DOI:
10.1039/C8RA03278E
(Paper)
RSC Adv., 2018,
8, 28668-28675
Nickel(II)-catalyzed tandem C(sp2)–H bond activation and annulation of arenes with gem-dibromoalkenes†
Received
17th April 2018
, Accepted 25th July 2018
First published on 13th August 2018
Abstract
A nickel(II)/silver(I)-catalyzed tandem C(sp2)–H activation and intramolecular annulation of arenes with dibromoalkenes has been successfully achieved, which offers an efficient approach to the 3-methyleneisoindolin-1-one scaffold. Attractive features of this system include its low cost, ease of operation, and its ability to access a wide range of isoindolinones.
Over the past years, the transition-metal-catalyzed oxidative C–H/C–H cross-coupling reaction has emerged as a useful, atom- and step-economic synthetic protocol to construct a series of important N-heterocycles.1 In this context, the synthesis of isoindolinones has attracted considerable attention owing to their interesting biological and pharmaceutical properties,2 as well as their usefulness as precursors for the synthesis of structurally diverse and complex molecules (Scheme 1).2c,3 Several methods have successfully been developed toward isoindolinone synthesis based on Pd,4 Cu,5 Ru,6 and Rh7 salts. Among these reactions, the oxidative coupling reactions of benzamides with alkenes4b,6,7a,f,g or alkynes5a,5d exhibit high atom economy and the application of this strategy to simple arenes is still largely underdeveloped.8 For instance, in 2015, Zhang's group9 revealed cobalt-catalyzed oxidative alkynylation and cyclization of simple arenes and terminal alkynes with silver-cocatalyst via 2-fold C–H bond and N–H bond cleavage and C–C bond and C–N bond formation. In 2016, Song's group10 developed a method of a cobalt(II)-catalyzed decarboxylative C–H activation/annulation of benzamides and alkynyl carboxylic acids and nickel(II)-catalyzed C(sp2)–H alkynylation/annulation cascade with terminal alkynes to synthesize 3-methyleneiso-indolin-1-ones. Zhang also reported a nickel-catalyzed oxidative alkynylation with amides and terminal acetylenes.10c In addition, from an environmentally point of view, in 2015, wei's group11 described an operationally simple, Pd-catalyzed C–H functionalization for the synthesis of important and useful isoindolinones from readily available carboxamides and carboxylic acids or anhydrides. The protocol avoided the use of excess oxidants including benzoquinone, Cu(OAc)2, or Ag2CO3 of previous all the reactions, thus generating stoichiometric amounts of undesired wastes.
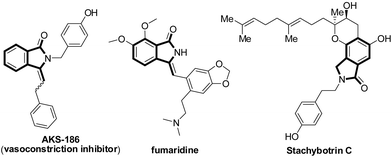 |
| Scheme 1 Representative isoindolinones with biological and pharmaceutical. | |
To our knowledge, the synthesis of alkynes is among the most fundamental and important synthetic transformations due to the unique reactivity of alkynes including addition, oxidation, reduction, and in particular cyclization.12 However, the lack of reactivity of alkynes, more electron-deficient than the corresponding alkenes, makes it harder to couple them with heteroarenes. As a consequence, terminal alkyne precursors have been developed to facilitate acetylene exchange.13 Halogenoalkynes,14 hypervalent alkynyliodoniums,15acetylenic sulfones,16 copper acetylides17 and α,β-ynoic acids18 allowed the generation of more activated alkyne moieties thus broadening the applications of direct alkynylation reactions to heterocycles. Among these alternatives, gem-dihaloalkenes emerged as more efficient coupling partners than the corresponding monohalogenated alkynes along with being inexpensive and readily-available.19 Indeed, the two geminal halogen atoms on the alkenyl carbon enhance the reactivity of metal complexes thus facilitating cross coupling reactions.20 Stable and readily-available 1,1-dibromo-1-alkenes and our interests in the C–H activation21 led us to consider using these reagents in the C–H functionalization to construct the valuable isoindolinones. We can envision that the abundance and structural diversity of the aldehydes (used the preparation of gem-dibromoethylenes via wittig reaction) as well as the merits of C–H functionalization would make the synthetic methods desirable and attractive. Herein, we wish to disclose the nickel(II)/silver(I)-mediated tandem transformation involving sequential C(sp2)–H/C(sp2)–H alkynylation and intramolecular annulation of unactivated arenes with dibromoethylenes with the assistance of 8-aminoquinoline (Scheme 2). These features of this approach operational simplicity, a wide-ranging substrate scope, and tolerance of various synthetically useful functional groups.
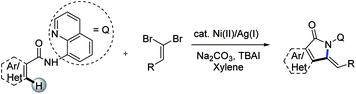 |
| Scheme 2 Nickel(II)/silver(I)-catalyzed alkynylation/annulation of arenes with dibromoalkenes. | |
Results and discussion
Initially, our investigation to explore the Ni(II)-catalyzed C sp2–H activation began with the reaction of N-(quinolin-8-yl) benzamide (1a) and (2,2-dibromovinyl) benzene (2a) in the presence of selected catalysts as shown in Table 1. Gratifyingly, (Z)-3-benzylidene-2-(quinolin-8-yl) isoindolin-1-one 3a was obtained in 54% yield when NiCl·6H2O (10 mol%) was employed in xylene at 140 °C for 12 h (Table 1, entry 1). In order to search out an appropriate condition, we firstly screened a series of Ni(II) salts and Ni(PPh3)2Cl2 (10 mol%) was chosen as the superior catalyst to give the corresponding product with a yield of 69% (entry 4). Moreover, the replacement of Ni(II) salts with Cu(OAc)2 and Co(OAc)2·4H2O gave a slightly less yield (entries 5 and 6). These results reconfirmed that Ni(PPh3)2Cl2 is the optimal nickel catalyst source with a great advantage that other metal catalysts cannot match. Subsequently, the solvent effect was also evaluated and the promotional effect of the common solvents, such as DMF, DMSO, dioxane, and MeCN decreased drastically except chlorobenzene delivered 3a in up to 68% yield (entries 8–11). We suppose that the enhanced reactivity with aromatic solvent arises in part from its coordination with nickel catalyst, which would facilitate the formation of nickel chelate, intermediate. To further improve the conversion, we turned our attention to the oxidant effect and here we screened a number of silver(I) salts and another oxidant Cu(OAc)2, which showed Ag2CO3 was the best choice which promoted the formation of ring-closing reaction to give the desired product (entries 12–16). Next, we investigated the remarkable effect of additives using sodium iodide and different ammonium salts, however the reaction even did not work except TBAB with 58% yield, which implicated that the main role of TBAI in this transformation may be the phase transfer catalyst (entries 17–20). And finally, we examined the reaction under argon and oxygen atmosphere, obtained 37% and 41% yield respectively, which indicated the influence of gas atmosphere for the reaction was very low (entries 22 and 23). In addition, no reaction was observed in the absence of the nickel catalyst or silver salt and additive, indicating the necessity of both catalyst, Ag(I) salts and additives for the reaction (entries 7, 15, 21).
