DOI:
10.1039/C8RA00271A
(Paper)
RSC Adv., 2018,
8, 11419-11423
A novel glutathione-triggered theranostic prodrug for anticancer and imaging in living cells†
Received
10th January 2018
, Accepted 7th March 2018
First published on 22nd March 2018
Abstract
A novel theranostic prodrug was designed and synthesized by conjugating a naphthalimide derivative with vitamin D2 via a disulfide linker. The prodrug featured a highly selective detection process for glutathione (GSH) and showed a red-shifted fluorescence within 30 min. Notably, it also exhibited antitumor activity similar to vitamin D2 and could be monitored by cellular imaging.
1 Introduction
Theranostics is an emerging technology, which is defined as a combination of therapy and diagnostic imaging. Due to its potential to be targeted in a specific manner to the site of disease and reduce or eliminate the possible numerous untoward side effects, theranostics has attracted considerable attention.1,2 Until now, various imaging techniques have been used for theranostics, such as ultrasound,3 computed tomography (CT),4 positron emission tomography (PET)5 and magnetic resonance imaging (MRI).6 However, these methods always suffered from some drawbacks, including poor resolution and high costs.7 In contrast to these conventional methods, naphthalimide-based fluorescence imaging has been developing rapidly due to the advantages of low cost, convenient preparation and high resolution.8–11
Biological thiols including glutathione (GSH), cysteine (Cys) and homocysteine (Hcy) always play crucial roles in biological systems.12–17 An abnormal level of biothiols could be an indicator in many diseases. Given that cancer cells have much higher intracellular glutathione (GSH) concentration than normal cells and tissues, considerable efforts have been devoted to the development of GSH-activatable prodrugs.18–20 Due to its high sensitivity and rapid response to the disulfide bond, GSH has been broadly utilized as a trigger to cleave the disulfide-containing prodrug and release the active drug. For instance, Zhou et al. designed and synthesized a prodrug for cancer treatment by conjugating a naphthalimide derivative and chlorambucil (CLB) via a disulfide linker.21 Kim et al. also conjugated a naphthalimide derivative with camptothecin (CPT) using a disulfide linker, which was highly desirable for in situ fluorescence-tracking of cancer chemotherapy.22
As an important sterol, vitamin D plays a critical role in calcium homeostasis and bone mineralization and also regulates the proliferation and differentiation of various types of cancer cells.23–27 However, there has been no report about vitamin D-based theranostic prodrug before. Inspired by this and as continuation of our study, herein, we report a novel theranostic prodrug, which can release vitamin D2 in the presence of GSH and exhibit distinct fluorescence variation.28–30
2 Results and discussion
2.1 Design and synthesis of prodrug
The theranostic prodrug was designed using three elements. (1) A naphthalimide derivative served as fluorescent reporter, which could be synthesized conveniently from the corresponding 1,8-naphthalic anhydrides by reacting with ethylamine. (2) A disulfide linker that could be cleaved by GSH with high sensitivity and rapid response. (3) An anticancer drug vitamin D2, which could be used to regulate gene expression in functions as varied as calcium and phosphate homeostasis, cancer cell growth regulation and differentiation.
Treatment of 4-bromo-1,8-naphthalic anhydride in DMF with sodium azide at room temperature provided the compound 2. It was then reduced with H2 and 10% Pd/C as catalyst to afford the compound 3. Without any purification, the obtained naphthalic anhydride was condensed with ethylamine to give the key intermediate 4, which was then reacted with triphosgene and 2,2-dithiolethanol to afford compound 5. Finally, the desired theranostic prodrug was obtained by reacting compound 5 with vitamin D2 (Scheme 1). The structure of prodrug was characterized by 1H NMR, 13C NMR and HRMS.
