DOI:
10.1039/C8RA01268G
(Paper)
RSC Adv., 2018,
8, 14201-14211
Differences in weathering pattern, stress resistance and community structure of culturable rock-weathering bacteria between altered rocks and soils
Received
9th February 2018
, Accepted 24th March 2018
First published on 17th April 2018
Abstract
In this study, we isolated and characterized rock-weathering bacteria from the surfaces of less and more altered tuffs, along with the adjacent soils, with respect to their rock weathering pattern, stress resistance, community structure, and the changes in these rocks and soils. Using a moderate-nutrition medium, we obtained 150 isolates from the rocks and soils. The rock-weathering patterns of the isolates were characterized using batch cultures that measure the quantity of Si, Al, and Fe released from tuff under aerobic conditions. Based on the potential of the bacterial influence on the element releases, the isolates could be grouped into highly, moderately, and least effective element solubilizers, respectively. Significantly more highly effective Al and Fe solubilizers were observed in the altered rocks, while the soils had more highly effective Si solubilizers. Furthermore, more isolates from the altered rocks significantly acidified the culture medium in the rock weathering process. Dynamic changes in the element release showed the distinct element releasing patterns of three selected isolates. More isolates from the altered rocks could grow at 4 °C or at 55 °C or at pH 4. Some isolates from the altered rocks could grow at pH 10 and with 10–15% (w/v) NaCl. The altered rocks and the soils existed in diverse and different highly weathering-specific culturable rock-weathering community structures. The changes in the culturable weathering communities between the altered rocks and the soils were attributable not only to major bacterial groups but also to a change in the minor population structure.
Introduction
Rock-weathering is one of the most important geochemical phenomena. Weathering processes result in the formation of soils and maintenance of soil productivity, the denudation of continents, and a long time-scale carbon cycle.1–6 Microbial communities, as free living cells or as in biofilms, are the first colonizers of newly exposed rocks. They may influence the rate of mineral weathering and the amount and quality of organic matter in the soils.7 Field observations and laboratory experiments demonstrate that microbes can accelerate or inhibit rock and mineral weathering reactions4,8–11 by producing acids and metal-complexing ligands.8,12 There is a wealth of knowledge concerning the mineral weathering roles, community structure and distribution of culturable bacteria isolated from various environments,13–16 however previous studies on mineral-weathering bacteria have focused on soil environments, especially on the nutrition-poor forest soil ecosystems.12,17–19 In terrestrial environments, microbial communities have been identified in Tatahouine meteorite and various rocks.20,21 They are thought to facilitate the extraction of elements that cause bio-weathering.11,22,23 Although the microbial community and diversity on Tatahouine meteorite, basalt rock, schist, and gneiss have been reported,7 no studies have been published that provide an overview of the diversity of rock-weathering bacterial communities on tuff surfaces and how these populations change between the different levels of altered rocks and soils. Furthermore, there is little understanding on the linking of each phylogenetic group with rock-weathering.24,25 Tuff is a soil-forming rock and is the subject of this study. It is thus important to study the diversity of rock-weathering microorganisms that have colonized tuff to evidence the species that may be involved in weathering processes.
To date, the culture-dependent approach remains the only way to study the rock-weathering ability of bacteria.6,15 However, it is thought that both culturable and unculturable bacteria play important roles in rock-weathering. In this study, we provide insight into rock-weathering by the culturable portion of the active bacteria on the altered rock surfaces as well as in the adjacent soils. We hypothesize that the different levels of altered rocks and soils colonize diverse and different rock-weathering bacterial communities, and that the rock-weathering potentials and patterns of the rock-weathering communities change with the degree of rock alteration. To test these hypotheses we isolated culturable rock-weathering bacteria to compare the weathering capacity and pattern and community structure between the altered rocks and the soils and between the less and more altered rocks. In this study, we aim to establish relationships between bacterial species and the element releasing potential of tuff and to evaluate the changes in the weathering patterns and communities of the rock-weathering bacteria among the altered rocks and the soils to further our understanding of the geomicrobiological roles which rock-weathering bacteria could play in rock-weathering, elemental mobilization and soil formation in the rock and soil systems.
Materials and methods
Rock and soil sample collection
The sampling site was located in Dongxiang County, Fuzhou, Jiangxi province (China) (28°23′ N, 116°62′ E). The rocks (tuffs) occurred as variably altered outcrops. The less (LR) and more (MR) altered rock samples were aseptically collected in triplicate (a total of 6 samples, 600 g of each sample, and the size of the rock samples was <2 cm in diameter) using autoclaved spatulas and were placed in sterile bags. At the same time, soil samples close to the rock samples (5 m from the rock samples) were also collected for comparison with the rock samples. All samples were immediately transported back to the laboratory, sieved (2 mm) within 48 h of collection, and stored at 4 °C for chemical analysis and bacterial isolation within 24 h.
Physicochemical analyses of samples
Rock and soil sample pH was measured with a pH meter (PHS-3CT) after equilibrating 5 g of dry samples with 10 mL of deionized water for 30 min. Organic matter of the samples was determined by a dichromate wet oxidation procedure.26 Available Si, Al, K, Fe, Ca, and Mg of the samples were extracted with M3 soil test extractant27 by shaking at 25 °C and 200 rpm for 15 min. The mixtures were then filtered through filter paper and the above element concentrations in the filtrates were measured using an inductively coupled plasma optical emission spectrometer (ICP-OES) (Optimal 2100 DV, Perkin Elmer).
To determine the mineral compositions of the rock and soil samples, the samples were analysed by X-ray diffraction (XRD). The samples were ground to a powder (<150 μm) in an agate mortar and analyzed by powder X-ray diffraction (XRD) using a Bruker Axs D8 Advance diffractometer with Co-Kα radiation (40 kV, 40 mA) in step scan mode between 2θ = 3 and 60° with a 2θ step of 0.05° and counting time of 5 s per step. In order to better present the level of weathering of the rock and soil samples, the samples (<2 μm) were also separated from the rock and soil samples by sedimentation according to Stokes’ law28 for X-ray diffraction analysis. A standard powder diffraction file database connected to a graphical terminal was used for mineral identification.