Table 1 Optimization of the reaction conditiona
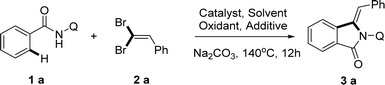
|
Entry |
Catalyst |
Solvent |
Oxidant |
Additive |
Yield (%)b |
Reaction condition: compound 1a (0.1 mmol), 2a (0.2 mmol), catalyst (10 mol%), Na2CO3 (2 equiv.), oxidant (4 equiv.), additive (3 equiv.), solvent (2.0 mL), under air, 110 °C, 12 h. Estimated by 1H NMR spectroscopy using CH2Br2 as an internal reference, Q = quinolin-8-yl. Under Ar. Under O2. |
1 |
NiCl·6H2O |
Xylene |
Ag2CO3 |
TBAI |
54 |
2 |
Ni(acac)2 |
Xylene |
Ag2CO3 |
TBAI |
62 |
3 |
Ni(OTf)2 |
Xylene |
Ag2CO3 |
TBAI |
52 |
4 |
Ni(PPh3)2Cl2 |
Xylene |
Ag2CO3 |
TBAI |
73 |
5 |
Cu(OAc)2 |
Xylene |
Ag2CO3 |
TBAI |
42 |
6 |
Co(OAc)2·4H2O |
Xylene |
Ag2CO3 |
TBAI |
31 |
7 |
— |
Xylene |
Ag2CO3 |
TBAI |
0 |
8 |
Ni(PPh3)2Cl2 |
DMF |
Ag2CO3 |
TBAI |
Trace |
9 |
Ni(PPh3)2Cl2 |
DMSO |
Ag2CO3 |
TBAI |
41 |
10 |
Ni(PPh3)2Cl2 |
PhCl |
Ag2CO3 |
TBAI |
68 |
11 |
Ni(PPh3)2Cl2 |
Dioxane |
Ag2CO3 |
TBAI |
56 |
12 |
Ni(PPh3)2Cl2 |
Xylene |
AgOAc |
TBAI |
11 |
13 |
Ni(PPh3)2Cl2 |
Xylene |
Ag2O |
TBAI |
20 |
14 |
Ni(PPh3)2Cl2 |
Xylene |
AgOTf |
TBAI |
0 |
15 |
Ni(PPh3)2Cl2 |
Xylene |
— |
TBAI |
0 |
16 |
Ni(PPh3)2Cl2 |
Xylene |
Cu(OAc)2 |
TBAI |
0 |
17 |
Ni(PPh3)2Cl2 |
Xylene |
Ag2CO3 |
NaI |
0 |
18 |
Ni(PPh3)2Cl2 |
Xylene |
Ag2CO3 |
TBAB |
58 |
19 |
Ni(PPh3)2Cl2 |
Xylene |
Ag2CO3 |
TBAF·4H2O |
0 |
20 |
Ni(PPh3)2Cl2 |
Xylene |
Ag2CO3 |
Me4NCl |
Trace |
21 |
Ni(PPh3)2Cl2 |
Xylene |
Ag2CO3 |
— |
0 |
22c |
Ni(PPh3)2Cl2 |
Xylene |
Ag2CO3 |
TBAI |
64 |
23d |
Ni(PPh3)2Cl2 |
Xylene |
Ag2CO3 |
TBAI |
61 |
Under the optimized conditions, we examined the applicability of the catalytic system for different directing groups, as shown in Scheme 3. Interestingly, N-(quinolin-8-yl) benzamide (1a-1) could promote the alkynylation/annulation reaction smoothly as well, delivering the corresponding isoindolinone in 73% yield, with high selectivity. Nevertheless, other common directing groups including the monodentate group, N-methoxybenzamide (1a-2), N-(naphthalen-1-yl) benzamide (1a-3), the bidentate coordinating groups, N-(pyridin-2-yl) benzamide (1a-4) and N-(pyridin-1-oxide-2-yl) benzamide (1a-5) were incapable of promoting the reaction. These control experiments showed the indispensable role of the 8-aminoquinoline moiety for this reaction.
 |
| Scheme 3 The effect of directing groups for the alkynylation/annulation reaction under standard reaction conditions. | |
Next, we set out to explore the scope of N-(quinolin-8-yl) benzamide partners as summarized in Scheme 4. To our delight, the reaction system could accelerate the tandem reaction of a wide array of N-(quinolin-8-yl) benzamides with (2,2-dibromovi-nyl)benzene, delivering a series of functionalized 3-methyleneisoindolin-1-ones in moderate to excellent yields. The positions of substituent on arenes had a remarkable effect on the transformation. While the substituent was installed on the ortho-position of the aromatic ring, the reaction did not proceed smoothly with only 31% yield (3ba–ca, 3ga–ha), which implicated that steric hindrance has a huge effect on the transformation. N-(Quinolin-8-yl) benzamides bearing the synthetically valuable groups, such as F, Cl, NO2 and CF3, were also tolerated as well in this transformation, delivering the corresponding products (3ba–fa) in moderate yields. Notably, the protocol was also compatible with heterocyclic substrates, liberating 3ka in acceptable yields (63.5%).
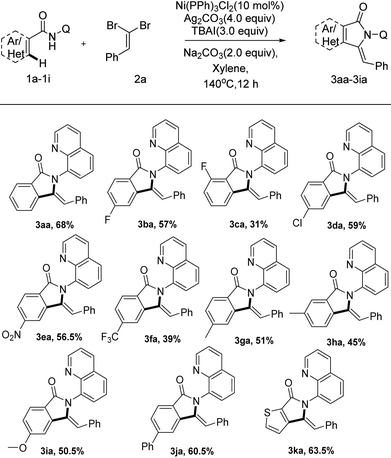 |
| Scheme 4 Scope of N-(quinolin-8-yl) benzamidesa,b. aReaction condition: compound 1a (0.1 mmol), 2a (0.2 mmol), Ni(PPh3)2Cl2 (10 mol%), Na2CO3 (2 equiv.), Ag2CO3 (4 equiv.), TBAI (3 equiv.), solvent (2.0 mL), under air, 140 °C, 12 h. bIsolated yield of 3 by flash column chromatography. Q = quinolin-8-yl, TBAI = tetrabutylammonium iodide. | |
Subsequently, we investigated a series of various dibromoalkenes under the optimized conditions as displayed in Scheme 5. Electron-rich and electron-deficient gem-dibromo olefins were all successfully engaged in this transformation (3ab–3ah). Dibromoalkenes bearing both electron-rich groups (Me, OMe and N-propyl substitutions) allowed better results furnishing alkynylation and cyclization products (3ae–3ah) between 54 to 81% isolated yields. The steric hindrance of the ortho-position on aromatic ring had an apparent effect on the reaction. Comparing with the gem-dibromoalkenes bearing substituents on the para-site (3ab, 58.5%; 3af, 70%), the ortho-substituted ones gave lower yields (3ac, 44%; 3ae, 54.5%). Additionally, we were pleased to notice that alkyl gem-dibromoalkenes were also tolerated under the catalytic system, delivering 3-methyleneisoindolin-1-ones in acceptable yields (3ai, 62.5%; 3aj, 69%), respectively.
