DOI:
10.1039/C7RA13471A
(Paper)
RSC Adv., 2018,
8, 6910-6914
Desymmetrisation of meso-diones promoted by a highly recyclable polymer-supported chiral phosphoric acid catalyst†
Received
19th December 2017
, Accepted 4th February 2018
First published on 12th February 2018
Abstract
A polystyrene-supported BINOL-derived chiral phosphoric acid has been applied to the desymmetrisation of meso-diones to produce enantioenriched cyclohexenones. The catalytic resin has proven highly active and robust, giving rise to Hajos–Parrish or Wieland–Miescher type products in good yields and enantioselectivities, while allowing for extended recycling.
Introduction
The Robinson annulation represents one of the most powerful synthetic strategies for the synthesis of cyclohexenone derivatives,1 which are extremely versatile building blocks for the preparation of natural products. In 1971, two industrial research groups, Hajos and Parrish2 (working at Hoffmann-La Roche) and Eder, Sauer and Weichert3 (from Schering AG), independently discovered a catalytic asymmetric variant of the Robinson annulation that was mediated by proline, in what is nowadays considered one of the most important breakthroughs in organocatalysis.4 This transformation, also known as the Hajos–Parrish–Eder–Sauer–Wiechert reaction (Scheme 1, top), has emerged as a powerful tool for the total synthesis of natural products5 and bioactive compounds,6 predominantly sesquiterpenoids,7 terpenoids8 and steroids,9 which stand out for their antimicrobial, antiviral and anticancer effect (Scheme 1, bottom).
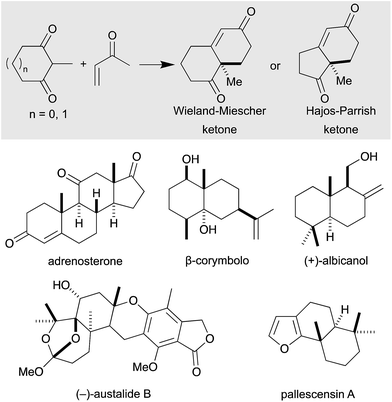 |
| Scheme 1 Wieland–Miescher and Hajos–Parrish ketones; natural products prepared via Robinson annulation. | |
Efforts to identify an alternative organocatalyst able to promote the asymmetric Robinson annulation include proline derivatives,10 amines,11 amino acids,12 peptides,13 antibodies,14 prolinamide15 or chiral vicinal diamines.16 It is thus evident that the common motif in most of the catalytic entities employed so far is the presence of a chiral amine, which is assumed to promote the reaction through the enamine activation mode.17 However, according to the commonly accepted mechanistic rationalisations, the presence of a base slows down the second half of the process, namely, the dehydration of the β-hydroxyketone intermediate. This step is accelerated in acidic media and high temperatures, which is why several protocols involve addition of an acid after the starting material is consumed. Actually, even Brønsted acids alone are known to promote the Robinson annulation in an efficient manner.1b
On the basis of these precedents, Akiyama and co-workers hypothesised that chiral phosphoric acids (CPA) should be able to mediate the desymmetrisation of meso-1,3-diones in the absence of an acidic additive; indeed, the proof of concept was reported in 2009.18 In this case, in contrast to what happens in the aminocatalytic processes, the stereoselectivity was controlled via non-covalent interactions and the same catalyst promoted the desymmetrisation and the final dehydration.
Chiral phosphoric acids were independently developed in 2004 by Akiyama and Terada19 in an attempt to harness the reactivity of imines. However, these Brønsted acid catalysts have proven to be much more versatile than initially expected, giving high levels of enantioinduction in very disparate reactions.20 Perhaps the main drawback of these CPAs is the long and tedious reaction sequences required for their preparation. In this scenario, catalyst immobilisation21 can provide a solution by rendering materials that ideally retain the properties of the monomer while being insoluble in the reaction media. With this idea in mind, several research groups, including our own, have studied the immobilisation of CPAs to assess the impact of this approach on the activity and recyclability of the resulting catalytic materials.22 Indeed, solid-supported CPAs23 turned out to be very active and robust; remarkably, in the event of loss of catalytic activity, simply washing the catalytic resin with acid restored its initial behaviour. More recently, we turned our attention to the CPA that has been more successful to date, namely, the TRIP catalyst developed by List et al.24 Thus, we prepared PS-TRIP (Fig. 1) based on a co-polymerisation strategy and applied it to the asymmetric allylboration of aldehydes.25 The catalytic resin admitted recycling in batch and the implementation of a flow process spanning 28 h. It is worth noting that, about the same time, Kobayashi et al. reported on a very similar immobilisation strategy to obtain a hybrid material that combined supported TRIP and Au/Pd nanoparticles.26 This heterogeneous catalyst exploited the benefits of both catalysts, allowing to carry out a sequence of oxidation and enantioselective aza-Friedel–Crafts reaction.
