DOI:
10.1039/C7RA13033C
(Paper)
RSC Adv., 2018,
8, 4362-4371
Total phenylethanoid glycosides and magnoloside Ia from Magnolia officinalis var. biloba fruits inhibit ultraviolet B-induced phototoxicity and inflammation through MAPK/NF-κB signaling pathways
Received
4th December 2017
, Accepted 13th January 2018
First published on 24th January 2018
Abstract
Magnolia officinalis var. biloba is used as a traditional medicine in China and as a food additive in the United Kingdom and the European Union. In this study, total phenylethanoid glycosides (TPG) and magnoloside Ia (MIa) from M. officinalis var. biloba fruits showed excellent radical scavenging activities and potent inhibition activities against ultraviolet B (UVB)-induced oxidative damage in vitro. In vivo, TPG and MIa inhibited UVB-induced skin phototoxicity in mice upon continuous irradiation for 10 days. Changes in the levels of malondialdehyde, catalase, glutathione peroxidase, superoxide dismutase, and hydroxyproline caused by UVB irradiation were remarkably reversed in a dose-dependent manner after treatment with TPG or MIa. Protein-level analysis further showed that compared with the UVB group, the TPG high-dose group or MIa group significantly down-regulated MAPK/NF-κB signaling pathways. Therefore, TPG and MIa possessed a powerful photoprotective property that can be applied in food and antiphototoxicity formulations.
Introduction
Repeated ultraviolet B (UVB) (wavelength range: 280–320 nm) exposure can initiate a photooxidative reaction that destroys the antioxidant status of an organism. Moreover, it can trigger some reactive oxygen species (ROS)-dominant signaling pathways, thus increasing the ROS level in the cell.1,2 The oxidative stress caused by repeated UVB exposure can make toxic products more stable; then these toxic products can cause injury to skin tissues.3 More seriously, repeated UVB exposure can cause lipid peroxidation (LPO) and produce toxic products that can speed up further oxidative injury.4 The significant damage of UVB exposure primarily has various adverse effects on human skin, including erythemas, edemas, sunburn, hyperplastic responses, hyperpigmentation, photoaging, and immune suppression.5,6 Repeated UVB exposure also causes acute skin inflammation and even skin carcinogenesis.7 Therefore, suitable and efficient formulations to alleviate UVB-induced phototoxicity are necessary.
Magnolia officinalis var. biloba is a kind of deciduous tree in the Magnoliaceae family, distributed over the mountains and valleys of China at altitudes of 300–1500 m.8,9 The barks of M. officinalis var. biloba, “Cortex Magnoliae Officinalis” in Latin and “Houpo” in Chinese,8 are frequently used as a medicinal herb in China and as a food additive in gum and mints in the United Kingdom and European Union.10,11 Our previous research showed that M. officinalis var. biloba fruits extract is rich in phenylethanoid glycosides and the isolated phenylethanoid glycosides had significant antioxidant properties.10 Literature showed that phenylethanoid glycosides are found mainly in Orobanchaceae plants12,13 and possess different pharmacological properties, including anti-inflammatory, antioxidant, antitumor, antiviral, antibacterial, immunomodulatory, and neuroprotective effects.14–21 Protective effects against UVB-induced phototoxicity are related to plant extracts which are rich in antioxidants.22,23 The purpose of this study is to assess the inhibitory activity against UVB-induced phototoxicity and its underlying mechanisms of total phenylethanoid glycosides (TPG) and magnoloside Ia (MIa) from M. officinalis var. biloba fruits.
Materials and methods
Plant material
Fruits were picked from the forests of Enshi (Hubei, China; 30°17′51.56′′N, 109°28′27.33′′E, A: 1545 m). M. officinalis var. biloba was authenticated by the corresponding author and deposited as a voucher specimen (no. 14610) at the Traditional Chinese Medicine Specimen Museum, School of Pharmaceutical Science, Wuhan University, China.
Reagents
Diagnostic kits for malondialdehyde (MDA), superoxide dismutase (SOD), glutathione peroxidase (GPx), hydroxyproline (Hyp), and catalase (CAT) were bought from Nanjing Jiancheng Technology Co., Ltd. (Nanjing, China). The antibodies of β-actin, JNK, p-JNK, IκBα, and p-65 were obtained from Santa Cruz Biotechnology, Inc. (Santa Cruz, CA, USA). COX-2, ERK, p-ERK, and IKKα antibodies were purchased from Cell Signaling Technology (Beverly, MA, USA). The antibodies of p38 and p-p38 were bought from Abcam (Cambridge, UK). Gallic acid (GA), butylated hydroxytoluene (BHT), ascorbic acid (Vc), and other reagents were bought from Sinopharm Chemical Reagent Co., Ltd. (Shanghai, China).
Experimental animals
The animal experiments were approved by the Institutional Animal Ethical Committee, the Committee for the Purpose of Control, and Supervision of Experiments on Animals at Wuhan University (Wuhan, China). Female Sprague Dawley (SD) rats (200 ± 20 g, n = 10) and male BALB/c mice (20 ± 2 g, n = 56) were bought from the Laboratory Animal Center of Wuhan University, Wuhan, China. All rats and all mice were maintained on a cycle of 12 h light and 12 h dark with a relative humidity of 30–60% at 25 °C ± 2 °C. Standard pellet diets and unlimited purified water were provided for these animals. Animals were acclimatized to the surrounding environment for one week. All experimental procedures conformed to the Regulations for the Administration of Affairs Concerning Experimental Animals of China.