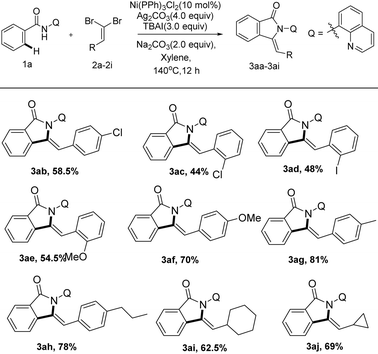 |
| Scheme 5 Scope of various dibromoalkenesa,b. aReaction condition: compound 1a (0.1 mmol), 2a (0.2 mmol), Ni(PPh3)2Cl2 (10 mol%), Na2CO3 (2 equiv.), Ag2CO3 (4 equiv.), TBAI (3 equiv.), solvent (2.0 mL), under air, 140 °C, 12 h. bIsolated yield of 3 by flash column chromatography. Q = quinolin-8-yl, TBAI = tetrabutylammonium iodide. | |
Very recently, the transition of metal-catalyzed tandem C–H activation and annulation of arene with terminal alkyne has been reported by many researchers, especially in which the Co(II)/Co(III) and Ni(I)/Ni(III) catalytic cycle was proved.9,22a On the basis of the above results of experiments and relevant literatures.22 We speculated that the reaction might also proceed through a Ni(I)/Ni(III) catalytic process and the plausible reaction mechanism has been proposed in Scheme 6. The catalytic cycle initiates with the oxidation of Ni(II) by Ag2CO3 to give Ni(III) species, which activated the inert sp2 C–H bond of N-(quinolin-8-yl)benzamide (1a) to generate the key intermediate II. Subsequently, the attack of the corresponding bromoalkyne via dehydrobromination of the dibromoalkene into intermediate II to oxidize addition gives the essential intermediate III, which undergoes the reductive elimination to give the alkynylated product IV and liberate the Ni(II) species. The oxidation of Ni(II) to Ni(III) by silver salts continues the cycle. And the ortho-alkynyl amide IV occurs rapidly intramolecular cyclization in the presence of Ag2CO3 and TBAI to give the 3-methyleneisoindolin-1-one products 3aa. Additionally, the possible pathway of a part of products is through the radical exchange of intermediate II with alkynyl radical and subsequent reductive elimination to give the alkynylated product IV through a Ni(III)/Ni(I) mechanism.22
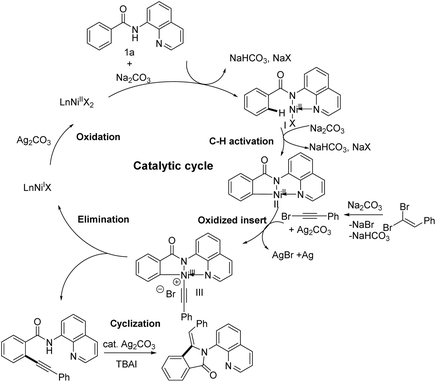 |
| Scheme 6 Plausible mechanism. | |
Conclusions
In conclusion, we have developed an efficient, operationally simple, and scalable nickel/silver-catalyzed unactivated C sp2–H bond activation and cyclization for the synthesis of isoindolinones divergently with excellent selectivity from readily available gem-dibromoalkenes. This protocol enables operational convenience with good tolerance of various aromatic amides and dibromoalkenes. Furthermore, this practical methodology may provide insight into the development of transition metal catalyzed C–H functionalization and further complement existing synthetic methods.
Experimental
General information
Unless otherwise noted, all of the reagents were purchased from commercial suppliers and used without purification. All product mixtures were analyzed by thin layer chromatography glass-backed silica TLC plates with a fluorescent indicator from Branch of Qingdao Haiyang Chemical CO. LTD. UV-active compounds were detected with a UV lamp (λ = 254 nm). For flash column chromatography, silica gel (200–300 mesh) was used as stationary phase. 1H NMR spectra ere recorded on a Bruker Advance III 400 MHz spectrometer in deuterated chloroform. The chemical shifts (δ) are reported in parts per million relatives to tetramethylsilane. The multiplicities of signals are designated by the following abbreviations: s (singlet), d (doublet), t (triplet), q (quartet), m (multiplet). Coupling constants (J) are given in hertz. 13C NMR spectra were recorded using a 100 MHz spectrometer. The chemical shifts are reported relative to residual CHCl3 (δ C = 77.00 ppm). High resolution mass spectra (HRMS) were measured with a Waters Micromass GCT instrument, accurate masses were reported for the molecular ion ([M]+ or [M + H]+).
General procedure for the preparation of carboxamides
To the solution of carboxylic acid (10 mmol) and 10 drops of DMF in 30 mL dry DCM at 0 °C, oxalyl chloride (20 mmol) was added dropwise under Ar. The mixture was then warm to r.t and stirred for another 5 h. The solvent was removed under vacuum to give crude acid cholid, which was used directly for next step without further purification.
To the mixture of 8-aminoquinoline (10 mmol) and Et3N (12 mmol) in dry DCM (30 mL) at 0 °C, the crude acid chloride obtained from previous step in 20 mL dry DCM was added dropwise. The mixture was then warm to r.t and stirred overnight. The reaction was quenched with H2O. The mixture was extracted, washed with saturated NaHCO3 solution. The combined organic layers were dried (MgSO4) and concentrated in vacuum and then purified by silica gel chromatography with a mixture of hexanes and ethyl acetate as the eluent to afford the corresponding amide products (reference: Chem. Commun., 2015, 51, 7863–7866).
General procedure for the preparation of gem-dibromoalkenes
To an ice cooled stirred solution of aldehyde (5.0 mmol) and carbon tetrabromide (2.5 g, 7.5 mmol) in anhydrous CH2Cl2 (40 mL) was added slowly a solution of triphenylphosphine (4.0 g, 15.0 mmol) in dichloromethane (30 mL) by several portions. The reaction was monitored by TLC. After the reaction was complete, the mixture was diluted with hexane (100 mL) and purified directly by column chromatography on silica gel. If it is not specified, hexane was used as an eluent for the column chromatography (reference: RSC Adv., 2014, 4, 2322–2326).
Typical procedure for copper(II)/silver(I)-catalyzed sequential alkynylation and annulation of arenes with gem-dibromoalkenes
A mixture of N-(quinolin-8-yl) benzamides (1, 24.8 mg, 0.1 mmol), Ni(PPh3)2Cl2(10 mol%, 6.2 mg), Ag2CO3 (110.3 mg, 0.4 mmol), TBAI (110.8 mg, 0.3 mmol), gem-dibromoalkenes (2, 0.2 mmol) and xylene (2.0 mL) was added to a 25 mL open tube. The tube was stirred at 140 °C for 12 h under air. The reaction was monitored by TLC. After the reaction was complete, then the reaction mixture was cooled to room temperature, and the reaction solution was treated with dilute p-toluenesulfonic acid for half hour. A saturated solution of sodium bicarbonate (10.0 mL) was added to the reaction tube and the pH of the solution in the reaction tube is neutralized to about 6–7. The mixture was extracted with ethyl acetate (3 × 15 mL), and the organic phase was combined and dried over Na2SO4 and was concentrated in vacuo. Then the mixture was subjected to column chromatography on silica gel using petroleum ether/ethyl acetate = 2
:
1 as eluent to afford the desired products (3). And then calculated the yields.
(Z)-3-Benzylidene-2-(quinolin-8-yl) isoindolin-1-one (3a)
1H NMR (400 MHz, CDCl3) δ 8.86 (dd, J = 4.2, 1.7 Hz, 1H), 7.98 (dd, J = 11.2, 4.6 Hz, 2H), 7.89 (d, J = 7.8 Hz, 1H), 7.69 (td, J = 7.6, 1.1 Hz, 1H), 7.60–7.54 (m, 2H), 7.48 (dd, J = 7.3, 1.4 Hz, 1H), 7.33–7.27 (m, 2H), 6.81 (s, 1H), 6.67 (dd, J = 11.1, 4.3 Hz, 1H), 6.58–6.51 (m, 4H). 13C NMR (101 MHz, CDCl3) δ 168.25, 150.47, 144.47, 138.77, 136.16, 135.92, 134.26, 133.62, 132.36, 130.14, 129.19, 128.94, 128.49, 128.38, 128.22, 126.39, 126.12, 125.75, 124.03, 121.33, 119.76, 107.49, 77.50, 77.18, 76.86. MS (ESI) m/z: 348.1 [M]+.