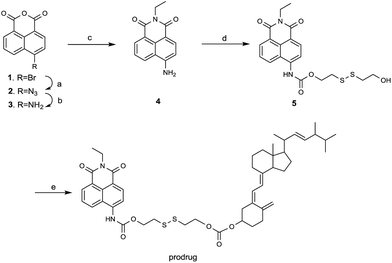 |
| Scheme 1 Synthesis of prodrug (a) NaN3, DMF, 89.3%; (b) 10% Pd/C, H2, DMF, 36 h, 80.1%; (c) ethylamine, ethanol, 4 h, 89.1%; (d) triphosgene, triethylamine, 2-hydroxyethyl disulfide, DCM, 73.2%; (e) triphosgene, triethylamine, vitamin D2, 39.1%. | |
2.2 Rapid optical response of the prodrug to GSH
As expected, the prodrug displayed both colorimetric and fluorescence spectral changes upon the addition of GSH. When GSH (200 μM) was added to the PBS/DMSO solution containing prodrug (10 μM), the absorption peak at 374 nm decreased sharply, and a new band centered at 437 nm could be observed (Fig. 1a). Meanwhile, significant fluorescence turn-on signal changes at 534 nm were observed. Thus, the solution revealed strong yellow fluorescence (Fig. 1b). The fluorescence intensity at 534 nm exhibited a good linear relationship with the GSH concentration and the detection limit was 1.98 μM (Fig. 1c). In addition, the fluorescence intensity of prodrug at 534 nm gradually increased to a plateau within 30 min (Fig. 1d). The time-dependent release efficiency of prodrug in the presence of GSH was displayed in Fig. S1.†
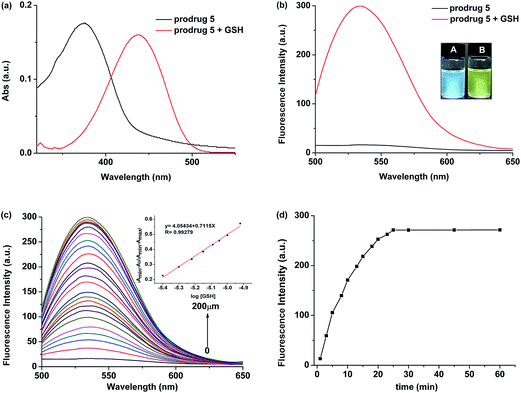 |
| Fig. 1 (a) UV-Vis spectra changes and (b) fluorescent spectra changes of prodrug (10 μM) before and after incubation with GSH (200 μM) in PBS/DMSO (40 : 60, v/v, pH = 7.4, 10 mM) at 37 °C for 30 min. Fluorescence of prodrug in the absence (A) and in the presence (B) of GSH are inserted. (c) Fluorescence intensity changes of prodrug (10 μM) at 534 nm upon the addition of different concentrations of GSH in PBS. Inset: the linear relationship between fluorescent intensity and GSH concentration (d) time dependence of fluorescence intensity of prodrug in the presence of GSH. | |
2.3 The optical pH range and selectivity of the prodrug for GSH
Similar spectroscopic changes could be observed upon the addition of other free thiols, such as Cys and Hcy. Moreover, the presence of other analytes including amino acids and metal ions induced small or no fluorescence change (Fig. 2a). However, since the concentration of Cys and Hcy was lower than that of GSH in cytoplasm, the possible interference of Cys and Hcy could be neglected. The fluorescence intensity of the prodrug was unaffected over a wide pH range and indicated that the prodrug was quite stable. When GSH (200 μM) was added to the solution, a dramatic fluorescence enhancement could be observed over a pH range of 6–10 (Fig. 2b). These results demonstrated that the prodrug can be applied as a GSH-triggered prodrug in theranostic system with high selectivity over various potential interferons.
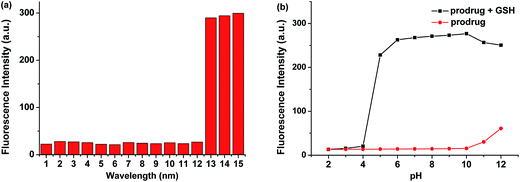 |
| Fig. 2 (a) Fluorescent spectra responses of prodrug for various analytes (1–14 represent: (1) Al3+, (2) Cu2+, (3) NO3−, (4) SO42−, (5) Leu, (6) Tyr, (7) Arg, (8) Glu, (9) Lys, (10) Thr, (11) Ser, (12) Hcy, (13) Cys, (14) GSH). (b) The pH effect of the fluorescence intensity of prodrug in the absence and in the presence of GSH. Each spectrum was collected after 30 min of mixing each analyte with prodrug in PBS/DMSO (40 : 60, v/v, pH = 7.4, 10 mM) at 37 °C. | |
2.4 Proposed response mechanism of the prodrug for GSH
The proposed mechanism of the prodrug for GSH was displayed by a two-step reaction: cleavage of the disulfide bond and intramolecular cyclization (Scheme 2).31–33 MS and spectroscopic studies were used to verify the hypothesis. First, the spectra of compound 4 were consistent with that of the prodrug after the treatment with GSH, suggesting that compound 4 was the product (Fig. S2†). Furthermore, ESI-MS titration experiment was carried out to prove the anticipated release of vitamin D2. The peaks at m/z of 279.07 and 435.35 were observed, which were attributed to [compound 4 + K]+ and [vitamin D2 + K]+ (Fig. S3†). These results were indicative of the cleavage of the disulfide group and the production of fluorophore and vitamin D2 in the sensing reaction.
