DOI:
10.1039/C8RA00280K
(Paper)
RSC Adv., 2018,
8, 17677-17684
Deactivation by HCl of CeO2–MoO3/TiO2 catalyst for selective catalytic reduction of NO with NH3
Received
10th January 2018
, Accepted 23rd April 2018
First published on 15th May 2018
Abstract
The effect of HCl on a CeO2–MoO3/TiO2 catalyst for the selective catalytic reduction of NO with NH3 was investigated with BET, XRD, NH3-TPD, H2-TPR, XPS and catalytic activity measurements. The results showed that HCl had an inhibiting effect on the activity of the CeO2–MoO3/TiO2 catalyst. The deactivation by HCl of the CeO2–MoO3/TiO2 catalyst could be attributed to pore blockage, weakened interaction among ceria, molybdenum and titania, reduction in surface acidity and degradation of redox ability. The Ce3+/Ce4+ redox cycle was damaged because unreactive Ce3+ in the form of CeCl3 lost the ability to be converted to active Ce4+ in the SCR reaction. In addition, a decrease in the amount of chemisorbed oxygen and the concentrations of surface Ce and Mo was also responsible for the deactivation by HCl of the CeO2–MoO3/TiO2 catalyst.
1. Introduction
The selective catalytic reduction (SCR) of NOx with NH3 is an efficient technology for the removal of NOx in flue gas from stationary sources.1 V2O5–WO3(MoO3)/TiO2 catalysts have been widely used in the last few decades.2,3 In recent years, great attention has been paid to develop environment-friendly vanadium-free catalysts for SCR applications mainly owing to the toxicity of vanadium.4,5 Cerium-based catalysts are regarded as promising candidates due to the high oxygen storage capacity and excellent redox properties of CeO2.4,6,7 Many researchers have developed numerous cerium-based metal oxide catalysts, which possessed good SCR activity, such as MoO3/CeO2–ZrO2,8 Cu–Ce–Ti oxide,6,9 Mn–Ce–Ti oxide,10 etc. Our group prepared a CeO2–MoO3/TiO2 catalyst using a single step sol–gel method, which exhibited high SCR activity and resistance to 10% H2O and 1000 ppm SO2.11 However, from the point of view of industrial applications, further studies are still required to clarify their adaptability to other components in flue gas from stationary sources, such as alkali (earth) metals, heavy metals, HCl, etc.
It is well known that HCl is widely present in the flue gas from coal-fired boilers and municipal solid waste (MSW) incinerators. The effect of HCl on SCR catalyst has been investigated by several researchers. Chen et al.12 attributed the deactivation by HCl of V2O5/TiO2 catalyst to the formation of volatile vanadium chlorides and NH4Cl. Lisi et al.13 reported that HCl suppressed the SCR activity of commercial V2O5–WO3/TiO2 catalyst due to the formation of new acid sites showing a lower activity compared to the original one. Yang et al.14 proposed that Cl could inhibit the adsorption of NH3 and NOx species, thereby leading to the deactivation of Ce/TiO2. On the contrary, Hou et al.15 found that HCl had a positive effect on the catalytic activity of V2O5/AC catalyst at 150 °C. As for the effect of HCl on CeO2–MoO3/TiO2 catalyst for the SCR of NO with NH3, there were few reports to our knowledge till now.
In this work, the effect of HCl on CeO2–MoO3/TiO2 catalyst was investigated for the SCR of NO with NH3. A series of characterizations, including BET, XRD, XPS, NH3-TPD and H2-TPR, were performed to provide an insight into the substantial change in CeO2–MoO3/TiO2 catalyst caused by HCl.
2. Experimental
2.1. Catalyst preparation
The CeO2–MoO3/TiO2 catalyst was prepared by a single step sol–gel method as reported in our previous study.11 The mass ratio of CeO2
:
MoO3
:
TiO2 was 20
:
10
:
100. All chemicals used in the catalyst preparation were purchased from Sinopharm Chemical Reagent Corp. (Shanghai, China). Except that titanium butoxide (TBOT) was chemically pure, the other reagents were of analytical grade. TBOT, anhydrous ethanol, deionized water, 65–68 wt% nitric acid, cerium nitrate hexahydrate (Ce(NO3)3·6H2O) and ammonium molybdate tetrahydrate ((NH4)6Mo7O24·4H2O) were mixed at a molar ratio of 1
:
35
:
19
:
2
:
0.1
:
0.1. After continuously stirred for 3 h at room temperature, the solution was kept at 80 °C for 24 h to form xerogel. The obtained xerogel were milled and sieved out, followed by calcination at 500 °C for 5 h in static air. The catalyst was denoted as CMT.