(Z)-3-Benzylidene-5-fluoro-2-(quinolin-8-yl) isoindolin-1-one (3b)
1H NMR (400 MHz, CDCl3) δ 8.85 (dd, J = 4.2, 1.7 Hz, 1H), 8.85 (dd, J = 4.2, 1.7 Hz, 1H), 7.99–7.95 (m, 2H), 7.59 (dd, J = 8.2, 1.3 Hz, 1H), 7.54 (dd, J = 8.5, 2.1 Hz, 1H), 7.48 (dd, J = 7.4, 1.4 Hz, 1H), 7.33–7.27 (m, 3H), 6.75 (s, 1H), 6.69 (dt, J = 8.2, 4.1 Hz, 1H), 6.54 (d, J = 4.9 Hz, 4H). 13C NMR (101 MHz, CDCl3) δ 167.21, 150.49, 144.32, 135.95, 135.34, 133.99, 133.14, 130.09, 128.94, 128.58, 128.16, 126.43, 126.34, 126.21, 125.75, 124.50, 121.37, 117.16, 116.92, 108.49, 106.98, 106.73, 77.44, 77.12, 76.81. MS (ESI) m/z: 366.1 [M]+.
(Z)-3-Benzylidene-5-chloro-2-(quinolin-8-yl) isoindolin-1-one (3c)
1H NMR (400 MHz, CDCl3) δ 8.84 (dd, J = 4.2, 1.7 Hz, 1H), 7.97 (dd, J = 8.3, 1.7 Hz, 1H), 7.92 (d, J = 8.1 Hz, 1H), 7.87 (d, J = 1.6 Hz, 1H), 7.59 (dd, J = 8.2, 1.4 Hz, 1H), 7.53 (dd, J = 8.1, 1.7 Hz, 1H), 7.48 (dd, J = 7.4, 1.4 Hz, 1H), 7.32–7.28 (m, 2H), 6.78 (s, 1H), 6.69 (dt, J = 8.8, 4.3 Hz, 1H), 6.54 (d, J = 4.5 Hz, 4H). 13C NMR (101 MHz, CDCl3) δ 167.27, 150.53, 144.35, 140.32, 138.84, 135.95, 135.19, 134.00, 133.19, 130.12, 129.60, 128.98, 128.66, 128.21, 126.79, 126.48, 126.41, 125.78, 125.37, 121.43, 120.18, 108.69, 77.48, 77.16, 76.84. MS (ESI) m/z: 382.0 [M]+.
(Z)-3-Benzylidene-5-methyl-2-(quinolin-8-yl)isoindolin-1-one (3d)
1H NMR (400 MHz, CDCl3) δ 8.84 (dd, J = 4.2, 1.6 Hz, 1H), 7.96 (dd, J = 8.3, 1.6 Hz, 1H), 7.87 (d, J = 7.8 Hz, 1H), 7.68 (s, 1H), 7.57 (d, J = 8.2 Hz, 1H), 7.47 (dd, J = 7.3, 1.2 Hz, 1H), 7.37 (d, J = 7.2 Hz, 1H), 7.31–7.27 (m, 2H), 6.77 (s, 1H), 6.67 (t, J = 6.4 Hz, 1H), 6.56–6.49 (m, 4H), 2.55 (s, 3H). 13C NMR (101 MHz, CDCl3) δ 168.33, 150.41, 144.47, 143.04, 139.14, 136.24, 135.90, 134.36, 133.73, 130.32, 130.13, 129.60, 128.92, 128.37, 128.21, 126.35, 126.01, 125.74, 123.87, 121.27, 120.07, 107.04, 77.46, 77.14, 76.83, 22.30. MS (ESI) m/z: 362.1 [M]+.
(Z)-3-Benzylidene-5-methoxy-2-(quinolin-8-yl) isoindolin-1-one (3e)
1H NMR (400 MHz, CDCl3) δ 8.84 (dd, J = 4.2, 1.7 Hz, 1H), 7.96 (dd, J = 8.3, 1.7 Hz, 1H), 7.89 (d, J = 8.4 Hz, 1H), 7.56 (dd, J = 8.2, 1.3 Hz, 1H), 7.47 (dd, J = 7.4, 1.4 Hz, 1H), 7.33 (d, J = 2.1 Hz, 1H), 7.30–7.26 (m, 2H), 7.09 (dd, J = 8.4, 2.2 Hz, 1H), 6.74 (s, 1H), 6.67 (ddd, J = 8.7, 4.7, 1.9 Hz, 1H), 6.57–6.50 (m, 4H), 3.96 (s, 3H). 13C NMR (101 MHz, CDCl3) δ 168.05, 163.62, 150.40, 144.52, 141.05, 136.20, 135.91, 134.41, 133.63, 130.16, 128.95, 128.34, 128.23, 126.38, 126.09, 125.76, 125.56, 121.38, 121.27, 116.58, 107.12, 103.81, 77.48, 77.16, 76.84, 55.94. MS (ESI) m/z: 378.1 [M]+.
(Z)-3-Benzylidene-6-methyl-2-(quinolin-8-yl) isoindolin-1-one (3f)
1H NMR (400 MHz, CDCl3) δ 8.84 (dd, J = 4.2, 1.7 Hz, 1H), 7.96 (dd, J = 8.3, 1.7 Hz, 1H), 7.79–7.78 (m, 1H), 7.76 (d, J = 7.9 Hz, 1H), 7.57 (dd, J = 8.2, 1.4 Hz, 1H), 7.50–7.47 (m, 1H), 7.46 (dd, J = 7.4, 1.4 Hz, 1H), 7.29 (dd, J = 4.4, 3.9 Hz, 1H), 7.26 (d, J = 8.2 Hz, 1H), 6.74 (s, 1H), 6.69–6.64 (m, 1H), 6.56–6.49 (m, 4H), 2.51 (s, 3H). 13C NMR (101 MHz, CDCl3) δ 168.42, 150.45, 144.56, 139.52, 136.40, 136.30, 135.90, 134.44, 133.83, 133.44, 130.17, 128.96, 128.63, 128.41, 128.28, 126.39, 126.00, 125.76, 124.12, 121.31, 119.59, 106.74, 77.48, 77.16, 76.84, 21.72. MS (ESI) m/z: 362.1 [M]+.
(Z)-3-Benzylidene-5-phenyl-2-(quinolin-8-yl) isoindolin-1-one (3g)
1H NMR (400 MHz, CDCl3) δ 8.86 (dd, J = 4.2, 1.7 Hz, 1H), 8.07–8.04 (m, 2H), 7.97 (dd, J = 8.3, 1.7 Hz, 1H), 7.78 (dd, J = 7.9, 1.4 Hz, 1H), 7.72 (dd, J = 8.1, 1.0 Hz, 2H), 7.59 (dd, J = 8.2, 1.3 Hz, 1H), 7.54–7.50 (m, 3H), 7.45 (d, J = 7.2 Hz, 1H), 7.33–7.29 (m, 2H), 6.88 (s, 1H), 6.71–6.65 (m, 1H), 6.58–6.51 (m, 4H). 13C NMR (101 MHz, CDCl3) δ 168.10, 150.49, 145.82, 144.48, 140.81, 139.42, 136.25, 135.95, 135.12, 134.33, 133.64, 130.23, 129.14, 129.00, 128.58, 128.51, 128.27, 127.69, 127.28, 126.43, 126.16, 125.81, 124.47, 121.36, 118.54, 107.58, 77.48, 77.16, 76.84. MS (ESI) m/z: 424.1 [M]+.