Bacterial enumerations and isolation
Culture techniques were employed to determine bacterial counts in the rock and soil samples. Preliminary experiments showed that more bacterial colonies were obtained on sucrose-salts medium (SSM) agar plates than on Luria–Bertani (LB)29 or half-strength LB agar plates, which are often used for bacterial isolation and counting. SSM [1% sucrose, 0.1% (NH4)2SO4, 0.001% NaCl, 0.05% MgSO4·7H2O, 0.2% K2HPO4, 0.05% yeast extract, 0.001% CaCO3, and 2% agar at pH 7.2] was used to culture bacteria from the above samples.11 Rock and soil samples (10 g of each sample, wet weight) were added to flasks containing 100 mL of sterile physiological salt solution (0.85% NaCl) and shaken at 200 rpm for 30 min to allow the bacteria to detach from the rock and soil particles. The suspensions were then allowed to stand for about 10 min. Serial 10-fold dilutions of sample suspensions (10−3–10−5) were plated onto SSM agar plates to determine the total culturable bacteria. The plates were incubated for 7 days at 28 °C, and then the numbers of colonies per gram of dry rock and soil were calculated. One hundred and fifty bacteria (50 bacteria per rock and soil sample) were obtained from the above plates and purified on the same medium by streaking on fresh medium. The obtained bacteria were stored on slants to study the solubilization of tuff.
Rock dissolution experiment of the isolates
Tuff was used as the tested rock in order to evaluate the potential of the isolates in rock dissolution. The rock preparation for the dissolution experiment was carried out according to the method by Sheng et al.22 Briefly, tuff was crushed, ground, and passed through sieves. The rock powders were then ultrasonically cleaned, leached, and washed. The elemental composition of the rock [the rock was digested with HNO3/HClO4 (87/13 v/v) and the amounts of the elements were analyzed by ICP-OES] is as follows: SiO2 70.58%, Al2O3 14.05%, K2O 10.37%, Fe2O3 1.38%, Na2O 1.55%, CaO 0.95%, MgO 0.36%, and P2O5 0.12%. Based on the low nutrient Bushnell–Haas medium (BHM) (0.002% KCl, 0.015% MgSO4·7H2O, 0.008% NaH2PO4·2H2O, 0.009% Na2HPO4·2H2O, 0.0065% (NH4)2SO4, 0.01% KNO3, 0.002% CaCl2, and 0.2% glucose),18 a modified BHM (free of KCl and KNO3) was used to evaluate whether the isolates could release Si, Al, and Fe from tuff under low nutrient conditions. Considering the fact that much work was needed for showing the release of the elements from the rock over time by 150 bacterial isolates, we focused on showing the release of the elements by the isolates at one time point in this study. The bacterial inoculum was prepared based on the method by Zhao et al.11 Triplicate 250 mL polycarbonate Erlenmeyer culture flasks containing 40 mL of sterilized modified BHM (containing 0.20 g rock powders) were each inoculated with 0.5 mL of a bacterial suspension (108 cells mL−1). The flasks were incubated at 28 °C in a rotary shaker at 150 rpm for 7 days. Non-inoculated rock-amended medium was made as the control to determine the abiotic influence on rock dissolution. The dissolution of the rock in the presence of the isolates was monitored at 7 days of incubation. Samples (culture solutions) for chemical analyses were filtered through a 5 μm Millipore filter and 20 mL of the filtrate from each flask was then centrifuged at 10
000 rpm for 10 min in order to remove the cells and possible fine grained rock materials from the suspension. 5 mL of the supernatant was collected for pH determination and another 5 mL of the supernatant was acidified with HNO3 (final concentration 2% v/v) to avoid precipitation of dissolved chemical species and was analyzed for Si, Al, and Fe amounts by ICP-OES.
Dynamic changes in element release of the isolates
Based on the rock dissolution experiment, three rock-weathering isolates (L26, M23, and H41) (one isolate per rock and soil sample) were used to evaluate the dynamic changes in the element (Si, Al, K, and Fe) releases from tuff by the isolates. The rock dissolution experiment was performed as above. The element amounts and pH in the presence of the isolates were measured at 0, 1, 2, 4, 7, 10, 12, and 15 days of incubation. The element amounts were analyzed by ICP-OES.
Physiological and biochemical characteristics of the isolates
Liquid SSM was used to investigate the effects of salinity, temperature, and pH on the growth of the isolates according to the method by Sheng et al.22 The ranges of salinity, temperature, and pH were 1–15% (NaCl), 4–55 °C, and 4–10, respectively. Siderophore secretion by the isolates was detected by the “universal” method of Schwyn and Neilands30 using blue agar plates containing the dye chrome azurol S (CAS). Orange halos around the colonies on blue agar were indicative of siderophore excretion.
Identification of the isolates
Using the standard lysozyme–SDS–pronase protocol according to molecular cloning,31 genomic DNA was extracted from each isolate after growth in SSM to late exponential phase. The obtained crude DNA was purified using a DNA quick midi purification kit (TIANGEN Biotechnology Limited Company, Beijing) according to the manufacturer’s instructions. Then the 16S rRNA gene of the isolates was amplified with the universal bacterial 16S rRNA primers 27F (5′-AGAGTTTGATCCTGGCTCAG) and 1492R (5′-ACGGCTACCTTGTTACGACTT).32 The PCR reaction system contained: 2 μL of purified DNA extract, 0.2 μM of each primer, 12.5 μL Premix Ex Taq (containing DNA polymerase, buffer, and dNTP mixture) (TAKARA), and sterile deionized water to a final volume of 50 μL. The following cycle conditions were used: 95 °C for 5 min; 28 cycles of denaturation at 95 °C for 30 s; annealing at 55 °C for 30 s; extension at 72 °C for 90 s; and a final extension at 72 °C for 10 min. The presence of the PCR products was confirmed by electrophoresis on 1.5% agarose gels and staining with ethidium bromide.
Purified PCR products from the 16S rRNA genes of the isolates were sequenced on an ABI 3730×1 automated sequencer (Invitrogen) combined with a Sequencing Kit (BigDye Terminator) and the primers set as 27f and 1492r as well as M13-47 and RV-M. The resulting nucleotide sequences were blasted using the National Centre of Biotechnology Information (NCBI) database to obtain the closest species match. The phylogenetic affiliation was verified using the RDP classifier.33 The nucleotide sequences determined in this study have been deposited in the NCBI database. The accession numbers for the sequences of the cultured rock-weathering bacteria are JX848973 to JX849123.
Statistical analyses
One-way analysis of variance (ANOVA) and the Fisher’s Least Significant Difference test (Fisher’s LSD) (p < 0.05) were used to compare the averages of the less and more altered rock and soil parameters, the averages of the pH determinations, and the averages of the concentrations of Si, Al, and Fe released in the bacterial treatments with those from the control of the untreated medium. Statistical analyses were carried out using SAS 8.2 (Statistical Analysis System, USA).
Results
Characterization of the rock and soil samples
Physicochemical characterizations of the altered rock and soil samples are shown in Table 1. Significant changes in the organic matter and available K, Ca, and Mg amounts were observed among the less and more altered rock and soil samples. The pH was significantly higher in the less altered rock samples than in the more altered rock and soil samples. No significant difference in the pH between the more altered rock and soil samples was observed. The available Si, Al, and P amounts were significantly higher in the more altered rock samples than in the less altered rock and soil samples, however no significant change in the available Si and P amounts were observed between the less altered rock and soil samples. The available Fe content was significantly higher in the more altered rock and soil samples than in the less altered rock samples (Table 1).