The HCl-loaded CeO2–MoO3/TiO2 catalysts were prepared by impregnation via incipient wetness with appropriate hydrochloric acid solution on CMT. The mixture was aged for 24 h and dried at 80 °C for 12 h. The HCl-loaded CeO2–MoO3/TiO2 catalysts were denoted as CMTx, where x represented the molar ratio of Cl and Ce.
2.2. Catalyst characterization
BET surface area, total pore volume and average pore diameter were measured by N2 adsorption and desorption at −196 °C with ASAP2020-M (Micromeritics Instrument Corp.). Prior to the measurement, the sample was degassed in vacuum at 300 °C for 4 h.
X-ray diffraction (XRD) measurement was carried out with on a X'Pert PRO diffractometer (Panalytical Corp.) with Cu Kα radiation at 40 kV and 40 mA.
X-ray photoelectron spectroscopy (XPS) data were obtained with a Thermo ESCALAB 250 spectrometer using monochromated Al Kα X-rays (hm = 1486.6 eV) as a radiation source at 150 W. Sample charging effects were eliminated by correcting the observed spectra with the C 1s binding energy (BE) value of 284.6 eV.
Temperature programmed desorption of NH3 (NH3-TPD) and temperature programmed reduction of H2 (H2-TPR) were performed on a FINESORB-3010 chemisorption analyzer (FINETEC Instruments Corp.) with 0.1 g of the catalysts with a thermal conductivity detector (TCD). For NH3-TPD, the sample was pretreated at 500 °C in He for 1 h. After cooled down, it was exposed in a 0.5% NH3/He (30 mL min−1) gas flow for 1 h, followed by flushing with He for 1 h. Finally, the sample was heated up to 700 °C with the rate of 10 °C min−1 in He. H2-TPR were carried out in the flow of H2 (10%) in Ar (30 mL min−1) from room temperature to 800 °C with the heating rate of 10 °C min−1.
2.3. SCR activity test
The activity measurements were carried out in a fixed-bed quartz reactor (i.d. = 8 mm) using 0.23 g catalyst with 60–100 mesh at atmospheric pressure in the temperature range of 150–500 °C. The feed gas contained 1000 ppm NO, 1000 ppm NH3, 3 vol% O2, 10% H2O (when used), 500 ppm SO2 (when used) and N2 as balance gas. The total flow rate was 500 mL min−1, corresponding to a gas hourly space velocity (GHSV) of 150
000 h−1. The concentrations of NO, SO2 and O2 were continuously monitored by a gas analyzer (350 Pro, Testo). The concentrations of NO2 and N2O were recorded by a FT-IR gas analyzer (DX-4000, Gasmet). The NO conversion and N2 selectivity of catalysts were calculated by:16 |
 | (1) |
|
 | (2) |
3. Results and discussion
3.1. NH3-SCR performance
Fig. 1 shows the NH3-SCR performance of the fresh and HCl-loaded CMT. It could be seen from Fig. 1(a) that the catalytic activity of CMT declined and its active temperature window was shortened with increasing HCl loadings in the temperature range of 150–500 °C. Furthermore, it seemed that the inhibiting effect of HCl on the SCR activity of CMT was more obvious below 300 °C. As for the N2 selectivity, there was hardly a difference among the three samples at temperatures from 250 °C to 350 °C. The N2 selectivity of CMT10 decreased slightly below 250 °C and at 350–450 °C in comparison with those of CMT and CMT5. It could be seen that HCl loadings had little influence on the N2 selectivity of CMT.
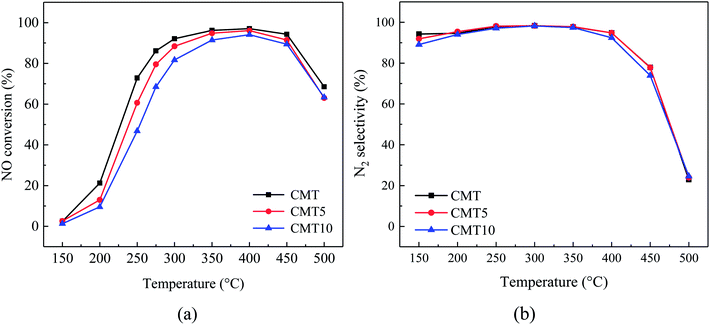 |
| Fig. 1 SCR activity (a) and N2 selectivity (b) of different samples as a function of reaction temperature. Reaction conditions: [NO] = [NH3] = 1000 ppm, [O2] = 3%, balance N2, GHSV = 150 000 h−1. | |
The effect of H2O on the SCR activities of the fresh and HCl-loaded CMT was investigated and the results are shown in Fig. 2. Compared with Fig. 1 and 2, it was found that CMT10 suffered much more serious deactivation than the other samples. The presence of H2O suppressed the NO removal process over SCR catalysts by competing with NH3 adsorption on the reaction sites or occupying the oxygen vacancies in active species.6,17,18 The existence of HCl on the surface of CMT was likely to aggravate the competitive adsorption of H2O with NH3 and the positioning of the oxygen vacancies in Ce species.