(Z)-3-Benzylidene-5-nitro-2-(quinolin-8-yl) isoindolin-1-one (3h)
1H NMR (400 MHz, CDCl3) δ 8.83 (dd, J = 4.2, 1.7 Hz, 1H), 8.76 (d, J = 1.8 Hz, 1H), 8.42 (dd, J = 8.3, 1.9 Hz, 1H), 8.15 (d, J = 8.3 Hz, 1H), 8.00 (dd, J = 8.3, 1.7 Hz, 1H), 7.63 (dd, J = 8.2, 1.3 Hz, 1H), 7.51 (dd, J = 7.4, 1.4 Hz, 1H), 7.36–7.31 (m, 2H), 6.97 (s, 1H), 6.76–6.70 (m, 1H), 6.56 (d, J = 4.7 Hz, 4H). 13C NMR (101 MHz, CDCl3) δ 166.11, 150.86, 150.64, 144.02, 139.58, 136.09, 134.65, 133.49, 132.79, 132.59, 130.03, 129.03, 128.17, 126.81, 126.59, 125.82, 125.28, 124.09, 121.61, 115.70, 110.61, 77.48, 77.16, 76.84. MS (ESI) m/z: 393.1 [M]+.
(Z)-3-(4-Chlorobenzylidene)-2-(quinolin-8-yl) isoindolin-1-one (3i)
1H NMR (400 MHz, CDCl3) δ 8.83 (dd, J = 4.2, 1.7 Hz, 1H), 8.04–7.97 (m, 2H), 7.87 (d, J = 7.8 Hz, 1H), 7.69 (dd, J = 13.6, 7.3 Hz, 2H), 7.58 (t, J = 7.5 Hz, 1H), 7.51 (d, J = 7.3 Hz, 1H), 7.38–7.30 (m, 2H), 6.71 (s, 1H), 6.50–6.43 (m, 4H). 13C NMR (101 MHz, CDCl3) δ 168.13, 150.54, 144.38, 138.52, 136.86, 136.01, 134.17, 132.49, 132.09, 131.91, 130.25, 129.43, 129.38, 129.00, 128.62, 128.39, 126.36, 125.89, 124.14, 121.54, 119.81, 105.91, 77.48, 77.16, 76.84. MS (ESI) m/z: 382.0 [M]+.
(Z)-3-(2-Chlorobenzylidene)-2-(quinolin-8-yl) isoindolin-1-one (3j)
1H NMR (400 MHz, CDCl3) δ 8.89 (dd, J = 4.2, 1.6 Hz, 1H), 8.02–7.92 (m, 3H), 7.71 (t, J = 7.6 Hz, 1H), 7.62–7.54 (m, 3H), 7.34–7.28 (m, 2H), 6.86 (d, J = 8.0 Hz, 1H), 6.71 (s, 1H), 6.62 (t, J = 7.7 Hz, 1H), 6.35 (d, J = 7.7 Hz, 1H), 6.14 (t, J = 7.5 Hz, 1H). 13C NMR (101 MHz, CDCl3) δ 168.24, 150.79, 144.49, 138.40, 137.24, 135.85, 133.82, 133.01, 132.50, 132.44, 130.49, 130.00, 129.52, 128.76, 128.62, 128.54, 127.89, 127.72, 125.77, 124.34, 124.09, 121.39, 120.13, 104.37, 77.48, 77.16, 76.84. MS (ESI) m/z: 382.0 [M]+.
(Z)-3-(2-Iodobenzylidene)-2-(quinolin-8-yl) isoindolin-1-one (3k)
1H NMR (400 MHz, CDCl3) δ 8.94 (dd, J = 3.3, 0.8 Hz, 1H), 8.02–7.97 (m, 2H), 7.95 (d, J = 7.8 Hz, 1H), 7.71 (t, J = 7.6 Hz, 1H), 7.60 (d, J = 8.1 Hz, 1H), 7.58–7.55 (m, 1H), 7.54 (s, 1H), 7.35 (d, J = 6.8 Hz, 1H), 7.33–7.26 (m, 2H), 6.58 (s, 1H), 6.39–6.33 (m, 2H), 6.21 (t, J = 7.5 Hz, 1H). 13C NMR (101 MHz, CDCl3) δ 168.23, 150.99, 144.46, 138.38, 137.86, 137.25, 136.58, 135.93, 133.80, 132.51, 130.14, 129.87, 129.50, 128.76, 128.64, 128.48, 127.81, 125.79, 125.75, 124.12, 121.42, 120.03, 110.49, 99.83, 77.48, 77.16, 76.84. MS (ESI) m/z: 474.0 [M]+.
(Z)-3-(2-Methoxybenzylidene)-2-(quinolin-8-yl) isoindolin-1-one (3l)
1H NMR (400 MHz, CDCl3) δ 8.86 (dd, J = 4.2, 1.7 Hz, 1H), 7.99–7.98 (m, 1H), 7.97–7.95 (m, 1H), 7.92 (d, J = 7.8 Hz, 1H), 7.67 (td, J = 7.6, 1.1 Hz, 1H), 7.55 (td, J = 7.9, 4.1 Hz, 2H), 7.48 (dd, J = 7.3, 1.4 Hz, 1H), 7.31–7.27 (m, 2H), 6.76 (s, 1H), 6.67 (ddd, J = 8.5, 7.6, 1.2 Hz, 1H), 6.30 (t, J = 8.2 Hz, 2H), 5.92 (td, J = 7.4, 0.7 Hz, 1H), 3.58 (s, 3H). 13C NMR (101 MHz, CDCl3) δ 168.33, 156.18, 150.34, 144.60, 138.77, 136.38, 135.95, 134.10, 132.22, 130.05, 130.00, 129.00, 128.79, 128.46, 128.39, 127.93, 125.52, 123.91, 122.52, 121.11, 120.10, 118.57, 108.84, 103.92, 77.48, 77.16, 76.84, 55.03. MS (ESI) m/z: 378.1 [M]+.
(Z)-3-(4-Methylbenzylidene)-2-(quinolin-8-yl) isoindolin-1-one (3n)
1H NMR (400 MHz, CDCl3) δ 8.83 (dd, J = 4.1, 1.5 Hz, 1H), 7.97 (t, J = 7.8 Hz, 2H), 7.85 (d, J = 7.8 Hz, 1H), 7.65 (t, J = 7.6 Hz, 1H), 7.59–7.51 (m, 2H), 7.45 (d, J = 7.4 Hz, 1H), 7.30–7.25 (m, 2H), 6.78 (s, 1H), 6.42 (d, J = 7.8 Hz, 2H), 6.31 (d, J = 7.8 Hz, 2H), 1.99 (s, 3H). 13C NMR (101 MHz, CDCl3) δ 168.17, 150.38, 144.52, 138.81, 135.82, 135.72, 134.38, 132.25, 130.53, 130.07, 128.99, 128.91, 128.28, 128.14, 128.06, 127.00, 125.72, 123.93, 121.29, 119.67, 107.67, 77.48, 77.16, 76.84, 20.95. MS (ESI) m/z: 362.1 [M]+.
