lett., 2006, 8, 2803 CrossRef PubMed.
- C. E. Castro, E. J. Gaughan and D. C. Owsley, J. Org. Chem., 1966, 31, 4071 CrossRef.
- For Pd catalyzed one-pot synthesis of 2-substituted benzo[b]furans, see:
(a) A. Arcadi, F. Marinelli and S. Cacchi, Synthesis, 1986, 9, 749 CrossRef;
(b) M. C. Kabalka, L. Wang and R. M. Pagni, Tetrahedron, 2001, 57, 8017 CrossRef;
(c) M. A. Berliner, E. M. Cordi, J. R. Dunetz and K. E. Price, Org. Process Res. Dev., 2010, 1, 180 CrossRef;
(d) J.-R. Wang and K. Manabe, J. Org. Chem., 2010, 75, 5340 CrossRef PubMed;
(e) M. Thevenin, S. Thoret, P. Grellier and J. Dubois, Bioorg. Med. Chem., 2013, 21, 4885 CrossRef PubMed;
(f) R. Zhou, W. Wang, Z.-J. Jiang, K. Wang, X.-L. Zheng, H.-Y. Fu, H. Chen and R.-X. Li, Chem. Commun., 2014, 50, 6023 RSC;
(g) M. Yamaguchi, T. Akiyama, H. Sasou, H. Katsumata and K. Manabe, J. Org. Chem., 2016, 81, 5450 CrossRef PubMed.
- For Cu catalyzed one-pot synthesis of 2-substituted benzo[b]furans, see:
(a) C. G. Bates, P. Saejueng, J. M. Murphy and D. Venkataraman, Org. Lett., 2002, 4, 4727 CrossRef PubMed;
(b) E. A. Jaseer, D. J. C. Prasad and G. Sekar, Tetrahedron, 2010, 66, 2077 CrossRef;
(c) R. Cano, M. Yus and D. J. Ramón, Tetrahedron, 2012, 68, 1393 CrossRef.
- For Fe catalyzed one-pot synthesis of 2-substituted benzo[b]furans, see:
(a) M. Carril, A. Correa and C. Bolm, Angew. Chem., Int. Ed., 2008, 47, 4862 CrossRef PubMed;
(b) J.-G. Yang, G.-D. Shen and D.-B. Chen, Synth. Commun., 2013, 43, 837 CrossRef.
- For phenols as the starting material, see:
(a) L. M. Geary and P. G. Hultin, Org. Lett., 2009, 11, 5478 CrossRef PubMed;
(b) S.-H. Wang, P.-H. Li, L. Yu and L. Wang, Org. Lett., 2011, 13, 5968 CrossRef PubMed;
(c) L. Arias, Y. Vara and F. P. Cossío, J. Org. Chem., 2012, 77, 266 CrossRef PubMed;
(d) U. Sharma, T. Naveen, A. Maji, S. Manna and D. Maiti, Angew. Chem., Int. Ed., 2013, 52, 12669 CrossRef PubMed.
-
(a) J. Hu, L. Liu, X.-C. Wang, Y.-Y. Hu, S.-D. Yang and Y.-M. Liang, Green Sustainable Chem., 2011, 1, 165 CrossRef;
(b) K. Damera, B. Ke, K. Wang, C. Dai, L. Wang and B. Wang, RSC Adv., 2012, 2, 9403 RSC.
- For base catalyzed examples, see:
(a) I. R. Siddiqui, M. A. Waseem, S. Shamim, Shireen, A. Srivastava and A. Srivastava, Tetrahedron Lett., 2013, 54, 4154 CrossRef;
(b) S.-X. Sun, J.-J. Wang, Z.-J. Xu, L.-Y. Cao, Z.-F. Shi and H.-L. Zhang, Tetrahedron, 2014, 70, 3798 CrossRef.
- S. Protti, M. Fagnoni and A. Albini, J. Org. Chem., 2012, 77, 6473 CrossRef PubMed.
- L. Liu, X.-Y. Ji, J.-Y. Dong, Y.-B. Zhou and S.-F. Yin, Org. Lett., 2016, 18, 3138 CrossRef PubMed.
- F. Lehmann, Synlett, 2004, 13, 2447 CrossRef.
Footnote |
† Electronic supplementary information (ESI) available: General experimental methods and procedures, characterization and spectra of substrates and products and other supplementary information. See DOI: 10.1039/c8ra03882a |
|
This journal is © The Royal Society of Chemistry 2018 |
Click here to see how this site uses Cookies. View our privacy policy here.