Table 1 Organic matter, pH, cell number, and available element amounts of the rock and soil samples
|
Rock and soil samplesa |
Less altered rock |
More altered rock |
Soil sample |
If values are followed by the same letter within a line, then they are not significantly different (P > 0.05) according to Tukey’s test. |
Organic matter (g kg−1) |
8.8 ± 1.0c |
11.3 ± 1.2b |
19.2 ± 3.6a |
pH |
7.32 ± 0.17a |
4.63 ± 0.04b |
4.77 ± 0.10b |
![[thin space (1/6-em)]](https://www.rsc.org/images/entities/char_2009.gif) |
Available element amounts (mg kg−1) |
Fe |
134 ± 14b |
176 ± 26a |
223 ± 22a |
Si |
41.02 ± 3.60b |
52.66 ± 7.49a |
34.18 ± 8.55b |
Al |
1353 ± 341b |
6616 ± 1061a |
5959 ± 789a |
K |
46.3 ± 2.1c |
145 ± 18b |
310 ± 84a |
Ca |
771 ± 50a |
443 ± 12b |
281 ± 48c |
Mg |
163 ± 10a |
84 ± 12b |
48 ± 5c |
P |
23.97 ± 0.98b |
50.51 ± 9.24a |
27.61 ± 3.40b |
Bacterial count (×104 cfu g−1 fresh rock or soil) |
2.5 ± 0.22c |
157 ± 23b |
278 ± 30a |
Although the relative intensity of the diffractogram of the minerals varied among the rock and soil samples, XRD revealed similar mineral constituents among the rock and soil samples (Fig. 1). XRD revealed quartz (d = 4.25 and 3.34), feldspar (d = 3.76, 3.22, 2.98, and 2.90), and kaolinite (d = 7.15 and 3.55) as the possible main mineral constituents, while illite (d = 9.94 and 4.25) and montmorillonite (d = 15.29) were revealed as the possible minor mineral constituents in the rock and soil samples. Montmorillonite was only detected in the less altered rock samples (Fig. 1A), while kaolinite was only detected in the more altered rock and soil samples (Fig. 1B). In addition, some possible minor mineral constituents could not be identified.
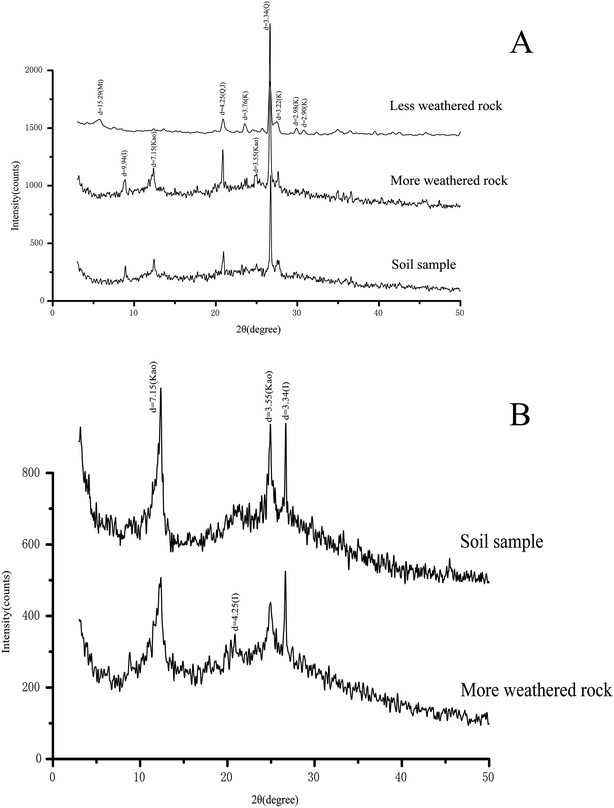 |
| Fig. 1 XRD patterns for the rock and soil samples ((A) <150 μm, (B) <2 μm). XRD revealed quartz (d = 4.25 and 3.34), feldspar (d = 3.76, 3.22, 2.98, and 2.90), and kaolinite (d = 7.15 and 3.55) as the possible main mineral constituents, while illite (d = 9.94 and 4.25) and montmorillonite (d = 15.29) were revealed as the possible minor mineral constituents in the rock and soil samples. Letter designations: Q, quartz; F, K-feldspar; K, kaolinite; I, illite; M, montmorillonite. | |
Bacterial counts and isolation of rock-weathering bacteria
In SSM (a moderate-nutrition medium), bacterial colonies were found to be the dominant populations. The culturable bacterial counts were significantly higher in the soil samples than in the altered rock samples (Table 1). The culturable bacterial counts in the soil samples were increased 1.8- and 111-fold compared with the more and less altered rocks, respectively. Furthermore, the culturable bacterial counts of the more altered rocks were increased 63-fold compared with the less altered rock samples (Table 1).
Using agar plates, we obtained 150 bacterial isolates. In the rock-weathering experiment, dissolved Fe, Si, and Al from tuff were used as an overall indicator of rock dissolution. Based on the rock dissolution experiment, all of the isolates from the altered rock and soil samples were found to have the capacity to solubilize tuff. After 7 days of incubation, the differences in the element (Si, Al, and Fe) releases by the isolates were observed. In the presence of the rock-weathering isolates from the less and more altered rock and soil samples, respectively, the Si release was increased 1.1- to 1.8-fold, 1.3- to 2.7-fold, and 1.2- to 4.0-fold, the Al release was increased 22- to 59-fold, 17- to 97-fold, and 3- to 195-fold, and the Fe release was increased 26- to 70-fold, 15- to 112-fold, and 17- to 129-fold, compared to the uninoculated controls, respectively.
Element release patterns of the isolates
Based on the potential of bacterial influence on Si, Al, and Fe releases from tuff during the rock dissolution experiment, the rock-weathering isolates could be grouped into three categories. The first group includes isolates with low potential for element solubilization (Si, Al, and Fe amounts were <300 μM, <20 μM, and <30 μM, respectively, in the culture medium); the second group includes isolates with moderate potential for element solubilization (Si, Al, and Fe amounts were 300–400 μM, 20–80 μM, and 30–40 μM, respectively); and the third group includes isolates with high potential for element solubilization (Si, Al, and Fe amounts were >400 μM, >80 μM, and >40 μM, respectively).