(Z)-3-(4-Propylbenzylidene)-2-(quinolin-8-yl)isoindolin-1-one (3o)
1H NMR (400 MHz, CDCl3) δ 8.83 (dd, J = 4.2, 1.7 Hz, 1H), 8.00–7.92 (m, 2H), 7.86 (d, J = 7.8 Hz, 1H), 7.66 (td, J = 7.6, 1.1 Hz, 1H), 7.54 (dd, J = 7.8, 6.9 Hz, 2H), 7.47 (dd, J = 7.4, 1.3 Hz, 1H), 7.30–7.26 (m, 2H), 6.79 (s, 1H), 6.45 (d, J = 7.9 Hz, 2H), 6.32 (d, J = 8.0 Hz, 2H), 2.22 (t, J = 7.5 Hz, 2H), 1.42–1.32 (m, 2H), 0.80 (t, J = 7.3 Hz, 3H). 13C NMR (101 MHz, CDCl3) δ 168.18, 150.40, 144.54, 140.56, 138.80, 135.89, 135.81, 134.38, 132.26, 130.89, 130.11, 129.02, 128.88, 128.32, 128.08, 126.53, 125.72, 123.98, 121.25, 119.70, 107.73, 77.48, 77.16, 76.84, 37.53, 24.47, 13.80. MS (ESI) m/z: 390.1 [M]+.
(Z)-4-Benzylidene-5-(quinolin-8-yl)-4,5-dihydro-6H-thieno[2,3-c] pyrrol-6-one (3p)
1H NMR (400 MHz, CDCl3) δ 8.92 (dd, J = 4.2, 1.7 Hz, 1H), 8.08 (dd, J = 8.3, 1.7 Hz, 1H), 7.76 (d, J = 5.2 Hz, 1H), 7.72 (dd, J = 8.2, 1.3 Hz, 1H), 7.51 (dd, J = 7.3, 1.4 Hz, 1H), 7.44–7.39 (m, 1H), 7.37 (dd, J = 8.3, 4.2 Hz, 1H), 7.29 (d, J = 5.2 Hz, 1H), 7.09 (d, J = 6.9 Hz, 2H), 7.04–6.99 (m, 1H), 6.94 (t, J = 7.4 Hz, 2H), 6.79 (s, 1H). 13C NMR (101 MHz, CDCl3) δ 159.32, 151.10, 146.16, 145.52, 144.79, 136.93, 136.37, 136.27, 134.06, 130.99, 129.48, 129.00, 128.96, 128.87, 128.12, 127.41, 125.89, 124.66, 121.68, 104.66, 77.48, 77.16, 76.84. MS (ESI) m/z: 354.0 [M]+.
(Z)-3-(Cyclohexylmethylene)-2-(quinolin-8-yl) isoindolin-1-one (3q)
1H NMR (400 MHz, CDCl3) δ 8.89 (dd, J = 4.2, 1.7 Hz, 1H), 8.24 (dd, J = 8.3, 1.7 Hz, 1H), 8.00–7.92 (m, 2H), 7.80 (dd, J = 7.3, 1.4 Hz, 1H), 7.72 (d, J = 7.8 Hz, 1H), 7.69–7.64 (m, 1H), 7.60 (td, J = 7.6, 1.1 Hz, 1H), 7.48 (td, J = 7.5, 0.8 Hz, 1H), 7.42 (dd, J = 8.3, 4.2 Hz, 1H), 5.45 (d, J = 10.7 Hz, 1H), 1.72 (dt, J = 14.6, 6.7 Hz, 1H), 1.48–1.42 (m, 1H), 1.41–1.35 (m, 1H), 1.26 (d, J = 7.0 Hz, 2H), 1.23–1.17 (m, 1H), 1.15–1.07 (m, 1H), 0.93–0.85 (m, 2H), 0.74 (ddd, J = 12.0, 10.8, 3.5 Hz, 2H). 13C NMR (101 MHz, CDCl3) δ 168.48, 151.33, 145.68, 138.84, 136.21, 135.72, 134.04, 131.93, 130.67, 129.30, 129.27, 128.42, 128.39, 126.35, 123.76, 121.92, 119.40, 115.12, 77.48, 77.16, 76.84, 35.28, 33.32, 33.15, 25.69, 25.57, 25.45. MS (ESI) m/z: 353.1 [M]+.
(Z)-3-(Cyclopropylmethylene)-2-(quinolin-8-yl) isoindolin-1-one (3r)
1H NMR (400 MHz, CDCl3) δ 8.92 (dd, J = 4.2, 1.7 Hz, 1H), 8.19 (dd, J = 8.3, 1.7 Hz, 1H), 7.93 (d, J = 7.6 Hz, 1H), 7.90 (dd, J = 8.2, 1.3 Hz, 1H), 7.85 (dd, J = 7.3, 1.3 Hz, 1H), 7.66–7.62 (m, 2H), 7.57 (td, J = 7.7, 0.9 Hz, 1H), 7.46 (t, J = 7.7 Hz, 1H), 7.41 (dd, J = 8.3, 4.2 Hz, 1H), 5.13–5.06 (m, 1H), 0.40–0.32 (m, 2H), 0.28 (dd, J = 9.2, 6.5 Hz, 1H), 0.11 (ddd, J = 11.9, 8.6, 3.6 Hz, 1H), −0.04 (tdd, J = 8.5, 6.4, 4.5 Hz, 1H). 13C NMR (101 MHz, CDCl3) δ 168.07, 151.23, 145.52, 138.15, 136.16, 135.46, 135.28, 131.89, 130.66, 129.20, 129.14, 128.16, 127.82, 126.26, 123.74, 121.82, 118.97, 113.93, 77.48, 77.16, 76.84, 8.76, 8.66. MS (ESI) m/z: 312.1 [M]+.
Conflicts of interest
There are no conflicts to declare.
Acknowledgements
We are grateful to the project sponsored by National Science Foundation of P. R. China (No. 21102063) and Natural Science Foundation of Gansu Province of China (1107RJYA080).
Notes and references
-
(a) L. Ackermann, R. Vicente and A. R. Kapdi, Angew. Chem., Int. Ed., 2009, 48, 9792–9826 CrossRef PubMed;
(b) P. Thansandote and M. Lautens, Chem.–Eur. J., 2009, 15, 5874–5883 CrossRef PubMed;
(c) D. A. Colby, R. G. Bergman and J. A. Ellman, Chem. Rev., 2010, 110, 624–655 CrossRef PubMed;
(d) T. W. Lyons and M. S. Sanford, Chem. Rev., 2010, 110, 1147–1169 CrossRef PubMed;
(e) R. J. Jazzar, J. Hitce, A. Renaudat, J. Sofack-Kreutzer and O. Baudoin, Chem.–Eur. J., 2010, 16, 2654–2672 CrossRef PubMed;
(f) T. Satoh and M. Miura, Chem.–Eur. J., 2010, 16, 11212–11222 CrossRef PubMed;
(g) L. McMurray, F. O'Hara and M. J. Gaunt, Chem. Soc. Rev., 2011, 40, 1885–1898 RSC;
(h) C. S. Yeung and V. M. Dong, Chem. Rev., 2011, 111, 1215–1292 CrossRef PubMed;
(i) C. Liu, H. Zhang, W. Shi and A. Lei, Chem. Rev., 2011, 111, 1780–1824 CrossRef PubMed;
(j) S. H. Cho, J. Y. Kim, J. Kwak and S. Chang, Chem. Soc. Rev., 2011, 40, 5068–5083 RSC;
(k) J. Yamaguchi, A. D. Yamaguchi and K. Itami, Angew. Chem., Int. Ed., 2012, 51, 8960–9009 CrossRef PubMed;
(l) C. Zhang, C. Tang and N. Jiao, Chem. Soc. Rev., 2012, 41, 3464–3484 RSC;
(m) G. Song, F. Wang and X. Li, Chem. Soc. Rev., 2012, 41, 3651–3678 RSC;
(n) P. B. Arockiam, C. Bruneau and P. H. Dixneuf, Chem. Rev., 2012, 112, 5879–5981 CrossRef PubMed;
(o) N. Kuhl, N. Schroder and F. Glorius, Adv. Synth. Catal., 2014, 356, 1443–1460 CrossRef;
(p) Q. Zhang, K. Chen and B.-F. Shi, Synlett, 2014, 25, 1941–1945 CrossRef.