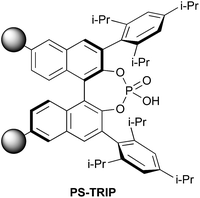 |
| Fig. 1 Polystyrene-supported TRIP phosphoric acid catalyst. | |
These previous results encouraged us to tackle the applicability of the PS-TRIP catalyst to the desymmetrisation of meso-1,3-diones to produce chiral cyclohexenones, with particular interest in the easy separation of final product and catalyst and the recovery of the latter. As previously mentioned, the acidic nature of the catalyst enables the application of the same reaction conditions to carry out both the desymmetrisation and the dehydration, without having to use any additive or modify the temperature. In contrast, previous attempts to apply solid-supported catalysts to promote this reaction have relied in aminocatalytic approaches involving proline or prolinamide derivatives supported on polystyrene,27 silica15,28 or polyethyleneglycol (Fig. 2).29 More recently, the Luo diamine catalyst16 has been supported onto polystyrene, proving to be recyclable and allowing operation in continuous flow.30
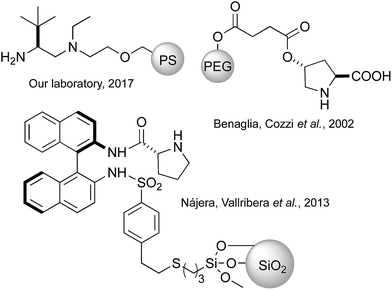 |
| Fig. 2 Selection of previously reported immobilised catalysts for the desymmetrisation of meso-diones. | |
Results and discussion
On the basis of the homogeneous precedent,18 we decided to initiate our investigation using 1a as a model substrate with our PS-TRIP catalyst in different solvents (Table 1, entries 1–5). As expected, almost no reactivity was observed at room temperature with the exception of toluene and hexane (35% yield). Heating up the reaction mixture to 70 °C effectively increased the yield while maintaining high enantioselectivities (entry 6). Increasing the catalyst loading to 10 mol%, higher yields were achieved (entry 7). After these preliminary results, we decided to expand the solvent screening (entries 8–9), but toluene and DCE did not improve the results observed with hexane. Finally, we increased the catalyst loading and the reaction time (entries 10–12), establishing the optimised reaction conditions to obtain the cyclohexenone product as follows: 20 mol% catalyst, 70 °C and 48 h (98% yield, 89% ee).
Table 1 Screening of reaction conditions for the desymmetrisation of meso-dione 1aa
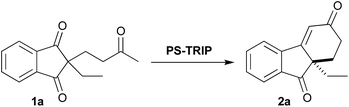
|
Entry |
Solvent |
Temp [°C] |
Cat. loading [%] |
Time [h] |
Yieldb [%] |
eec [%] |
Reactions were carried out with 0.2 mmol of 1a in 2 mL of solvent. Yield of isolated product. Determined by HPLC on a chiral stationary phase. |
1 |
Hexane |
rt |
5 |
32 |
35 |
90 |
2 |
EtOAc |
rt |
5 |
32 |
— |
— |
3 |
CH2Cl2 |
rt |
5 |
32 |
— |
— |
4 |
THF |
rt |
5 |
32 |
— |
— |
5 |
Toluene |
rt |
5 |
32 |
Traces |
— |
6 |
Hexane |
70 |
5 |
48 |
50 |
91 |
7 |
Hexane |
70 |
10 |
24 |
80 |
89 |
8 |
DCE |
70 |
10 |
24 |
64 |
90 |
9 |
Hexane |
70 |
10 |
24 |
75 |
88 |
10 |
Hexane |
70 |
15 |
24 |
64 |
88 |
11 |
Hexane |
70 |
20 |
24 |
69 |
88 |
12 |
Hexane |
70 |
20 |
48 |
98 |
89 |
With the optimised conditions in hand, we moved to explore the reaction scope, starting with the benzo-fused meso-diones (Scheme 2). The methyl-substituted analogue 2b was obtained in excellent yield and enantioselectivity (94%, 88% ee).