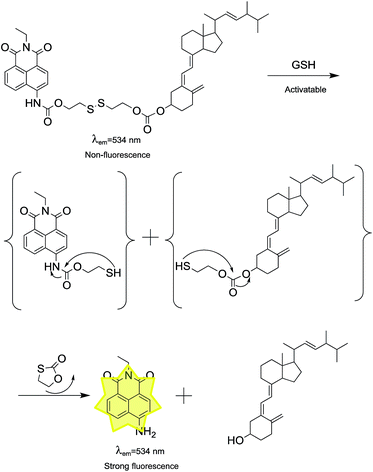 |
| Scheme 2 Proposed response mechanism of the prodrug for GSH. | |
2.5 Antitumor activity evaluation and live cell imaging
The prodrug displayed good biocompatibility to HEK 293T cells (Fig. S4†). Inspired by above results, we further investigated the practicability of the prodrug in biological systems by carrying out antitumor activity and bioimaging experiments. To determine the antitumor activity, vitamin D2, compound 5 and prodrug were first incubated with HeLa cells and then evaluated by using typical MTT assays. Prodrug and vitamin D2 showed similar antitumor activity against HeLa cell lines, while compound 5 showed low activity for living cells (Fig. 3). Then, the cellular uptake and intracellular localization of prodrug were investigated using a fluorescence microscope. When HeLa cells were incubated with the prodrug for 30 min, weak fluorescence from prodrug was observed, indicating that the prodrug was sufficiently activated by the high concentration of GSH in cancer cells. To demonstrate the role of the GSH presented in disulfide cleavage, additional 1.0 mM prodrug was added to HeLa cells and an enhanced fluorescence was observed (Fig. 4). These results were fully consistent with the design expectations and suggested that the prodrug can be used to monitor the drug release process in cancer cells.
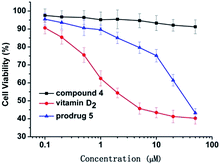 |
| Fig. 3 Antitumor activity of compound 5, vitamin D2 and prodrug on HeLa cancer cells. | |
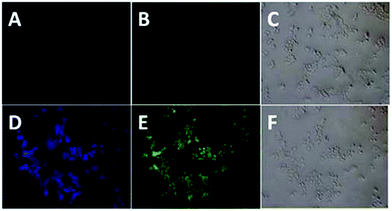 |
| Fig. 4 The upside: images of HeLa cells incubated only with the prodrug (10 μM) for 30 min; (A) fluorescence image at the blue channel, (B) fluorescence image at the green channel, (C) bright-field image. The downside: images of HeLa cells incubated with GSH (1.0 mM) for 30 min, then treated with the prodrug (10 μM) for 30 min. (D) Fluorescence image at the blue channel, (E) fluorescence image at the green channel, (F) bright-field image. | |
3 Conclusion
In summary, we developed a novel theranostic prodrug by conjugating the naphthalimide chromophore and vitamin D2 via a disulfide bond. This prodrug can discriminate GSH from a wide array of amino acids and ions. It also can be used to quantify GSH with a detection limit as low as 1.98 μM and rapid detection process for GSH within 30 min. In addition, this prodrug displayed similar antitumor activity with vitamin D2 and can be used for intracellular fluorescence imaging. Overall, this prodrug can be used as a valuable research tool for GSH-activatable drug delivery system and can be easily monitored by cellular imaging.
4 Experimental procedures
4.1 Materials and apparatus
All chemical reagents and solvents were purchased from commercial suppliers and used without further purification. 1H and 13C NMR spectra were obtained on Bruker Avance III 500 MHz spectrometer. HRMS spectra were obtained on a Bruker ApexII by means of the ESI technique. Absorption and fluorescence spectra were obtained on Lambda 35 UV-Vis spectrophotometer and a Shimadzu RF-5301PC Fluorescence Spectrometer, respectively. All titrations were carried out in PBS/DMSO solution (40
:
60, v/v, pH = 7.4).
4.2 Synthesis of compound 4
Initially, 4-bromo-1,8-naphthalic anhydride (10 g, 36.1 mmol) was dissolved in DMF (50 mL) and then, NaN3 (3.5 g, 53.8 mmol) was added into the solution. The mixture was stirred overnight and added to water to obtain yellow precipitate. The precipitate was filtered and dried to obtain compound 2 (7.6 g, 89.3%).