-
(a) M. H. Norman, D. J. Minick and G. C. Rigdon, J. Med. Chem., 1996, 39, 149–157 CrossRef PubMed;
(b) Z.-P. Zhuang, M.-P. Kung, M. Mu and H. F. Kung, J. Med. Chem., 1998, 41, 157–166 CrossRef PubMed;
(c) Y. Kato, M. Takemono and K. K. Achiwa, Chem. Pharm. Bull., 1999, 47, 529–535 CrossRef PubMed;
(d) T. R. Belliotti, W. A. Brink, S. R. Kesten, J. R. Rubin, D. J. Wustrow, K. T. Zoski, S. Z. Whetzel, A. E. Corbin, T. A. Pugsley, T. G. Heffner and L. D. Wise, Bioorg. Med. Chem. Lett., 1998, 8, 1499–1502 CrossRef PubMed;
(e) T. Lübbers, P. Angehrn, H. Gmünderb and S. Herziga, Bioorg. Med. Chem. Lett., 2007, 17, 4708–4714 CrossRef PubMed;
(f) D. K. Luci, E. C. Lawson, S. Ghosh, W. A. Kinney, C. E. Smith, J. Qi, Y. Wang, L. K. Minor and B. E. Maryanoff, Tetrahedron Lett., 2009, 50, 4958–4961 CrossRef;
(g) M. Lamblin, A. Couture, E. Deniau and P. Grandclaudon, Org. Biomol. Chem., 2007, 5, 1466–1471 RSC.
-
(a) V. Rys, A. Couture, E. Deniau and P. Grandclaudon, Tetrahedron, 2003, 59, 6615–6619 CrossRef;
(b) M. Jacolot, M. Jean, N. Tumma, A. Bondon, S. Chandrasekhar and P. Weghe, J. Org. Chem., 2013, 78, 7169–7175 CrossRef PubMed.
-
(a) K. Orito, A. Horibata, T. Nakamura, H. Ushito, H. Nagasaki, M. Yuguchi, S. Yamashita and M. Tokuda, J. Am. Chem. Soc., 2004, 126, 14342–14343 CrossRef PubMed;
(b) D.-D. Li, T.-T. Yuan and G.-W. Wang, Chem. Commun., 2011, 47, 12789–12791 RSC;
(c) Q. Yu, N. Zhang, J. Huang, S. Lu, Y. Zhu, X. Yu and K. Zhao, Chem.–Eur. J., 2013, 19, 11184–11188 CrossRef PubMed;
(d) N. Zhang, Q. Yu, R. Chen, J. Huang, Y. Xia and K. Zhao, Chem. Commun., 2013, 49, 9464–9466 RSC;
(e) Y. Xu, W. Hu, X. Tang, J. Jinwu Zhao, W. Wu and H. Jiang, Chem. Commun., 2015, 51, 6843–6846 RSC;
(f) X. Kou, Y. Li, L. Wu, X. Zhang, G. Yang and W. Zhang, Org. Lett., 2015, 17, 5566–5569 CrossRef PubMed.
-
(a) J. Dong, F. Wang and J. You, Org. Lett., 2014, 16, 2884–2887 CrossRef PubMed;
(b) H.-L. Wang, M. Shang, S.-Z. Sun, Z.-L. Zhou, B. N. Laforteza, H.-X. Dai and J.-Q. Yu, Org. Lett., 2015, 17, 1228–1231 CrossRef PubMed;
(c) W. Miura, K. Hirano and M. Miura, Org. Lett., 2015, 17, 4034–4037 CrossRef PubMed;
(d) K. Takamatsu, K. Hirano and M. Miura, Org. Lett., 2015, 17, 4066–4069 CrossRef PubMed;
(e) C. Yamamoto, K. Takamatsu, K. Hirano and M. Miura, J. Org. Chem., 2016, 81, 7675–7684 CrossRef PubMed.
-
(a) R. Manoharan and M. Jeganmohan, Chem. Commun., 2015, 51, 2929–2932 RSC;
(b) X. G. Li, M. Sun, K. Liu and P. N. Liu, Adv. Synth. Catal., 2015, 357, 395–399 CrossRef;
(c) M.-C. Reddy and M. Jeganmohan, Org. Lett., 2014, 16, 4866–4869 CrossRef PubMed.
-
(a) F. Wang, G. Song and X. Li, Org. Lett., 2010, 12, 5430–5433 CrossRef PubMed;
(b) C. Zhu and J. R. Falck, Chem. Commun., 2012, 48, 1674–1676 RSC;
(c) T. K. Hyster, K. E. Ruhl and T. Rovis, J. Am. Chem. Soc., 2013, 135, 5364–5367 CrossRef PubMed;
(d) B. Zhou, W. Hou, Y. Yang and Y. Li, Chem.–Eur. J., 2013, 19, 4701–4706 CrossRef PubMed;
(e) W. Hou, B. Zhou, Y. Yang, H. Feng and Y. Li, Org. Lett., 2013, 15, 1814–1817 CrossRef;
(f) Á. M. Martinez, N. Rodríguez, R. G. Arrayás and J. C. Carretero, Chem. Commun., 2014, 50, 6105–6107 RSC;
(g) S. Cai, C. Chen, P. Shao and C. Xi, Org. Lett., 2014, 16, 3142–3145 CrossRef PubMed;
(h) M. Fujioka, T. Morimoto, T. Tsumagari, H. Tanimoto, Y. Nishiyama and K. Kakiuchi, J. Org. Chem., 2012, 77, 2911–2923 CrossRef PubMed.
-
(a) N. Matsuyama, M. Kitahara, K. Hirano, T. Satoh and M. Miura, Org. Lett., 2010, 12, 2358 CrossRef PubMed;
(b) T. d. Haro and C. Nevado, J. Am. Chem. Soc., 2010, 132, 1512 CrossRef PubMed;
(c) Y. Wei, H. Zhao, J. Kan, W. Su and M. Hong, J. Am. Chem. Soc., 2010, 132, 2522 CrossRef PubMed;
(d) S. H. Kim, S. H. Park and S. Chang, Tetrahedron, 2012, 68, 5162 CrossRef.
- J.-T. Zhang, H. Chen, C. Lin, Z.-X. Liu, C. Wang and Y.-H. Zhang, J. Am. Chem. Soc., 2015, 137, 12990–12996 CrossRef PubMed.