The proportion of the highly effective Si solubilizers was significantly different among the less and more altered rock and soil samples and the maximum proportion was observed in the soil samples (Table 2). The proportions of the highly effective Al and Fe solubilizers were significantly higher in the altered rocks than in the soils but were not significantly different between the less and more altered rocks. The proportions of the isolates with high potential for Al and Si or Fe solubilization simultaneously were significantly different among the less and more altered rock and the soil samples. No isolates with high potential for Si and Fe solubilization simultaneously were found in the less altered rock and the soil samples. Furthermore, the proportion of the isolates with high potential for Si, Al and Fe solubilization simultaneously was not significantly different between the more altered rocks and the soils, however no isolates with high potential for Si, Al, and Fe solubilization simultaneously were found in the less altered rocks (Table 2).
Table 2 Proportion of the high, moderate, and low effective element solubilizers isolated from the less and more altered rock and soil samples
|
Proportiona (%) |
Less altered rock |
More altered rock |
Soil sample |
If ± standard deviation is followed by the same letter within a line, then the values are not significantly different (P > 0.05) according to Tukey’s test. |
Highly effective element solubilizer |
Si |
6 ± 1c |
36 ± 4b |
76 ± 7a |
Al |
96 ± 8a |
88 ± 7a |
52 ± 5b |
Fe |
38 ± 5a |
30 ± 3a |
22 ± 3b |
Si + Al |
6 ± 1c |
12 ± 2b |
18 ± 2a |
Si + Fe |
0 |
10 ± 2a |
0 |
Al + Fe |
38 ± 5a |
10 ± 2b |
0 |
Si + Al + Fe |
0 |
20 ± 3a |
18 ± 1a |
![[thin space (1/6-em)]](https://www.rsc.org/images/entities/char_2009.gif) |
Moderately effective element solubilizer |
Si |
80 ± 6a |
56 ± 6b |
22 ± 3c |
Al |
4 ± 1c |
12 ± 2b |
32 ± 3a |
Fe |
32 ± 2a |
32 ± 3a |
18 ± 2b |
Si + Al |
2 ± 1b |
8 ± 2a |
8 ± 2a |
Si + Fe |
24 ± 2a |
20 ± 3a |
8 ± 2b |
Al + Fe |
0 |
0 |
4 ± 1a |
Si + Al + Fe |
0 |
2 ± 1a |
0 |
![[thin space (1/6-em)]](https://www.rsc.org/images/entities/char_2009.gif) |
Least effective element solubilizer |
Si |
14 ± 2a |
8 ± 1b |
2 ± 1c |
Al |
0 |
0 |
16 ± 2a |
Fe |
30 ± 2c |
38 ± 4b |
60 ± 5a |
Si + Al |
0 |
0 |
0 |
Si + Fe |
2 ± 1a |
4 ± 1a |
2 ± 1a |
Al + Fe |
0 |
0 |
16 ± 2a |
Si + Al + Fe |
0 |
0 |
0 |
The proportions of the moderately effective Si and Al solubilizers were significantly different among the altered rock and soil samples. The proportion of the moderately effective Fe solubilizers was significantly higher in the altered rocks than in the soils. The isolates with moderate potential for Al and Fe solubilization simultaneously and for Si, Al, and Fe solubilization simultaneously were observed in the soils and the more altered rocks, respectively (Table 2). Furthermore, the proportions of the least effective Si and Fe solubilizers were significantly different among the altered rock and soil samples. The isolates with low potential for Al solubilization and for Al and Fe solubilization simultaneously were only obtained in the soils. No isolates with low potential for Si and Al and for Si, Al, and Fe solubilization simultaneously were obtained in the altered rock and soil samples. The proportion of the isolates with low potential for Si and Fe solubilization simultaneously was not significantly different (Table 2).
Dynamic changes in element release of the isolates
The time courses of Fe, Si, and Al releases under tuff conditions are shown in Fig. 2. No significant changes in the water-soluble Fe, Si, and Al amounts in the medium were observed in the medium during the rock dissolution process in the absence of the rock-weathering isolates. Inoculation with the isolates L26, M23, and H41 was found to significantly increase water-soluble Fe, Si, and Al release from tuff compared to the uninoculated controls. No significant change in water-soluble Fe in the medium was observed before 4 days of incubation, however water-soluble Fe was significantly increased after 4 days in the presence of isolates L26 and M23. A peak in Fe release from tuff in the medium was obtained at 7 days followed by a significant decline in Fe release at 10 days and then a gradual increase in Fe release until the end of the experiment in the presence of isolate M23. No significant change in water-soluble Fe was obtained between 7 and 15 days in the presence of isolate L26. Water-soluble Fe was significantly increased after 1 day, reaching a maximum peak of Fe release at 7 days and then a significant decrease in Fe release until the end of the experiment in the presence of isolate H41 (Fig. 2).
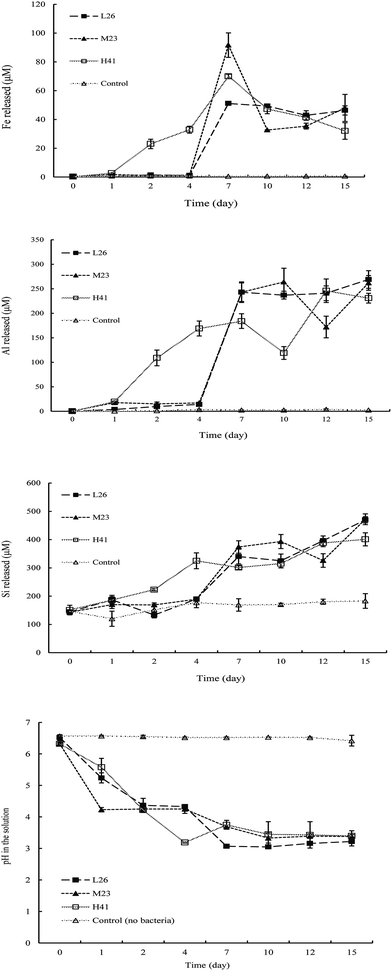 |
| Fig. 2 Influence of rock-weathering bacteria (L26, M23, and H41) on the releases of Fe, Si, and Al and on the pH of the medium added in tuff during 15 days of incubation. Error bars are ±standard error (n = 3). | |
In the presence of isolate L26, the amount of water-soluble Si in the medium was significantly increased after 1 day followed by a significant decline in Si release at 2 days and then a significant increase in Si release until the end of the experiment. No significant change in water-soluble Si in the medium was observed before 4 days, however water-soluble Si was significantly increased after 4 days followed by a significant decline in Si release at 12 days of incubation and then a significant increase in Si release until the end of the experiment in the presence of isolate M23. Water-soluble Si in the medium was significantly increased after 2 days followed by no significant change in Si release between 4 and 10 days and then a significant increase in Si release after 10 days in the presence of isolate H41. Water-soluble Al in the medium was significantly increased after 4 days followed by no significant change in Al release between 7 and 15 days in the presence of isolate L26. Water-soluble Al was also significantly increased after 4 days followed by a significant decline in Al release at 12 days and then a significant increase in Al release after 12 days in the presence of isolate M23. Water-soluble Al was significantly increased after 1 day followed by a significant decline in Al release at 10 days and then a significant increase in Al release after 10 days in the presence of isolate H41.