Compound 2 (5 g, 20.8 mmol) was dissolved in DMF (50 mL), followed by the addition of 10% Pd/C (400 mg). Then, the mixture was stirred under H2 atmosphere at room temperature for 36 h. After filtration, water was added into the solution to obtain yellow precipitate. The precipitate was filtered and dried to give compound 3 (3.5 g, 80.1%).
Compound 3 (3 g, 14.1 mmol) was dissolved in ethanol (160 mL), following which ethylamine (4 mL) was added dropwise. The mixture was refluxed for 4 h and then added to water to obtain precipitate. The precipitate was filtered and dried to yield compound 4 (3.7 g, 89.1%). 1H NMR (500 MHz, DMSO): δ 8.51 (1H, d, J = 8.1 Hz), 8.30 (1H, d, J = 7.2 Hz), 8.10 (1H, d, J = 8.4 Hz), 7.65 (1H, m), 7.33 (2H, s), 6.76 (1H, d, J = 8.4 Hz), 3.97 (2H, d, J = 7.1 Hz), 1.11 (3H, t, J = 7.0 Hz) ppm; 13C NMR (126 MHz, DMSO): δ 162.58, 152.11, 133.30, 130.34, 128.89, 123.37, 121.27, 118.82, 107.36, 33.68, 12.78 ppm.
4.3 Synthesis of compound 5
Compound 4 (0.5 g, 1.1 mmol) and triphosgene (1.2 g, 4.0 mmol) were dissolved in dichloromethane (30 mL) and stirred for 0.5 h at 0 °C, following which triethylamine (1.7 mL) was added and stirred for another 60 min. Then, 2-hydroxyethyl disulfide (2.8 mL) was added and stirred at room temperature to obtain yellow precipitate. The precipitate was filtered and dried to give compound 5 (1.3 g, 73.2%). 1H NMR (500 MHz, DMSO): δ 10.29 (1H, s), 8.64 (1H, d, J = 8.5 Hz), 8.40 (2H, m, J = 7.7 Hz), 8.13 (1H, d, J = 8.2 Hz), 7.76 (1H, s), 4.49 (2H, t, J = 6.4 Hz), 4.05 (2H, d, J = 7.1 Hz), 3.69 (2H, d, J = 6.4 Hz), 3.14 (2H, t, J = 6.4 Hz), 2.90 (2H, t, J = 6.4 Hz), 1.22 (3H, t, J = 7.0 Hz) ppm; 13C NMR (126 MHz, DMSO): δ 162.58, 162.02, 153.25, 139.97, 130.89, 130.14, 128.60, 127.58, 125.62, 123.15, 121.53, 117.52, 116.44, 62.48, 58.92, 40.62, 36.22, 34.08, 12.58 ppm.
4.4 Synthesis of prodrug
Compound 5 (0.2 g, 0.5 mmol) and triphosgene (0.62 g, 2.01 mmol) were dissolved in dichloromethane (30 mL) and stirred for 0.5 h at 0 °C, following which triethylamine (1.69 mL) was added and stirred for another 60 min. Then, vitamin D2 (0.2 g, 0.05 mmol) was added and stirred at room temperature until the reaction was complete. Finally, the mixture was concentrated under reduced pressure and purified by column chromatography on silica gel to obtain the prodrug (0.15 g, 39.1%). 1H NMR (500 MHz, CDCl3): δ 8.62 (1H, m, J = 13.2 Hz), 8.33 (1H, m, J = 8.4 Hz), 7.84 (1H, s), 7.80 (1H, m), 6.19 (1H, d, J = 11.2 Hz), 5.98 (1H, d, J = 11.2 Hz), 5.17 (1H, m, J = 7.4 Hz), 5.04 (1H, s), 4.89 (1H, m), 4.55 (1H, t, J = 6.1 Hz), 4.51 (1H, m), 4.24 (1H, q, J = 7.1 Hz), 3.15 (2H, m), 2.81 (1H, m), 2.60 (1H, m, J = 13.4 Hz), 2.40 (1H, m, J = 6.2 Hz), 2.21 (1H, m), 2.08 (2H, m), 1.89 (1H, m), 1.75 (3H, m), 1.52 (3H, m), 1.37 (5H, m), 1.05 (2H, m), 0.96 (6H, m), 0.52 (2H, s) ppm; 13C NMR (126 MHz, CDCl3): δ 162.96, 162.46, 153.53, 152.12, 143.01, 141.77, 138.09, 134.59, 132.49, 131.17, 130.22, 127.87, 125.54, 122.12, 116.54, 112.20, 75.17, 64.62, 62.28, 55.43, 44.81, 41.82, 40.99, 39.39, 36.55, 35.73, 34.51, 32.12, 30.83, 28.06, 26.80, 22.58, 21.22, 20.13, 18.84, 16.62, 12.39, 11.26 ppm. HRMS [M + H]+: calcd for C48H62N2O7S2 842.3998, found 843.4071.