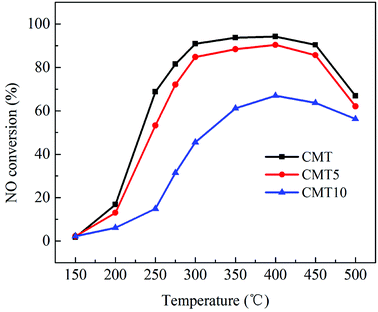 |
| Fig. 2 Effect of H2O on NO conversion over different samples. Reaction conditions: [NO] = [NH3] = 1000 ppm, [O2] = 3%, [H2O] = 10%, balance N2 and GHSV = 150 000 h−1. | |
Fig. 3 displays the co-effect of H2O and SO2 on the SCR of NO with NH3 over the fresh and HCl-loaded CMT. The presence of SO2 and H2O led to a rapid decrease in the SCR activities of the three samples to different extents. After about 4 hours, their activities became stable. It was clear that the resistance of CMT against SO2 and H2O declined with increasing the loadings of HCl. The deactivation by SO2 of Ce-based oxide SCR catalysts was believed to result from the formation of sulfate species on their surface, including NH4HSO4, Ce(SO4)2 and Ce2(SO4)3.17,19,20 In the presence of O2, SO2 and H2O might react with NH3 to produce NH4HSO4. NH4HSO4 could accumulate on catalyst surface and further cover active sites.19 On the other hand, SO2 might combine with Ce species on catalyst surface in the presence of O2 to form high thermally stable Ce(SO4)2 and Ce2(SO4)3. They could hinder the Ce4+/Ce3+ redox cycle and inhibit the formation and adsorption of surface nitrate species.19 After SO2 and H2O were cut off from the feed gas, the NO conversions over CMT and CMT5 increased slightly, while no obvious change was observed in the NO conversion over CMT10. It indicated that there might exist NH4HSO4 on the surface of CMT and CMT5. After removing SO2 and H2O, unstable NH4HSO4 volatilized or decomposed. The deactivation of the three samples was largely caused by Ce(SO4)2 and Ce2(SO4)3. The presence of HCl might promote the formation of the two sulfate species, thereby resulting in more serious deactivation of CMT10.
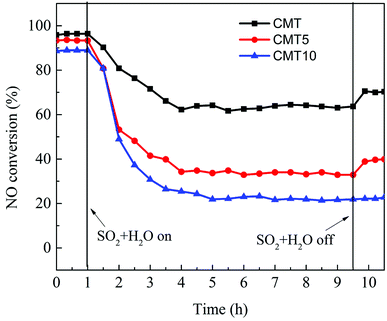 |
| Fig. 3 Effect of H2O and SO2 on NO conversion for different catalyst samples as a function of reaction temperature. Reaction conditions: [NO] = [NH3] = 1000 ppm, [O2] = 3%, [H2O] = 10%, [SO2] = 500 ppm, balance N2 and GHSV = 150 000 h−1. | |
3.2. Characterization of catalysts
3.2.1 BET and XRD analysis. According to the BET results, it was found that the BET surface area of different samples decreased by the following order: CMT (99.06 m2 g−1) > CMT5 (88.76 m2 g−1) > CMT10 (81.49 m2 g−1). It was clear that the BET surface area of CMT decreased with increasing Cl loadings, which was consistent with the catalytic activity (as shown in Fig. 1). Fig. 4 shows the pore size distribution of the samples. It could be seen that the pore sizes of CMT increased with increasing Cl loadings while the pore volumes fell gradually. This meant that a portion of small mesopores might be blocked by chloride species. Wang et al.21 found the similar phenomena in the study on the effect of F or Cl on Mn/TiO2 catalyst.