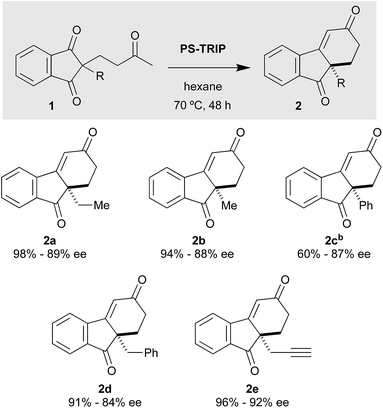 |
| Scheme 2 Scope of the desymmetrisation reaction with benzene-fused meso-diones 1.a a Unless otherwise stated, the reactions were carried out with 0.12 mmol of 1, 20 mol% of PS-TRIP in 1.2 mL of hexane. b In toluene at 90 °C. | |
The compound bearing a phenyl group gave moderate yields, which can be attributed to the low solubility of the starting material. Indeed, when the reaction was carried out in toluene at 90 °C, 2c was obtained in good yields. In comparison, the benzyl-substituted compound gave rise to the cyclohexenone 2d in considerably higher yields. As for the propargylic substrate, it displayed an excellent behaviour, furnishing 2e (an intermediate used in the synthesis of a gibbane framework2d) in excellent yield and ee. In general, we can say that this type of substrates give rise to the desired cyclohexenones in very good yields and enantioselectivities, despite the somewhat elevated reaction temperatures employed.
The results obtained with meso-diones 3, lacking the fused benzene ring, are summarised in Scheme 3; in this case the results were not as good as those recorded for the tricyclic compounds. For instance, the Hajos–Parrish 4a and Wieland–Miescher 4b ketones were synthesised in decent yields but moderate ee's. Our PS-TRIP catalyst was also able to give rise to 4c, bearing a tetrasubstituted alkene moiety, in 68% yield, but unfortunately in low enantioselectivity. Higher yield was observed in the case of the synthesis of Wieland–Miescher ketone derivative 4d, bearing the classical dimethylallyl group present in many terpene derivatives.
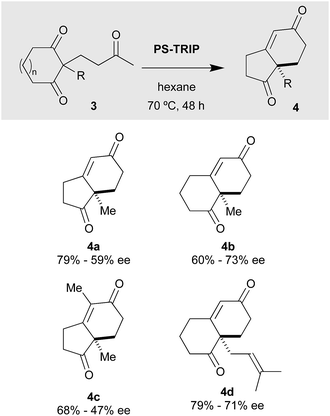 |
| Scheme 3 Scope of the desymmetrisation reaction with cyclic meso-diones 3.a a Reactions were carried out with 0.12 mmol of 3, 20 mol% of PS-TRIP in 1.2 mL of hexane. | |
The PS-TRIP resin has shown not only to be active in a wide variety of substrates, but also to be highly recyclable. Remarkably, all substrates have been run with two samples of polymer, which were already employed in the screening table. Accordingly, each sample of catalyst has been used 9 times in total without appreciable decay in activity. After each run, the catalyst was rinsed, dried in the vacuum line overnight and reused again without further reconditioning. Considering the multi-step, tedious preparation of the TRIP catalyst, this feature allows saving huge amounts of solvent and chemical waste, thus contributing to the greenness of the overall process.
Conclusions
In summary, we have established an efficient strategy for the desymmetrisation of meso-1,3-diones catalysed by an immobilised version of the TRIP phosphoric acid catalyst. This approach can be applied to a broad range of starting meso-diones, giving rise to the corresponding cyclised products in up to 98% yield and 92% enantiomeric excess. In this work, we also demonstrate the efficiency of the PS-TRIP catalyst, which is easily recovered by simple filtration and reused at least nine times, thus facilitating the isolation of the final product.
Conflicts of interest
There are no conflicts to declare.
Acknowledgements
Financial support from CERCA Programme/Generalitat de Catalunya, MINECO (CTQ2015-69136-R, AEI/MINECO/FEDER, UE, Severo Ochoa Excellence Accreditation 2014–2018, SEV-2013-0319 and FPI predoctoral grant to L. C.-A.) and AGAUR-Generalitat de Catalunya (Grant 2014SGR827) is acknowledged.