In addition, during 1–15 days of incubation, there was a significant change in the pH values of the medium inoculated with the isolates during the culture time. No significant change in the pH values of the medium was observed in the uninoculated control. In the presence of the isolates, the pH of the medium significantly decreased after 1 day and the isolates lowered the medium pH to < 4 after 7 days of incubation.
Acid-producing ability of the isolates during the rock-weathering process
The rock-weathering isolates exhibited different abilities to acidify the culture medium in the rock dissolution process. The proportions of the highly (pH < 4.00 in the medium) effective acid-producing isolates accounted for 96%, 74%, and 50% in the less and more altered rocks and the soils, respectively, while 4%, 26%, and 50% of the isolates had a moderate and low (pH ≥ 4.00 in the medium) effective acid-producing ability in the less and more altered rocks and the soils, respectively. More isolates from the altered rocks had high potential to acidify the culture medium during the rock dissolution process.
Physiological characteristics of the isolates
The rock-weathering isolates from the less and more altered rock samples and soil samples could grow well in the range of salinity 1–5%, at temperatures of 28–45 °C, and at pH 4–8.5, respectively (Fig. 3). The optimal conditions for the growth of the rock-weathering isolates were 1.0% of NaCl, 28 °C, and pH 7.0. The proportions of the rock-weathering isolates which could grow at 10–15% of NaCl, 4 °C or 55 °C, and pH 4 or 10 were significantly higher in the rock samples than in the soil samples (Fig. 3). No rock-weathering isolates from the soil samples could grow at 10–15% of NaCl and pH 10.0. Furthermore, the proportions of the rock-weathering isolates which could grow at 10–15% of NaCl, 4 °C or 55 °C, and pH 10 were significantly higher in the more altered rock samples than in the less altered rock samples (Fig. 3). After incubation at 28 °C for 48 h, orange halos formed around the colonies of the isolates on blue agar, indicating that all the rock-weathering isolates from the less and more altered rock and soil samples could produce siderophores.
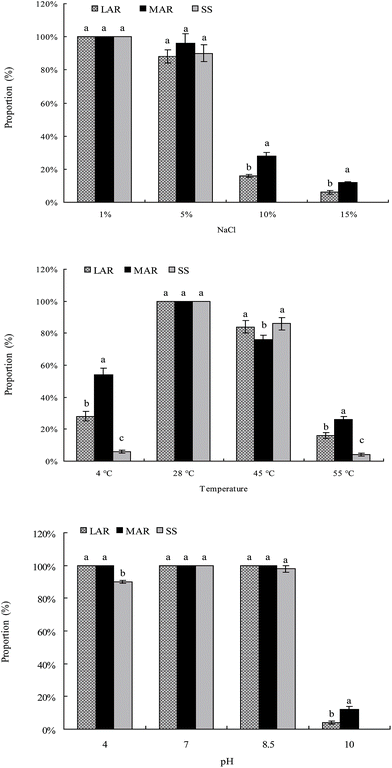 |
| Fig. 3 Influence of salt, temperature, and pH on the growth of the mineral-weathering bacteria isolated from the less and more altered rock samples and the soil samples. Error bars are ±standard error (n = 3). Bars indicated by the same letter are not significantly different (P > 0.05) according to Tukey’s test. | |
Phylogenetic analysis of the isolates
Sequencing of the 16S rRNA and phylogenetic analysis showed that the isolates were affiliated with 22 bacterial genera (6, 13, and 10 genera were obtained from the less and more altered rock and soil samples, respectively) (Table 3). The most frequently isolated rock-weathering bacteria from the less altered rocks belonged to Bacillus (88%); Bacillus (34%), Enterobacter (18%) and Acinetobacter (12%) were found to be the most frequently isolated rock-weathering bacteria in the more altered rocks. Meanwhile Arthrobacter (38%) and Bacillus (34%) were the most frequently isolated rock-weathering bacteria in the soils. Bacillus and Acinetobacter species were common in the altered rocks and the soils (Table 3). At the genus level, Brevibacillus and Proteus were specific to the less altered rocks, while Enterobacter, Alcaligenes, Kocuria, Microbacterium, Moraxella, Staphylococcus, Sphingomonas, Stenotrophomonas, and Cupriavidus were only observed in the more altered rocks. Arthrobacter, Burkholderia, Pseudomonas, Phyllobacterium, Agrobacterium, and Psychrobacter were only found in the soils (Table 3). Notably, an enrichment of Bacillus species (88%) was observed in the less altered rock samples, while Arthrobacter species (38%) were enriched in the soil samples.
Table 3 Proportions of the rock-weathering bacterial genera from the altered rocks and soils
Rank |
Rock-weathering bacterial genera (proportion [%]) from the altered rocks and soilsa |
Less altered rock |
More altered rock |
Soil sample |
Bacterial genera in boldface were specific to the respective altered rock and soil samples. |
1 |
Bacillus (88) |
Bacillus (34) |
Arthrobacter (38) |
2 |
Lysinibacillus (4) |
Enterobacter (18) |
Bacillus (34) |
3 |
Acinetobacter (2) |
Acinetobacter (12) |
Acinetobacter (6) |
4 |
Brevibacillus (2) |
Staphylococcus (8) |
Agrobacterium (4) |
5 |
Paenibacillus (2) |
Kocuria (6) |
Burkholderia (4) |
6 |
Proteus (2) |
Alcaligenes (4) |
Paenibacillus (4) |
7 |
|
Lysinibacillus (4) |
Psychrobacter (4) |
8 |
|
Sphingomonas (4) |
Brevibacterium (2) |
9 |
|
Brevibacterium (2) |
Phyllobacterium (2) |
10 |
|
Cupriavidus (2) |
Pseudomonas (2) |
11 |
|
Microbacterium (2) |
|
12 |
|
Moraxella (2) |
|
13 |
|
Stenotrophomonas (2) |
|
Community distribution of the element solubilizers
The highly effective Si solubilizers were affiliated with 3, 15, and 21 bacterial species in the less and more altered rocks and soils, respectively (Fig. 4), among which 53 and 71% of the species were specific to the more altered rocks and the soils, respectively. The moderately effective Si solubilizers belonged to 10, 16, and 8 species in the less and more altered rocks and the soils, respectively (Fig. 4), among which 40, 63 and 100% of the species were specific to the less and more altered rocks and the soils, respectively. The least effective Si solubilizers were affiliated with 6, 3, and 1 species in the less and more altered rocks and the soils, respectively (Fig. 4), among which 33, 100, and 100% of the species were specific to the respective rock and soil samples.