4.5 Absorption and fluorescence spectroscopy
Stock solution of prodrug, vitamin D2 and compound 4 (2.0 × 10−3 M) was prepared in DMSO. Individually, stock solutions (1 mM) of the analytes Cys, Hcy, GSH, leucine (Leu), tyrosine (Tyr), arginine (Arg), glutamic acid (Glu), lysine (Lys), threonine (Thr), and serine (Ser) were prepared in ultrapure water. For a typical optical study, the prodrug (10 μM) solution in PBS/DMSO (40
:
60, v/v, pH = 7.4, 10 mM) was prepared. Then, 3.0 mL of the solution was placed in a quartz cuvette at room temperature. For fluorescent measurements, slit width was set at dex = 3 nm, dem = 3 nm.
4.6 Cell incubation and imaging
HeLa cells used in this study were purchased from Cobioer Biosciences Co., Ltd. (Nanjing, China). HEK 293T cells used in this study were purchased from Chinese Academy of Sciences (Shanghai, China). HeLa cells were cultured in Dulbecco's modified Eagle's medium (DMEM) supplemented with 10% fetal bovine serum (FBS) at 37 °C in an atmosphere of 5% CO2. The images of cells were visualized and photographed by a fluorescence microscope (Nikon, Japan). In the experiment of cell imaging, cells were incubated with 10 μM of prodrug (with 0.2% DMSO, v/v) for 30 min at 37 °C, washed with pre-warmed PBS thrice and then imaged. For GSH treated experiments, the HeLa cells were pretreated with 1.0 mM GSH at 37 °C for 30 min, washed with PBS three times, and then incubated with 10 μM prodrug at 37 °C for 30 min. Cell imaging was then carried out after washing cells with prewarmed PBS.
Conflicts of interest
There are no conflicts to declare.
Notes and references
- A. Gharatape and R. Salehi, Eur. J. Med. Chem., 2017, 138, 221–233 CrossRef CAS PubMed.
- S. S. Kelkar and T. M. Reineke, Bioconjugate Chem., 2011, 22, 1879–1903 CrossRef CAS PubMed.
- J. Chen, S. Ratnayaka, A. Alford, V. Kozlovskaya, F. Liu, B. Xue, K. Hoyt and E. Kharlampieva, ACS Nano, 2017, 11, 3135–3146 CrossRef CAS PubMed.
- S. Moeendarbari, R. Tekade, A. Mulgaonkar, P. Christensen, S. Ramezani, G. Hassan, R. Jiang, O. K. Öz, Y. Hao and X. Sun, Sci. Rep., 2016, 6, 20614–20622 CrossRef CAS PubMed.
- J. Guo, H. Hong, G. Chen, S. Shi, T. R. Nayak, C. P. Theuer, T. E. Barnhart, W. Cai and S. Gong, ACS Appl. Mater. Interfaces, 2014, 6, 21769–21779 CAS.
- J. Kaur, Y. Tsvetkova, K. Arroub, S. Sahnoun, F. Kiessling and S. Mathur, Chem. Biol. Drug Des., 2017, 89, 269–276 CAS.
- M. G. Sikkandhar, A. M. Nedumaran, R. Ravichandar, S. Singh, I. Santhakumar, Z. C. Goh, S. Mishra, G. Archunan, B. Gulyás and P. Padmanabhan, Int. J. Mol. Sci., 2017, 18, 1036–1063 CrossRef PubMed.
- K. H. Hong, D. I. Kim, H. Kwon and H. J. Kim, RSC Adv., 2013, 4, 978–982 RSC.
- S. Banerjee, E. B. Veale, C. M. Phelan, S. A. Murphy, G. M. Tocci, L. J. Gillespie, D. O. Frimannsson, J. M. Kelly and T. Gunnlaugsson, Chem. Soc. Rev., 2013, 42, 1601–1618 RSC.