 |
| Fig. 4 Pore size distributions of the catalyst samples. | |
The XRD patterns of the fresh and HCl-loaded CMT are presented in Fig. 5. Only the diffraction peaks ascribed to anatase TiO2 were detected, while the characteristic peaks of CeO2 and MoO3 were not observed. This demonstrated that the presence of HCl had no obvious impact on the dispersion of CeO2 and MoO3 on the surface of TiO2. CeO2 and MoO3 were still highly dispersed and existed as amorphous or highly dispersed species in CMT. However, the intensity of the diffraction peaks due to anatase TiO2 was found to increase with increasing Cl loadings. This meant that the presence of HCl served to weaken the interaction among CeO2, MoO3 and TiO2, thereby resulting in the deactivation of CMT.
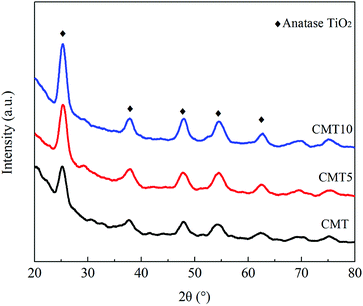 |
| Fig. 5 XRD patterns of the catalyst samples. | |
3.2.2 XPS analysis. The surface atomic concentrations of the catalyst samples are summarized in Table 1. The addition of HCl led to the decrease in the amount of reactive Ce and Mo atoms on the catalyst surface, which was in line with the catalytic activity. In addition, it was found that the measured Cl concentration on the catalyst surface was far lower than its nominal one. This might be due to its extraordinary high volatility.22
Table 1 Surface element compositions of the catalyst samples determined by XPS
Sample |
Surface atomic concentration (%) |
O |
Ti |
Mo |
Ce |
Cl |
Oβ/O |
Ce3+/Ce |
CMT |
66.53 |
27.71 |
3.09 |
2.68 |
— |
35.21 |
31.81 |
CMT10 |
64.73 |
29.42 |
2.64 |
2.01 |
1.20 |
23.62 |
34.76 |
As shown in Fig. 6, the binding energies of Ti 2p1/2 and Ti 2p3/2 in different catalysts were about 464.5 and 458.5 eV, respectively.23 It suggested that Ti in CMT and CMT10 existed in its highest oxidation state(IV).24 There was no significant change in the peak position among the two samples, but the intensities of both peaks increased after the introduction of Cl, which was in accordance with the XRD results. This meant that there existed an interaction between HCl and Ti.
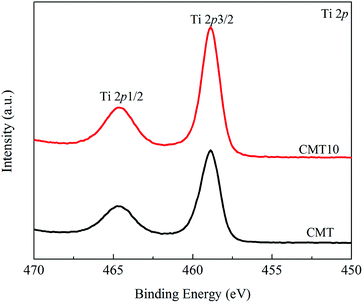 |
| Fig. 6 XPS spectra of Ti 2p for the catalyst samples. | |
After a curve-fitting procedure in Fig. 7, the spectra of O 1s can be deconvoluted into two peaks. The peak at about 529.7–530.1 eV could be assigned to lattice oxygen (denoted as Oα) and the other one at about 531.1–531.4 eV could be related to chemisorbed oxygen (denoted as Oβ) from oxide defects or hydroxyl-like groups.25,26 After treated by HCl, the Oβ/O ratio decreased from 35.21% to 23.62%, which was in good agreement with the catalytic activity. Oβ was considered to be more active in SCR reaction than Oα because of its higher mobility.27 As a result, the SCR activity of CMT decreased after loading HCl.
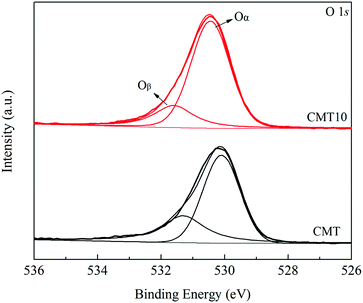 |
| Fig. 7 XPS spectra of O 1s for the catalyst samples. | |
The deconvoluted Ce 3d XPS results of the catalyst samples are shown in Fig. 8. The peaks labeled u, u′′, u′′′ and v, v′′, v′′′ are assigned to Ce4+, while u' and v' are attributed to Ce3+.28,29 The atomic ratio of Ce3+/Ce, calculated by the area of the corresponding peaks, increased from 31.81% to 34.76%. It indicated that the presence of HCl led to the increase in the amount of Ce3+ and the decrease in the amount of Ce4+ on the catalyst surface. It is widely accepted that Ce3+ can create a charge imbalance and form oxygen vacancies and unsaturated chemical bonds, which is helpful for the formation of chemisorbed oxygen on the catalyst surface.30 However, it was unexpected that the increase in the amount of Ce3+ due to the presence of HCl did not promote the formation of chemisorbed oxygen. On the contrary, the amount of chemisorbed oxygen decreased. It might be supposed that not all Ce3+ species could act as a promoter on SCR activity.