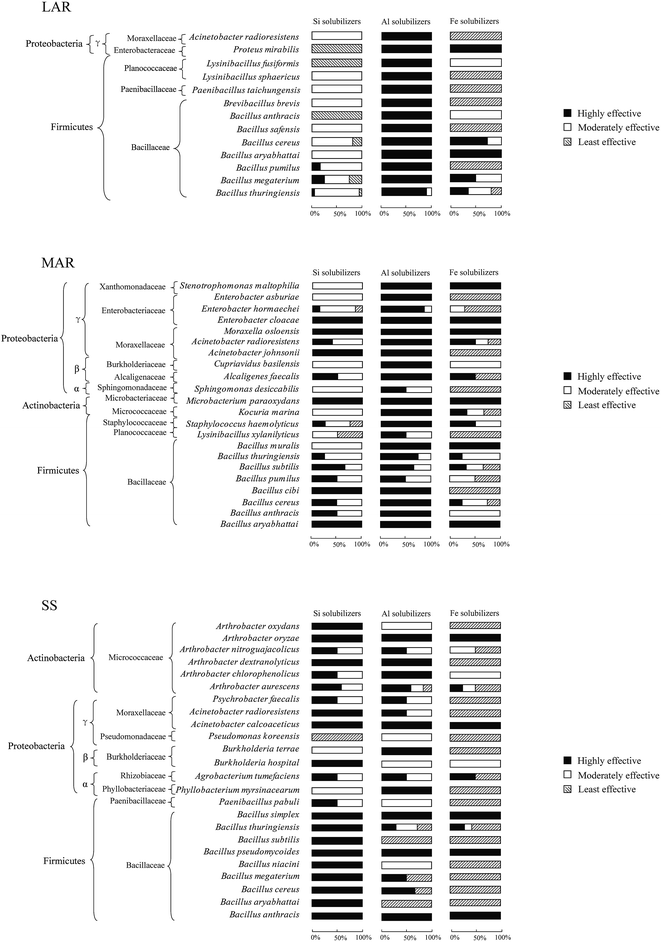 |
| Fig. 4 Proportional abundance of the high, moderate, and low effective element (Si, Al, and Fe) solubilizers from the less (LR) and more (MR) altered rock and soil samples (SS), respectively. | |
The highly effective Al solubilizers belonged to 13, 22, and 17 species in the less and more altered rocks and the soils, respectively (Fig. 4), among which 46, 68, and 71% of the species were specific to the less and more altered rocks and the soils, respectively. The moderately effective Al solubilizers were affiliated with 1, 6, and 11 species in the less and more altered rocks and the soils, respectively (Fig. 4), among which 50 and 82% of the species were specific to the more altered rocks and the soils respectively. The least effective Al solubilizers were affiliated with 6 species in the soils (Fig. 4), however no least effective Al solubilizers were found in the less or more altered rocks. The highly effective Fe solubilizers were affiliated with 5, 13, and 8 species in the less and more altered rocks and the soils, respectively (Fig. 4), among which 20, 62, and 75% of the species were specific to the less and more altered rocks and the soils, respectively. The moderately effective Fe solubilizers were affiliated with 5, 10, and 5 species in the less and more altered rocks and the soils, respectively (Fig. 4), among which 20, 40, and 80% of the species were specific to the less and more altered rocks and the soils, respectively. The least effective Fe solubilizers were affiliated with 7, 12, and 17 species in the less and more altered rocks and the soils, respectively (Fig. 4), among which 57, 67, and 65% of the species were specific to the less and more altered rocks and the soils, respectively.
Discussion
Although little is known about the weathering communities on rock surfaces, in light of the environments on the surfaces of tuffs and in the adjacent soils (Table 1), it can be assumed that rock-adapted isolates are found on rock surfaces and in the soils. The knowledge of the interaction between the rocks and the bacteria is of importance to the ultimate understanding of the bio-weathering process of rocks. In this study, 104–106 bacterial counts per gram of rock or soil were obtained on altered rock surfaces and in the adjacent soils, suggesting that the bacteria present on the rock surfaces and in the soils have a range of physiological properties clearly related to their environments. Our studies showed that the bacteria from the altered rocks had a stronger adaptation to the extreme conditions (high or low temperature, low or high pH, and salt stress) than the bacteria from the soils (Fig. 3).
Bacteria can accelerate rock and mineral weathering.34,35 The rock dissolution experiment also showed that all isolated bacteria have the ability to release elements from tuff under a low nutrient medium. Furthermore, the rock-weathering ability of the bacteria was different between the altered rocks and the soils and between the less and more altered rocks (Table 2). Several studies have also demonstrated that the effectiveness of culturable mineral-weathering bacteria varies in relation to the ecological niche they occupy.14,36 Furthermore, changes in the concentrations of Fe, Si, and Al released from tuff fluctuated with time during the weathering experiment in the selected three bacteria (Fig. 2). The possible explanation for the fluctuation in the concentrations of Fe, Si, and Al from tuff by the tested bacteria may represent re-precipitation and re-dissolution of secondary minerals.37,38 In addition, the rock dissolution was non-stoichiometric with respect to Fe, Si, and Al release in our study. Despite the difficulties associated with attempting to interpret complex processes occurring in the natural environment based on simple laboratory experiments, it is possible to glean information about the basic principles of bacterially mediated rock-weathering from experimental work. Microbiologic processes may play important roles in weathering despite the cold temperatures and oligotrophic conditions, as could be seen on the natural rock surfaces.39 In fact, rock-weathering bacteria have been frequently isolated from low organic and oligotrophic environments.11,36 Moreover, Höppener-Ogawa et al.40 also demonstrated that the abundance of the mineral weathering collimonads was significantly higher in the mineral than in the organic layer of forest soils. This result suggests that the rock or mineral weathering ability may be a functional and ecological trait of the rock-weathering bacteria. Also, rocks are important in ecosystems as they are the primary pools of inorganic nutrients such as K, Ca, Mg, Fe and P, as well as essential trace elements. Heterotrophs can utilize organic compounds as carbon sources and their energy is provided through the oxidation-reduction reactions of these organic compounds. So, we speculate that the differences in the available inorganic nutrients and organic matter result in the different growth and metabolism of the bacteria inhabited on the surfaces of the altered rocks as well as in the soils. Consequently, different rock weathering abilities of the bacteria are observed among the less and more altered rocks and the soils (Table 2). For instance, the ability of each element release from tuff was dependent on the bacteria and their origin (Table 2). Most of the isolates displayed different abilities in releasing each element from tuff among the altered rocks and the soils (Table 2). Furthermore, the proportions of the highly or moderately or least effective element solubilizers were different between the altered rocks and the soils and between the less and more altered rocks. The above discussion suggests the diversity and weathering-related change in the element releasing patterns of the culturable rock-weathering bacteria. Differences in the available Si, Al, and Fe amounts may suggest differences in the element releasing patterns of the bacteria between the less and more altered rocks (Table 1). Similarly, Lapanje et al.41 also demonstrated that the patterns of elemental release during the granite dissolution can be changed by different aerobic heterotrophic bacteria isolated from deglaciated granite sand.