- L. Rong, C. Zhang, Q. Lei, H. L. Sun, S. Y. Qin, J. Feng and X. Z. Zhang, Chem. Commun., 2015, 51, 388–390 RSC.
- H. S. Kim, W. Y. Song and H. J. Kim, Org. Biomol. Chem., 2015, 13, 73–76 CAS.
- S. Zhang, C. N. Ong and H. M. Shen, Cancer Lett., 2004, 208, 143–153 CrossRef CAS PubMed.
- X. Chen, Y. Zhou, X. Peng and J. Yoon, Chem. Soc. Rev., 2010, 39, 2120–2135 RSC.
- J. Lačná, F. Foret and P. Kubáň, Electrophoresis, 2016, 38, 203–222 CrossRef PubMed.
- Q. Sun, D. Sun, L. Song, Z. Chen, Z. Chen, W. Zhang and J. Qian, Anal. Chem., 2016, 28, 177–221 Search PubMed.
- L. Song, H. Tian, X. Pei, Z. Zhang, W. Zhang and J. Qian, RSC Adv., 2015, 5, 59056–59061 RSC.
- J. Qian, L. Song, Q. Sun, N. Wang, Z. Chen and W. Zhang, Anal. Methods, 2015, 7, 10371–10375 RSC.
- M. H. Lee, J. H. Han, P. S. Kwon, S. Bhuniya, J. Y. Kim, J. L. Sessler, C. Kang and J. S. Kim, J. Am. Chem. Soc., 2012, 134, 1316–1322 CrossRef CAS PubMed.
- F. Kong, Z. Liang, D. Luan, X. Liu, K. Xu and B. Tang, Anal. Chem., 2016, 88, 6450–6456 CrossRef CAS PubMed.
- X. Wu, X. Sun, Z. Guo, J. Tang, Y. Shen, T. D. James, T. He and W. Zhu, J. Am. Chem. Soc., 2014, 136, 3579–3588 CrossRef CAS PubMed.
- J. Wu, R. Huang, C. Wang, W. Liu, J. Wang, X. Weng, T. Tian and X. Zhou, Org. Biomol. Chem., 2013, 11, 580–585 CAS.
- M. H. Lee, Z. Yang, C. W. Lim, Y. H. Lee, S. Dongbang, C. Kang and J. S. Kim, Chem. Rev., 2013, 113, 5071–5109 CrossRef CAS PubMed.
- K. K. Deeb, D. L. Trump and C. S. Johnson, Nat. Rev. Cancer, 2007, 7, 684–700 CrossRef CAS PubMed.
- J. L. Costa, P. P. Eijk, M. A. van de Wiel, D. ten Berge, F. Schmitt, C. J. Narvaez, J. Welsh and B. Ylstra, BMC Genomics, 2009, 10, 499–504 CrossRef PubMed.
- G. Jones, Endocrinol. Metab. Clin. North Am., 2010, 39, 447–472 CAS.
- X. Gu, Q. Chen and Z. Fang, Dyes Pigm., 2017, 139, 334–343 CrossRef CAS.
- T. Zhang, T. Wang and Z. Fang, RSC Adv., 2016, 6, 18357–18363 RSC.
- L. Li and Z. Fang, Spectrosc. Lett., 2015, 48, 578–585 CrossRef CAS.
- W. Guo, Z. Fang, H. Li and Y. Liu, J. Chem. Res., 2014, 38, 231–235 CrossRef CAS.
- H. Li, Z. Fang, H. Dai, H. Zhang and Y. Liu, J. Chem. Res., 2015, 39, 368–372 CrossRef CAS.
- Y. Zhang, Q. Yin, J. Yen, J. Li, H. Ying, H. Wang, Y. Hua, E. J. Chaney, S. A. Boppart and J. Cheng, Chem. Commun., 2015, 51, 6948–6951 RSC.
- M. Ye, X. Wang, J. Tang, Z. Guo, Y. Shen, H. Tian and W. H. Zhu, Chem. Sci., 2016, 7, 4958–4965 RSC.
- Z. Yang, J. H. Lee, H. M. Jeon, J. H. Han, N. Park, Y. He, H. Lee, K. S. Hong, C. Kang and J. S. Kim, J. Am. Chem. Soc., 2013, 135, 11657–11662 CrossRef CAS PubMed.
Footnote |
† Electronic supplementary information (ESI) available: NMR spectra and supplementary data spectra associated with this article can be found. See DOI: 10.1039/c8ra00271a |
|
This journal is © The Royal Society of Chemistry 2018 |