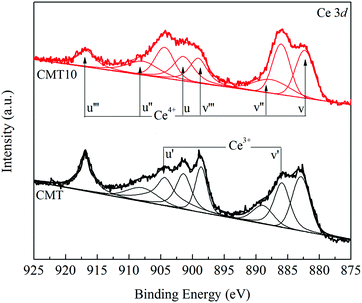 |
| Fig. 8 XPS spectra of Ce 3d for the catalyst samples. | |
Fig. 9 illustrates the XPS spectra of Cl 2p in CMT10. The peak appearing at 198.4 eV was assigned to Cl−.31 According to the handbook of X-ray photoelectron spectroscopy,32 it could be inferred that CeCl3 molecules might form on the surface of CMT10. It could be speculated that a fraction of CeO2 might react with HCl according to the following reaction:
|
2CeO2 + 8HCl → 2CeCl3 + Cl2 + 4H2O
| (3) |
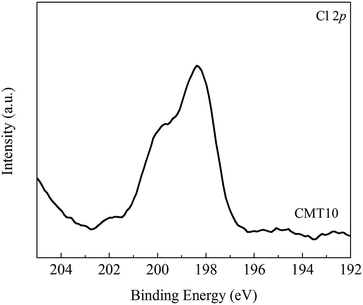 |
| Fig. 9 XPS spectra of Cl 2p for the CMT10 sample. | |
The presence of HCl could result in the transformation of Ce4+ into Ce3+. However, Ce3+ in the form of CeCl3 lost the ability to be converted into active Ce4+ and was unreactive in SCR reaction. Therefore, the Ce3+/Ce4+ redox cycle was damaged. It is generally accepted that the redox pairs of Ce3+/Ce4+ on catalyst surface are vital for the SCR reaction over CeO2-based catalyst.33 As a result, the catalytic performance of CMT decreased.
The Mo XPS profiles of catalyst samples are shown in Fig. 10. The binding energies of Mo 3d5/2 and Mo 3d3/2 were observed at 232.6 and 235.9 eV, and both spectra of the catalysts provide typical patterns for MoO3.34 Regardless of the peak positions or intensities, there was no obvious difference between CMT and CMT10 catalyst samples. It suggested that the chemical environment of Mo6+ remained almost the same after the introduction of Cl. Unlike active Ce species, MoO3 supported on TiO2 was not to be highly active for the SCR reaction.17 MoO3 primarily acted as a structural and chemical promoter rather than main active species. Compared with the Ce and Mo XPS results, it could be supposed that HCl preferentially reacted with active Ce species instead of Mo species. Consequently, Mo XPS curve was not changed in the presence of HCl.
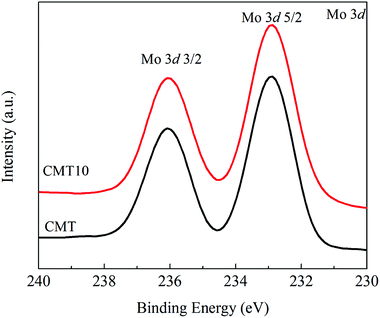 |
| Fig. 10 XPS spectra of Mo 3d for the catalyst samples. | |
3.2.3 NH3-TPD analysis. NH3-TPD analysis was performed to study the surface acidity of the catalyst samples and the results are presented in Fig. 11. Each catalyst sample contained a broad NH3 desorption peak centered at about 200 °C, which could be assigned to the weakly absorbed NH3 on Brønsted acid sites.21,24 As for CMT, one small peak centered at about 600 °C was observed and could be attributed to the strongly absorbed NH3 on Lewis acid sites.35 The presence of HCl resulted in the decrease in the NH3 desorption peak area at low and high temperatures. Furthermore, the peak at high temperature shifted to higher temperatures. It indicated that the Brønsted acid sites and Lewis acid sites on the surface of CMT decreased, thereby inhibiting the adsorption of NH3 species on the catalyst. As a result, HCl has a negative effect on the activity of CMT.