Bacteria can weather rock or minerals by proton- and/or ligand-promoted mineral dissolution under aerobic conditions.1,17,42,43 The proton- and/or ligand-promoted rock dissolution mechanisms are linked to the geochemically reactive organic acids, protons, and siderophores produced by bacteria.44 The decrease in pH of solutions in the weathering experiments reported here may therefore be presumed to be related to a mixture of acid and siderophore molecules in the presence of bacteria. The obtained rock-weathering bacteria varied in their ability to produce acids. For the altered rocks, 74–96% of bacteria produced large pH changes (pH < 4.0 in the solutions), suggesting that most bacteria from the altered rocks could weather tuff by proton-promoted dissolution mechanisms. In the soils, 50% of bacteria produced large pH changes and another 50% of bacteria produced small pH changes (pH ≥ 4.0), suggesting that half of the bacteria from the soils could weather tuff by proton-promoted or by ligand-promoted dissolution mechanisms. Furthermore, similar siderophore production by the rock-weathering bacteria from the altered rocks and the soils was observed, suggesting that chelation by siderophores may not be the dominant mechanism causing enhanced element release from tuff.
Gleeson et al.45 revealed a diversity of bacterial structures on surfaces of all variably weathered minerals and whole-rock granite. Furthermore, Uroz et al.17 revealed a broad diversity of the mineral-weathering bacteria within α-, β-, γ-Proteobacteria, Firmicutes, and Actinobacteria lineages. Our studies also showed that diverse and distinct rock-weathering bacterial populations existed on the surfaces of the altered rocks as well as in the adjacent soils (Fig. 4). Furthermore, most of the rock-weathering species from the altered rocks and the soils have not been previously reported (Fig. 4), suggesting that these weathering bacteria were specific to their environments. Also, more than half of the rock-weathering bacteria from the altered rocks were different to those from the adjacent soils. Certini et al.46 showed that rock surfaces seem to be colonized by specific bacteria that are different to those inhabiting the surrounding soils. Notably, the most efficient mineral-weathering Burkholderia and Collimonas genera frequently found in acidic and nutrient-poor soils17 were not detected in the altered rocks. The above results indicate significant differences in the rock-weathering bacterial communities between different environments. In this study, the highly effective element solubilizers included not only the major population structure but also the minor bacterial groups (Fig. 4). In addition, some identical bacterial species were observed in the least, moderately, and highly effective rock-weathering bacteria, while some bacterial species were specific to the least or to the moderately or to the highly effective rock-weathering bacteria (Fig. 4). These findings not only revealed the functional and metabolic diversity of the rock-weathering bacterial communities but also important implications for the understanding of weathering processes, as alteration-related rock-weathering may be attributed to the different indigenous weathering bacterial communities.
Although Bacillus and Acinetobacter species displayed an even distribution among the less and more altered rocks and the soils (Table 3), bacterial species of the weathering communities showed different weathering-specific distributions among the altered rocks and the soils (Fig. 4). Specific rock-weathering bacterial species existed in the altered rocks and the soils and more specific rock-weathering species were obtained in the soils and the altered rocks than in the less altered rocks. Even among the common Bacillus and Acinetobacter species in the altered rocks and the soils, weathering-specific distributions were found (Fig. 4). Furthermore, the Bacillus and Acinetobacter species were enriched in the less and more altered rocks, respectively (Table 3). Gleeson et al.45 showed that mineral chemistry affected individual bacterial ribotypes and that Al, Si, and Ca had a significant impact on the bacterial community structure within the system. The available K, Ca, Mg, and organic matter amounts were significantly different among the less and more altered rocks and the soils (Table 1), suggesting that the change in the rock-weathering bacterial populations between the altered rocks and the soils may be shaped by these distinct edaphic factors. Also, the pH and the available Si, Al, Fe, and P amounts were significantly different between the less and more altered rocks, suggesting that the change in the rock-weathering bacterial populations between the less and more altered rocks may also be affected by these distinct factors. Furthermore, the difference in the secondary minerals may also be responsible for the distinct rock-weathering bacterial populations between the less and more altered rocks (Fig. 1). Altogether, these results suggest that differential impacts of weathering degree on the culturable rock-weathering bacterial populations could be linked to the nutrient availability rate on the rock surfaces and in the soils.
Conflicts of interest
There are no conflicts to declare.
Acknowledgements
This work was supported by the Natural Science Research Key Project of Education Office of Anhui Province (KJ2016A478) and the Research Fund of Bengbu Medical College (BYKY1611ZD).
References
- B. Agra, J. Ygor, N. Araujo, W. Clistenes and M. Biondi Caroline, Geoderma, 2016, 284, 1–22 CrossRef.
- W. Von Bloh, S. Franck, C. Bounama and H.-J. Schellnhuber, Geomicrobiology, 2003, 20, 501–511 CrossRef.
- C. S. Cockell, N. Kennerley, M. Lindstrom, J. Watson, V. Ragnarsdottir, E. Sturkell, S. Ott and A. G. Tindle, Geomicrobiology, 2007, 24, 425–440 CrossRef CAS.
- P. Van Thienen, K. Benzerara, D. Breuer, C. Gillmann, S. Labrosse, P. Lognonné and T. Spohn, Space Sci. Rev., 2007, 129, 167–203 CrossRef CAS.
- Z. Balogh-Brunstad, C. K. Keller, J. T. Dickinson, F. Stevens, C. Y. Li and B. T. Bormann, Geochim. Cosmochim. Acta, 2008, 72, 2601–2618 CrossRef CAS.
- C. Balland, A. Poszwa, C. Leyval and C. Mustin, Geochim. Cosmochim. Acta, 2010, 74, 5478–5493 CrossRef CAS.
- A. Esposito, S. Ciccazzo, L. Borruso, S. Zerbe, D. Daffonchio and L. Brusetti, Curr. Microbiol., 2013, 67, 472–479 CrossRef CAS PubMed.
- Q. Wang, R. R. Wang, L. Y. He, J. J. Lu, Z. Huang and X. F. Sheng, Biol. Fertil. Soils, 2014, 50(7), 1025–1034 CrossRef.