Notes and references
-
(a) W. S. Rapson and R. Robinson, J. Chem. Soc., 1935, 1285–1288 RSC
;
(b) C. H. Heathcock, J. E. Ellis, J. E. McMurry and A. Coppolino, Tetrahedron Lett., 1971, 12, 4995–4996 CrossRef
;
(c) R. E. Gawley, Synthesis, 1976, 777–794 CAS
. -
(a) Z. G. Hajos and D. R. Parrish, German patent DE 2102623, 1971
;
(b) Z. G. Hajos and D. R. Parrish, J. Org. Chem., 1974, 39, 1615–1621 CrossRef CAS
;
(c) R. A. Micheli, Z. G. Hajos, N. Cohen, D. R. Parrish, L. A. Portland, W. Sciamanna, M. A. Scott and P. A. Wehrli, J. Org. Chem., 1975, 40, 675–681 CrossRef PubMed
;
(d) S. Takano, C. Kasahara and K. Ogasawara, J. Chem. Soc., Chem. Commun., 1981, 635–637 RSC
. -
(a) U. Eder, G. R. Sauer and R. Wiechert, German patent DE 2014757, 1971
;
(b) U. Eder, G. Sauer and R. Wiechert, Angew. Chem., Int. Ed., 1971, 10, 496–497 CrossRef CAS
;
(c) J. Gutzwiller, P. Buchschacher and A. Früst, Synthesis, 1977, 167–168 CrossRef CAS
. - For a historical essay, see: C. F. Barbas, Angew. Chem., Int. Ed., 2008, 47, 42–47 CrossRef CAS PubMed
. -
(a) J. E. McMurry and S. J. Isser, J. Am. Chem. Soc., 1972, 94, 7132–7137 CrossRef CAS
;
(b) A. B. Smith and R. Mewshaw, J. Org. Chem., 1984, 49, 3685–3689 CrossRef CAS
;
(c) P. A. Grieco, J. L. Collins, E. D. Moher, T. J. Fleck and R. S. Gross, J. Am. Chem. Soc., 1993, 115, 6078–6093 CrossRef CAS
;
(d) L. A. Paquette, T.-Z. Wang and M. R. Sivik, J. Am. Chem. Soc., 1994, 116, 11323–11334 CrossRef CAS
;
(e) X.-P. Zeng, Z.-Y. Cao, Y.-H. Wang, F. Zhou and J. Zhou, Chem. Rev., 2016, 116, 7330–7396 CrossRef CAS PubMed
. -
(a) E. J. Cragoe, O. W. Woltersdorf, N. P. Gould, A. M. Pietruszkiewicz, C. Ziegler, Y. Sakurai, G. E. Stokker, P. S. Anderson and R. S. Bourke, J. Med. Chem., 1986, 29, 825–841 CrossRef CAS PubMed
;
(b) R. C. A. Isaacs, M. J. Di Grandi and S. J. Danishefsky, J. Org. Chem., 1993, 58, 3938–3941 CrossRef CAS
;
(c) S. Yamashita, K. Iso and M. Hirama, Org. Lett., 2008, 10, 3413–3415 CrossRef CAS PubMed
. -
(a) E. J. Corey, M. Ohno, R. B. Mitra and P. A. Vatakencherry, J. Am. Chem. Soc., 1964, 86, 478–485 CrossRef CAS
;
(b) H. M. C. Ferraz, A. J. C. Souza, B. S. M. Tenius and G. G. Bianco, Tetrahedron, 2006, 62, 9232–9236 CrossRef CAS
. - T. J. Reddy, G. Bordeau and L. Trimble, Org. Lett., 2006, 8, 5585–5588 CrossRef CAS PubMed
. - C. D. Dzierba, K. S. Zandi, T. Möllers and K. J. Shea, J. Am. Chem. Soc., 1996, 118, 4711–4712 CrossRef CAS
. -
(a) P. H.-Y. Cheong, K. N. Houk, J. S. Warrier and S. Hanessian, Adv. Synth. Catal., 2004, 346, 1111–1115 CrossRef CAS
;
(b) P. H.-Y. Cheong and K. N. Houk, Synthesis, 2005, 1533–1537 CAS
;
(c) E. Lacoste, E. Vaique, M. Berlande, I. Pianet, J.-M. Vincent and Y. Landais, Eur. J. Org. Chem., 2007, 167–177 CrossRef CAS
. -