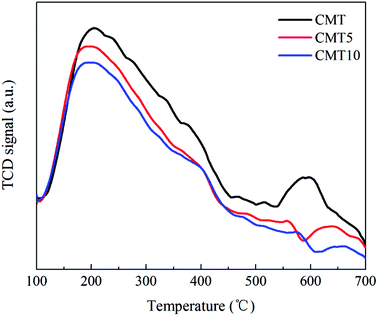 |
| Fig. 11 NH3-TPD profiles of the catalyst samples. | |
3.2.4 H2-TPR analysis. Apart from the surface acidity of the catalyst, redox property is also another key factor which influences the performance of SCR catalyst. Fig. 12 illustrated the H2-TPR profiles of the catalyst samples. The TPR of the fresh CMT illustrated two overlapping reduction peaks, at 536 °C and 612 °C, indicating the co-reduction of surface Ce and well dispersed octahedral Mo species.36,37 After adding HCl, the peak at 612 °C disappeared while the peak at 536 °C moved to higher temperature. It was possible that the introduction of HCl make the two peaks overlap. Jin et al.38 reported the similar phenomenon on CeO2/TiO2 catalyst with the modulation of HF. Nevertheless, the H2 consumption decreased in the order: CMT > CMT5 > CMT10. It indicated that reducibility of CMT was weakened due to the presence of HCl. It was clear that HCl should be responsible for the strong inhibition in the catalytic activity of CMT.
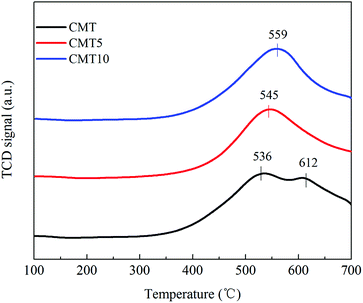 |
| Fig. 12 H2-TPR profiles of the catalyst samples. | |
4. Conclusions
In this work, the influence of HCl on the selective catalytic reduction of NO with NH3 over CeO2–MoO3/TiO2 catalyst was investigated. The experimental results showed that HCl had a negative effect on the SCR performance of CeO2–MoO3/TiO2 catalyst. The presence of HCl led to pore blockage, weakened interaction among CeO2, MoO3 and TiO2, reduction in surface acidity and degradation of redox ability. Though the amount of Ce3+ increased on the catalyst surface after adding HCl, Ce3+ in the form of CeCl3 was not active in SCR reaction. The Ce3+/Ce4+ redox cycle was damaged. In addition, the concentration of surface Ce and Mo atoms and the amount of chemisorbed oxygen were found to decrease. All of these factors were responsible for the deactivation by HCl of CMT.
Conflicts of interest
There are no conflicts to declare.
Acknowledgements
This work was supported by the National Natural Science Foundation of China (No. 51506226), Natural Science Foundation of Shandong Province (No. ZR2015EM010), “the Fundamental Research Funds for the Central Universities” (No. 15CX05005A) and the scholarship from China Scholarship Council, China (CSC) (No. 201706455013).
References
- H. Chang, X. Chen, J. Li, L. Ma, C. Wang, C. Liu, W. S. Johannes and J. Hao, Improvement of activity and SO2 tolerance of Sn-modified MnOx–CeO2 catalysts for NH3-SCR at low temperatures, Environ. Sci. Technol., 2013, 47(10), 5294–5301 CrossRef PubMed.
- X. Du, J. Xue, X. Wang, Y. Chen, J. Ran and L. Zhang, Oxidation of sulfur dioxide over V2O5/TiO2 catalyst with low vanadium loading: a theoretical study, J. Phys. Chem. C, 2018, 122(8), 4517–4523 Search PubMed.
- L. Lietti, I. Nova and P. Forzatti, Selective catalytic reduction (SCR) of NO by NH3 over TiO2-supported V2O5–WO3 and V2O5–MoO3 catalysts, Top. Catal., 2000, 11–12, 111–122 CrossRef.
- C. Tang, H. Zhang and L. Dong, Ceria-based catalysts for low-temperature selective catalytic reduction of NO with NH3, Catal. Sci. Technol., 2016, 6(5), 1248–1264 Search PubMed.
- R. Guo, P. Sun, W. Pan, M. Li, S. Liu, X. Sun, S. Liu and J. Liu, A highly effective MnNdOx catalyst for the selective catalytic reduction of NOx with NH3, Ind. Eng. Chem. Res., 2017, 56(44), 12566–12577 CrossRef.
- X. Gao, X. Du, L. Cui, Y. Fu, Z. Luo and K. Cen, A Ce–Cu–Ti oxide catalyst for the selective catalytic reduction of NO with NH3, Catal. Commun., 2010, 12(4), 255–258 CrossRef.