- K. Benzerara, M. Barakat, N. Menguy, F. Guyot, G. De Luca, C. Audrain and T. Heulin, Geomicrobiology, 2004, 21, 341–349 CrossRef CAS.
- C. J. Daughney, J.-P. Rioux, D. Fortin and T. Pichler, Geomicrobiology, 2004, 21, 21–31 CrossRef CAS.
- F. Zhao, G. Qiu, Z. Huang, L. Y. He and X. F. Sheng, Geomicrobiology, 2013, 30, 616–622 CrossRef CAS.
- D. P. Oliveira, L. R. Sartor, V. S. Souza, M. M. Correa, R. E. Romero and G. R. P. Andrade, et al., Catena, 2018, 162, 325–332 CrossRef CAS.
- H. Abdulla, Microb. Ecol., 2009, 58, 753–761 CrossRef CAS PubMed.
- C. Calvaruso, M. P. Turpault, E. Leclerc, J. Ranger, J. Garbaye, S. Uroz and P. Frey-Klett, Appl. Environ. Microbiol., 2010, 76, 4780–4787 CrossRef CAS PubMed.
- C. Collignon, S. Uroz, M.-P. Turpault and P. Frey-Klett, Soil Biol. Biochem., 2011, 43, 2012–2022 CrossRef CAS.
- M. E. Puente, C. Y. Li and Y. Bashan, Environ. Exp. Bot., 2009, 66, 389–401 CrossRef CAS.
- S. Uroz, P. Oger, C. Lepleux, C. Collignon, P. Frey-Klett and M. P. Turpault, Res. Microbiol., 2011, 162, 820–831 CrossRef CAS PubMed.
- S. Uroz, M. P. Turpault, L. Van Scholl, B. Palin and P. Frey-Klett, Soil Biol. Biochem., 2011, 43, 2275–2282 CrossRef CAS.
- C. Lepleux, M. P. Turpault, P. Oger, P. Frey-Klett and S. Uroz, Appl. Environ. Microbiol., 2012, 78, 7114–7119 CrossRef CAS PubMed.
- R. Matlakowska and A. Skłodowska, Appl. Microbiol., 2009, 107, 858–866 CrossRef CAS PubMed.
- K. Olsson-Francis, A. E. Simpson, D. Wolff-Boenisch and C. S. Cockell, Geobiology, 2012, 10, 434–444 CrossRef CAS PubMed.
- X. F. Sheng, F. Zhao, L. Y. He, G. Qiu and L. Chen, Can. J. Microbiol., 2008, 54, 1064–1068 CrossRef CAS PubMed.
- R. Matlakowska, A. Skłodowska and K. Nejbert, FEMS Microbiol. Ecol., 2012, 81, 99–110 CrossRef CAS PubMed.
- Z. L. Song, H. Y. Liu, C. A. E. Stromberg, H. L. Wang, P. J. Strong and X. M. Yang, et al., Sci. Total Environ., 2018, 615, 1–8 CrossRef CAS PubMed.
- D. Gleeson, F. McDermott and N. Clipson, Adv. Appl. Microbiol., 2007, 62, 81–104 CAS.
- J. C. Yeomans and J. M. Bremmer, Commun. Soil Sci. Plant Anal., 1998, 19, 1467–1476 CrossRef.
- A. Mehlich, Commun. Soil Sci. Plant Anal., 1984, 15, 1409–1416 CrossRef CAS.
- P. R. Day, Methods of Soil Analysis, 1965, pp. 545–567 Search PubMed.
- X. D. Wang, N. Yamaguchi, T. Someya and M. Nasu, J. Microbiol. Methods, 2007, 71, 1–6 CrossRef CAS PubMed.
- B. Schwyn and J. B. Neilands, Anal. Biochem., 1987, 160, 47–56 CrossRef CAS PubMed.
- J. Sambrook and D. Russell, Molecular Cloning, a Laboratory Manual, Cold Spring Harbor Laboratory Press, NY, 2001 Search PubMed.
- D. J. Lane, Nucleic acid techniques in bacterial systematics, 1991, pp. 115–175 Search PubMed.
- Q. Wang, G. M. Garrity, J. M. Tiedje and J. R. Cole, Appl. Environ. Microbiol., 2007, 73, 5261–5267 CrossRef CAS PubMed.
- B. Frey, S. R. Rieder, I. Brunner, M. Plötze, S. Koetzsch, A. Lapanje, H. Brandl and G. Furrer, Appl. Environ. Microbiol., 2010, 76, 4788–4796 CrossRef CAS PubMed.
- I. Štyriaková, I. Štyriak and H. Oberhänsli, Mineral. Petrol., 2012, 105, 135–144 CrossRef.
- S. Uroz, C. Calvaruso, M. P. Turpault, J. C. Pierrat, C. Mustin and P. Frey-Klett, Appl. Environ. Microbiol., 2007, 73, 3019–3027 CrossRef CAS PubMed.
- P. Delvasto, A. Valverde, A. Ballester, J. M. Igual, J. A. Muñoz, F. González, M. L. Blázquez and C. García, Soil Biol. Biochem., 2006, 38, 2645–2654 CrossRef CAS.
- M. Dopson, L. Lovgren and D. Bostrom, Hydrometallurgy, 2009, 96, 288–293 CrossRef CAS.
- A. Seward and A. Prieto, Renewable Energy, 2018, 118, 546–554 CrossRef.
- S. Höppener-Ogawa, J. H. J. Leveau, W. Smant, J. A. van Veen and W. de Boer, Appl. Environ. Microbiol., 2007, 73, 4191–4197 CrossRef PubMed.
- A. Lapanje, C. Wimmersberger, G. Furrer, I. Brunner and B. Frey, Microb. Ecol., 2012, 63, 865–882 CrossRef CAS PubMed.
- H. L. Buss, A. Lüttge and S. L. Brantley, Chem. Geol., 2007, 240, 326–342 CrossRef CAS.
- A. Neaman, J. Chorover and S. L. Brantley, Am. J. Sci., 2006, 306, 451–473 CrossRef CAS.
- S. Uroz, C. Calvaruso, M. P. Turpault, A. Sarniguet, W. de Boer, J. H. J. Leveau and P. Frey-Klett, Soil Biol. Biochem., 2009, 41, 2178–2186 CrossRef CAS.
- D. B. Gleeson, N. M. Kennedy, N. Clipson, K. Melville, G. M. Gadd and F. P. McDermott, Microb. Ecol., 2006, 51, 526–534 CrossRef PubMed.
- G. Certini, C. D. Campbell and A. C. Edwards, Soil Biol. Biochem., 2004, 36, 1119–1128 CrossRef CAS.
|
This journal is © The Royal Society of Chemistry 2018 |
Click here to see how this site uses Cookies. View our privacy policy here.