(a) T. Kanger, K. Kriis, M. Laars, T. Kailas, A.-M. Müürisepp, T. Pehk and M. Lopp, J. Org. Chem., 2007, 72, 5168–5173 CrossRef CAS PubMed
;
(b) M. Laars, K. Kriis, T. Kailas, A.-M. Müürisepp, T. Pehk, T. Kanger and M. Lopp, Tetrahedron: Asymmetry, 2008, 19, 641–645 CrossRef CAS
;
(c) Á. L. Fuentes de Arriba, D. G. Seisdedos, L. Simón, V. Alcázar, C. Raposo and J. R. Morán, J. Org. Chem., 2010, 75, 8303–8306 CrossRef PubMed
. -
(a) T. Wakabayashi, K. Watanabe and Y. Kato, Synth. Commun., 1977, 7, 239–244 CrossRef CAS
;
(b) S. G. Davies, R. L. Sheppard, A. D. Smith and J. E. Thomson, Chem. Commun., 2005, 41, 3802–3804 RSC
;
(c) S. G. Davies, A. J. Russell, R. L. Sheppard, A. D. Smith and J. E. Thomson, Org. Biomol. Chem., 2007, 5, 3190–3200 RSC
. - V. D'Elia, H. Zwicknagl and O. Reiser, J. Org. Chem., 2008, 73, 3262–3265 CrossRef PubMed
. - G. Zhong, T. Hoffmann, R. A. Lerner, S. Danishefsky and C. F. Barbas, J. Am. Chem. Soc., 1997, 119, 8131–8132 CrossRef CAS
. - A. Monge-Marcet, R. Pleixats, X. Cattoen, M. W. C. Man, D. A. Alonso and C. Najera, New J. Chem., 2011, 35, 2766–2772 RSC
. -
(a) P. Zhou, L. Zhang, S. Luo and J.-P. Cheng, J. Org. Chem., 2012, 77, 2526–2530 CrossRef CAS PubMed
;
(b) C. Xu, L. Zhang, P. Zhou, S. Luo and J.-P. Cheng, Synthesis, 2013, 45, 1939–1945 CrossRef CAS
. -
(a) B. List, Acc. Chem. Res., 2004, 37, 548–557 CrossRef CAS PubMed
;
(b) S. Mukherjee, J. W. Yang, S. Hoffmann and B. List, Chem. Rev., 2007, 107, 5471–5569 CrossRef CAS PubMed
. - K. Mori, T. Katoh, T. Suzuki, T. Noji, M. Yamanaka and T. Akiyama, Angew. Chem., Int. Ed., 2009, 48, 9652–9654 CrossRef CAS PubMed
. -
(a) T. Akiyama, J. Itoh, K. Yokota and K. Fuchibe, Angew. Chem., Int. Ed., 2004, 43, 1566–1568 CrossRef CAS PubMed
;
(b) D. Uraguchi and M. Terada, J. Am. Chem. Soc., 2004, 126, 5356–5357 CrossRef CAS PubMed
. - For reviews on chiral Brønsted acid catalysis, see:
(a) T. Akiyama, J. Itoh and K. Fuchibe, Adv. Synth. Catal., 2006, 348, 999–1010 CrossRef CAS
;
(b) M. Rueping, A. Kuenkel and I. Atodiresei, Chem. Soc. Rev., 2011, 40, 4539–4549 RSC
;
(c) F. Lv, S. Liu and W. Hu, Asian J. Org. Chem., 2013, 2, 824–836 CrossRef CAS
;
(d) D. Parmar, E. Sugiono, S. Raja and M. Rueping, Chem. Rev., 2014, 114, 9047–9153 CrossRef CAS PubMed
. - For reviews on (chiral) catalyst immobilisation, see:
(a) D. E. De Vos, I. F. J. Vankelecom and P. A. Jacobs, Chiral Catalyst Immobilisation and Recycling, Wiley-VCH, Weinheim, 2000 Search PubMed
;
(b) M. Benaglia, Recoverable and Recyclable Catalysts, Wiley-VCH, Weinheim, 2009 Search PubMed
;
(c) F. Cozzi, Adv. Synth. Catal., 2006, 348, 1367–1390 CrossRef CAS
;
(d) A. Corma and H. Garcia, Adv. Synth. Catal., 2006, 348, 1391–1412 CrossRef CAS
;
(e) M. Benaglia, New J. Chem., 2006, 30, 1525–1533 RSC