- W. Shan, F. Liu, Y. Yu and H. He, The use of ceria for the selective catalytic reduction of NOx with NH3, Chin. J. Catal., 2014, 35, 1251–1259 CrossRef.
- Z. Liu, H. Su, J. Li and Y. Li, Novel MoO3/CeO2–ZrO2 catalyst for the selective catalytic reduction of NOx by NH3, Catal. Commun., 2015, 65, 51–54 CrossRef.
- Z. Liu, Y. Yi, J. Li, S. Woo, B. Wang, X. Cao and Z. Li, A superior catalyst with dual redox cycles for the selective reduction of NOx by ammonia, Chem. Commun., 2013, 49(70), 7726–7728 RSC.
- Z. Liu, J. Zhu, J. Li, L. Ma and S. Woo, Novel Mn−Ce−Ti mixed-oxide catalyst for the selective catalytic reduction of NOx with NH3, ACS Appl. Mater. Interfaces, 2014, 6, 14500–14508 Search PubMed.
- Y. Jiang, Z. Xing, X. Wang, S. Huang, Q. Liu and J. Yang, MoO3 modified CeO2/TiO2 catalyst prepared by a single step sol–gel method for selective catalytic reduction of NO with NH3, J. Ind. Eng. Chem., 2015, 29, 43–47 CrossRef.
- J. P. Chen, M. A. Buzanowski, R. T. Yang and J. E. Cichanowicz, Deactivation of the vanadia catalyst in the selective catalytic reduction process, J. Air Waste Manage. Assoc., 1990, 40(10), 1403–1409 Search PubMed.
- L. Lisi, G. Lasorella, S. Malloggi and G. Russo, Single and combined deactivating effect of alkali metals and HCl on commercial SCR catalysts, Appl. Catal., B, 2004, 50(4), 251–258 CrossRef.
- N. Z. Yang, R. T. Guo and W. G. Pan, et al. The deactivation mechanism of Cl on Ce/TiO2 catalyst for selective catalytic reduction of NO with NH3, Appl. Surf. Sci., 2016, 378, 513–518 CrossRef.
- Y. Hou, G. Cai, Z. Huang, X. Han and S. Guo, Effect of HCl on V2O5/AC catalyst for NO reduction by NH3 at low temperatures, Chem. Eng. J., 2014, 247, 59–65 CrossRef.
- X. Yu, F. Cao, X. Zhu, X. Zhu, X. Gao, Z. Luo and K. Cen, Selective catalytic reduction of NO over Cu–Mn/OMC catalysts: effect of preparation method, Aerosol Air Qual. Res., 2017, 17, 302–313 CrossRef.
- Y. Jiang, X. Zhang, M. Lu, C. Bao, G. Liang, C. Lai, W. Shi and S. Ma, Activity and characterization of Ce–Mo–Ti mixed oxide catalysts prepared by a homogeneous precipitation method for selective catalytic reduction of NO with NH3, J. Taiwan Inst. Chem. Eng., 2018, 86, 133–140 CrossRef.
- M. Casapu, O. Kröcher and M. Elsener, Screening of doped MnOx–CeO2 catalysts for low-temperature NO-SCR, Appl. Catal., B, 2009, 88, 413–419 CrossRef.
- W. Xu, H. He and Y. Yu, Deactivation of a Ce/TiO2 catalyst by SO2 in the selective catalytic reduction of NO by NH3, J. Phys. Chem., 2009, 113, 4426–4432 Search PubMed.
- Y. Peng, C. Liu, X. Zhang and J. Li, The effect of SiO2 on a novel CeO2–WO3/TiO2 catalyst for the selective catalytic reduction of NO with NH3, Appl. Catal., B, 2013, 140–141, 276–282 CrossRef.
- P. Wang, Q. S. Wang, X. X. Ma, R. T. Guo and W. G. Pan, The influence of F and Cl on Mn/TiO2, catalyst for selective catalytic reduction of NO with NH3: a comparative study, Catal. Commun., 2015, 71, 84–87 CrossRef.
- X. Wu, W. Yu, Z. Si and D. Weng, Chemical deactivation of V2O5–WO3/TiO2 SCR catalyst by combined effect of potassium and chloride, Front. Environ. Sci. Eng., 2013, 7(3), 420–427 CrossRef.