-
(a) X.-Q. Hao, C. Du, X.-J. Zhu, P.-X. Li, J.-H. Zhang, J.-L. Niu and M.-S. Song, Org. Lett., 2016, 18, 3610–3613 CrossRef PubMed;
(b) X.-X. Zheng, C. Du, X.-M. Zhao, X. Zhu, J.-F. Suo, X.-Q. Hao, J.-L. Niu and M.-P. Song, J. Org. Chem., 2016, 81, 4002–4011 CrossRef PubMed;
(c) C. Lin, J. Zhang, Z. Chen, Y. Liu, Z. Liu and Y. H. Zhang, Adv. Synth. Catal., 2016, 358, 1778–1793 CrossRef.
- H.-W. Liang, W. Ding, K. Jiang, L. Shuai, Y. Yuan and Y. Wei, Org. Lett., 2015, 17, 2764–2767 CrossRef PubMed; .
-
(a) L. Ackermann, Acc. Chem. Res., 2014, 47, 281–295 CrossRef PubMed;
(b) R. Chinchilla and C. Nájera, Chem. Rev., 2014, 114, 1783–1826 CrossRef PubMed.
- J. P. Brand and J. Waser, Chem. Soc. Rev., 2012, 41, 4165–4179 RSC.
-
(a) F. Besselievre and S. Piguel, Angew. Chem., Int. Ed., 2009, 48, 9553–9556 CrossRef PubMed;
(b) N. Matsuyama, K. Hirano, T. Satoh and M. Miura, Org. Lett., 2009, 11, 4156–4159 CrossRef PubMed;
(c) Y. Ano, M. Tobisu and N. Chatani, J. Am. Chem. Soc., 2011, 133, 12984–12986 CrossRef PubMed;
(d) J. He, M. Wasa, K. S. L. Chan and J.-Q. Yu, J. Am. Chem. Soc., 2013, 135, 3387–3390 CrossRef PubMed;
(e) E. Brachet and P. Belmont, J. Org. Chem., 2015, 80, 7519–7529 CrossRef PubMed;
(f) N. Sauermann, M. J. Gonzalez and L. Ackermann, Org. Lett., 2015, 17, 5316–5319 CrossRef PubMed;
(g) J. Zheng, L. Huang, Z. Li, W. Wu, J. Li and H. Jiang, Chem. Commun., 2015, 51, 5894–5897 RSC;
(h) Y.-J. Liu, Y.-H. Liu, S.-Y. Yan and B.-F. Shi, Chem. Commun., 2015, 51, 6388–6391 RSC.
-
(a) J. P. Brand, J. Charpentier and J. Waser, Angew. Chem., Int. Ed., 2009, 48, 9346–9349 CrossRef PubMed;
(b) J. P. Brand and J. Waser, Angew. Chem., Int. Ed., 2010, 49, 7304–7307 CrossRef PubMed;
(c) Z.-Z. Zhang, B. Liu, C.-Y. Wang and B.-F. Shi, Org. Lett., 2015, 17, 4094–4097 CrossRef PubMed;
(d) R. Boobalan, P. Gandeepan and C.-H. Cheng, Org. Lett., 2016, 18, 3314–3317 CrossRef PubMed.
-
(a) J. Gong and P. L. Fuchs, J. Am. Chem. Soc., 1996, 118, 4486–4487 CrossRef;
(b) J. S. Xiang and P. L. Fuchs, Tetrahedron Lett., 1996, 37, 5269–5272 CrossRef.
- C. Theunissen and G. Evano, Org. Lett., 2014, 16, 4488–4491 CrossRef PubMed.
-
(a) J. Kim, D. Kang, E. J. Yoo and P. H. Lee, Eur. J. Org. Chem., 2013, 35, 7902–7906 CrossRef;
(b) K. Park and S. Lee, RSC Adv., 2013, 3, 14165–14182 RSC.
-
(a) P. B. Berciano, S. Lebrequier, F. Besselievre and S. Piguel, Org. Lett., 2010, 12, 4038–4041 CrossRef PubMed;
(b) L. Ackermann, C. Kornhaass and Y. Zhu, Org. Lett., 2012, 14, 1824–1826 CrossRef PubMed;
(c) G. C. Reddy, P. Balasubramanyam, N. Salvanna and B. Das, Eur. J. Org. Chem., 2012, 471–474 CrossRef;
(d) C. Shen, Y. Yang, Z. Liu and Y. Zhang, Synth. Commun., 2014, 44, 1970–1977 CrossRef;
(e) A. Srivastava, L. Aggarwal and N. Jain, ACS Comb. Sci., 2015, 17, 39–48 CrossRef PubMed.
-
(a) G. Chelucci, Chem. Rev., 2012, 112, 1344–2146 CrossRef PubMed;
(b) J. Aziz, T. Baladi and S. Piguel, J. Org. Chem., 2016, 81, 4122–4133 CrossRef PubMed;
(c) Y. Li, L. Cheng, Y. Shao, S. Jiang, J. Cai and N. Qing, Eur. J. Org. Chem., 2015, 4325–4329 CrossRef;
(d) W. Dai, H. Shi, X. Zhao and S. Cao, Org. Lett., 2016, 18, 4284–4287 CrossRef PubMed;
(e) R. T. Thornbury and F. D. Toste, Angew. Chem., Int. Ed., 2016, 55, 11629–11632 CrossRef PubMed.
-
(a) Z. Huang, R. Shang, Z.-R. Zhang, X.-D. Tan, X. Xiao and Y. Fu, J. Org. Chem., 2013, 78, 4551–4557 CrossRef PubMed;
(b) L. Liu, Y. Lv, Y. Wu, X. Gao, Z. Zeng, Y. Gao, G. Tang and Y. Zhao, RSC Adv., 2014, 4, 2322–2326 RSC;
(c) A.-K. Morri, Y. Thummala and V.-R. Doddi, Org. Lett., 2015, 17, 4640–4643 CrossRef PubMed;
(d) M. Zhao, L. Ming, J. Tang and X. Zhao, Org. Lett., 2016, 18, 416–419 CrossRef PubMed.
-
(a) Q.-Q. Yan, Z.-K. Chen, W.-L. Yu, H. Yin, Z.-X. Liu and Y.-H. Zhang, Org. Lett., 2015, 17, 2482–2485 CrossRef PubMed;
(b) X.-S. Wu, Y. Zhao and H.-B. Ge, J. Am. Chem. Soc., 2014, 136, 1789–1792 CrossRef PubMed;
(c) J. Yi, L. Yang, C.-G. Xia and F.-W. Li, J. Org. Chem., 2015, 80, 6213–6221 CrossRef PubMed;
(d) V. G. Landge, G. Jaiswal and E. Balaraman, Org. Lett., 2016, 18, 812–815 CrossRef PubMed;
(e) W.-F. Song, S. Lackner and L. Ackermann, Angew. Chem., Int. Ed., 2014, 53, 2477–2480 CrossRef PubMed;
(f) Y.-J. Liu, Z.-Z. Zhang, S.-Y. Yan, Y.-H. Liu and B.-F. Shi, Chem. Commun., 2015, 51, 7899–7902 RSC;
(g) B. Wang, C.-X. Lu, S.-Y. Zhang, G. He, W. A. Nack and G. Chen., Org. Lett., 2014, 16, 6260–6263 CrossRef PubMed.
Footnote |
† Electronic supplementary information (ESI) available. See DOI: 10.1039/c8ra03278e |
|
This journal is © The Royal Society of Chemistry 2018 |