;
(f) M. Gruttadauria, F. Giacalone and R. Noto, Chem. Soc. Rev., 2008, 37, 1666–1688 RSC
;
(g) A. F. Trindade, P. M. P. Gois and C. A. M. Afonso, Chem. Rev., 2009, 109, 418–514 CrossRef CAS PubMed
;
(h) J. Lu and P. H. Toy, Chem. Rev., 2009, 109, 815–838 CrossRef CAS PubMed
;
(i) T. E. Kristensen and T. Hansen, Eur. J. Org. Chem., 2010, 3179–3204 CrossRef CAS
;
(j) A. Zamboulis, N. Moitra, J. J. E. Moreau, X. Cattoën and M. Wong Chi Man, J. Mater. Chem., 2010, 20, 9322–9338 RSC
. -
(a) M. Rueping, E. Sugiono, A. Steck and T. Theissmann, Adv. Synth. Catal., 2010, 352, 281–287 CrossRef CAS
;
(b) C. Bleschke, J. Schmidt, D. S. Kundu, S. Blechert and A. Thomas, Adv. Synth. Catal., 2011, 353, 3101–3106 CrossRef CAS
;
(c) D. S. Kundu, J. Schmidt, C. Bleschke, A. Thomas and S. Blechert, Angew. Chem., Int. Ed., 2012, 51, 5456–5459 CrossRef CAS PubMed
;
(d) L. Osorio-Planes, C. Rodríguez-Escrich and M. A. Pericàs,
Chem.–Eur. J., 2014, 20, 2367–2372 CrossRef CAS PubMed
. - For reviews on solid-supported, chiral Brønsted acid catalysts, see:
(a) A. K. Mutyala and N. T. Patil, Org. Chem. Front., 2014, 1, 582–586 RSC
;
(b) C. Rodríguez-Escrich, Chim. Oggi, 2015, 33, 12–15 Search PubMed
. -
(a) S. Hoffmann, A. M. Seayad and B. List, Angew. Chem., Int. Ed., 2005, 44, 7424–7427 CrossRef CAS PubMed
;
(b) G. Adair, S. Mukherjee and B. List, Aldrichimica Acta, 2008, 41, 31–39 CAS
;
(c) M. Klussmann, L. Ratjen, S. Hoffmann, V. Wakchaure, R. Goddard and B. List, Synlett, 2010, 2189–2192 CrossRef CAS
. - L. Clot-Almenara, C. Rodríguez-Escrich, L. Osorio-Planes and M. A. Pericàs, ACS Catal., 2016, 6, 7647–7651 CrossRef CAS
. - H.-G. Cheng, J. Miguelez, H. Miyamura, W.-J. Yoo and S. Kobayashi, Chem. Sci., 2017, 8, 1356–1359 RSC
. -
(a) K. Kondo, T. Yamano and K. Takemoto, Makromol. Chem., 1985, 186, 1781–1785 CrossRef CAS
;
(b) R. Pedrosa, J. M. Andrés, R. Manzano and C. Pérez-López, Tetrahedron Lett., 2013, 54, 3101–3104 CrossRef CAS
. -
(a) A. Monge-Marcet, X. Cattoen, D. A. Alonso, C. Najera, M. W. C. Man and R. Pleixats, Green Chem., 2012, 14, 1601–1610 RSC
;
(b) A. Bañón-Caballero, G. Guillena, C. Nájera, E. Faggi, R. M. Sebastián and A. Vallribera, Tetrahedron, 2013, 69, 1307–1315 CrossRef
. - M. Benaglia, M. Cinquini, F. Cozzi, A. Puglisi and G. Celentano, Adv. Synth. Catal., 2002, 344, 533–542 CrossRef CAS
. - S. Cañellas, C. Ayats, A. H. Henseler and M. A. Pericàs, ACS Catal., 2017, 7, 1383–1391 CrossRef
.
Footnote |
† Electronic supplementary information (ESI) available: Experimental procedures, characterisation data, NMR spectra and HPLC traces. See DOI: 10.1039/c7ra13471a |
|
This journal is © The Royal Society of Chemistry 2018 |
Click here to see how this site uses Cookies. View our privacy policy here.