- X. Gao, Y. Jiang, Y. Zhong, Z. Luo and K. Cen, The activity and characterization of CeO2–TiO2 catalysts prepared by the sol–gel method for selective catalytic reduction of NO with NH3, J. Hazard. Mater., 2010, 174(1–3), 734–739 CrossRef PubMed.
- X. Du, X. Gao, L. Cui, Y. Fu, Z. Luo and K. Cen, Investigation of the effect of Cu addition on the SO2-resistance of a CeTi oxide catalyst for selective catalytic reduction of NO with NH3, Fuel, 2012, 92(1), 49–55 CrossRef.
- Y. Peng, K. Li and J. Li, Identification of the active sites on CeO2–WO3 catalysts for SCR of NOx with NH3: an in situ IR and Raman spectroscopy study, Appl. Catal., B, 2013, 140–141, 483–492 CrossRef.
- N. Z. Yang, R. T. Guo, W. G. Pan, Q. L. Chen, Q. S. Wang and C. Z. Lu, The promotion effect of Sb on the Na resistance of Mn/TiO2 catalyst for selective catalytic reduction of NO with NH3, Fuel, 2016, 169, 87–92 CrossRef.
- H. Chang, J. Li, J. Yuan, L. Chen, Y. Dai, A. Hamidreza, J. Xu and J. Hao, Ge, Mn-doped CeO2–WO3 catalysts for NH3-SCR of NOx: effects of SO2 and H2 regeneration, Catal. Today, 2013, 201(1), 139–144 CrossRef.
- W. Shan, F. Liu, H. He, X. Shi and C. Zhang, A superior Ce–W–Ti mixed oxide catalyst for the selective catalytic reduction of NOx with NH3, Appl. Catal., B, 2012, 115–116, 100–106 CrossRef.
- D. Devaiah, D. Jampaiah, P. Saikia and B. M. Reddy, Structure dependent catalytic activity of Ce0.8Tb0.2O2–δ and TiO2 supported Ce0.8Tb0.2O2–δ solid solutions for CO oxidation, J. Ind. Eng. Chem., 2014, 20(2), 444–453 CrossRef.
- R. T. Guo, C. Z. Lu, W. G. Pan, W. L. Zhen, Q. S. Wang, Q. L. Chen, H. L. Ding and N. Z. Yang, A comparative study of the poisoning effect of Zn and Pb on Ce/TiO2 catalyst for low temperature selective catalytic reduction of NO with NH3, Catal. Commun., 2015, 59, 136–139 CrossRef.
- F. Y. Chang, J. C. Chen and M. Y. Wey, Activity and characterization of Rh/Al2O3 and Rh–Na/Al2O3 catalysts for the SCR of NO with CO in the presence of SO2 and HCl, Fuel, 2010, 89(8), 1919–1927 CrossRef.
- C. Wagner, W. Riggs, L. Davis, J. Moulder and G. Muilenberg, Hand book of X-Ray Photoelectron Spectroscopy, Perkin-Elmer Corporation, 1st. minnesota, 1979 Search PubMed.
- X. Li, X. Li, J. Li and L. Hao, Identification of the arsenic resistance on MoO3 doped CeO2/TiO2 catalyst for selective catalytic reduction of NOx with ammonia, J. Hazard. Mater., 2016, 318, 615–622 CrossRef PubMed.
- H. L. Koh and H. K. Park, Characterization of MoO3–V2O5/Al2O3 catalysts for selective catalytic reduction of NO by NH3, J. Ind. Eng. Chem., 2013, 19(1), 73–79 CrossRef.
- R. Zhang, Q. Zhong and W. Zhao, et al. Promotional effect of fluorine on the selective catalytic reduction of NO with NH3 over CeO2–TiO2 catalyst at low temperature, Appl. Surf. Sci., 2014, 289(289), 237–244 CrossRef.
- Z. Liu, S. Zhang, J. Li and L. Ma, Promoting effect of MoO3 on the NOx reduction by NH3 over CeO2/TiO2 catalyst studied with in situ DRIFTS, Appl. Catal., B, 2014, 144(1), 90–95 CrossRef.
- L. Caero, A. Romero and J. Ramirez, Niobium sulfide as a dopant for Mo/TiO2 catalysts, Catal. Today, 2003, 78, 513–518 CrossRef.
- Q. Jin, Y. Shen and S. Zhu, Effect of fluorine additive on CeO2(ZrO2)/TiO2 for selective catalytic reduction of NO by NH3, J. Colloid Interface Sci., 2017, 487, 401–409 CrossRef PubMed.
|
This journal is © The Royal Society of Chemistry 2018 |