Preparation of TPG and MIa
TPG were prepared using a previously published method.10 In brief, the powder of dried M. officinalis var. biloba fruits was extracted with 70% alcohol (solvent/fruit ratio of 10
:
1, v/w) for 3 h. The alcohol extracts were suspended in pure water and then partitioned with chloroform, ethyl acetate, and n-butanol. Based on the pre-experiment, the n-butanol fraction (63.4 g) was rich in phenylethanoid glycoside, so it was named TPG. The TPG yield was 1.2% relative to the dry raw material. MIa was prepared on a preparative HPLC system using our described method,10 and its purity was more than 98%.
HPLC analysis of TPG
The HPLC analysis of TPG was performed on a Shimadzu LC-20AT system (Shimadzu, Tokyo, Japan), and it was furnished with an SPD-20A UV detector and a binary solvent manager. Diamonsil C18 analytical column (5 μm, 250 mm × 4.6 mm, Dikma Technologies, Beijing, China) was used. Acetonitrile (A) and 0.1% formic acid water (B) were selected as mobile phase. The gradient started from 10% A and altered to 15% A after 10 min, 20% A after 15 min, 30% A after 10 min, and 35% after 5 min. The temperature of the column oven was 25 °C, and 20 μL was injected into the system. The flow rate was 1.0 mL min−1, and UV spectra were collected at 294 nm.
Determination of phenylethanoid glycoside content in TPG
The total phenylethanoid glycoside content in TPG was measured using a method in a previous study.24 In short, using a detection wavelength of 330 nm and MIa as a standard, UV spectrophotometry was used to determine phenylethanoid glycoside content in TPG. Experimental results were showed in mg of MIa equivalents per g of dry extract.
Free radical scavenging assays
The abilities of test samples to scavenge ABTS radical cation (ABTS˙+) and DPPH were determined as previously described.22,25 Radical scavenging activities were expressed in the value of half maximal inhibitory concentration (IC50). The capacity to scavenge superoxide anion radicals (O2−˙) was tested by a pyrogallol auto-oxidation system.26 The rate constant (Kb) of automatic oxidation reaction was computed based on the curve of A325 nm versus time. Vc and BHT were used as positive controls.
In vitro protective activities
Separation of mitochondria. The mitochondria were prepared from SD rats as previously published.22 Briefly, rat liver was isolated from SD rats and homogenized in 0.25 M sucrose solutions containing 1 mM EDTA. All homogenates were centrifuged at 3000 × g for 10 min first and then at 10
000 × g for 10 min. The first centrifugation was designed to remove cell debris, whereas the purpose of the second centrifugation was to get sediment mitochondria. All procedures were carried out at 4 °C.
Experimental groups and UVB irradiation. In the reaction system, 0.5 mL mitochondrial protein and 0.1 mL of different TPG concentrations (10, 50 and 100 μg mL−1 in 0.05 M phosphate buffer, pH 7.4) were added. The concentration of MIa groups were designated according to their percentage in the corresponding TPG groups. BHT and GA were the positive control groups, whereas 0.1 mL phosphate buffer was added to the model group but without test sample. The model, BHT, GA, and test compounds groups were placed in an orifice plate at 37 °C and exposed to radiation for 4 h using a 20 W UVB lamp (TL/12RS, Philips). The distance of the lamp to the orifice plate was 15 cm. The blank control had the same ingredients as the model group, but it was not exposed to radiation. The irradiation dose was detected to be 0.88 J cm−2 by a UV irradiance meter (UV-340B, Sampo Scientific Instrument Co. Ltd., Shenzhen).
Measurement of MDA and lipid hydroperoxide (LOOH). The level of UVB-induced MDA in the mitochondria from rat liver was tested by measuring the amount of thiobarbituric acid reactive substances using a previously published thiobarbituric acid method.1 While a previous approach was adjusted to measure the level of LOOH,22,27 the prevention percentage of MDA and LOOH was computed through the following formula: prevention percentage = [(Am − As)/(Am − Ab)] × 100. In this case, Am was the absorbance of the model group, As was the absorbance of the sample groups treated with TPG, MIa, GA, and BHT, and Ab was the absorbance of the blank control group.
In vivo protective activities
Depilation treatment of test animals. For three days before the start of the UVB irradiation experiment, the back furs of all BALB/C mice were clipped by electric shaver. Moreover, the back skin (9 cm2) were thoroughly dehaired using 8% sodium sulfide.28,29
Experimental groups and UVB radiation. The BALB/C mice were randomly separated into seven groups: control group, model group, GA group (200 mg kg−1), TPG low-dose group (50 mg kg−1), TPG medium-dose group (100 mg kg−1 body), TPG high-dose group (200 mg kg−1), and MIa group (110 mg kg−1). Each group contained eight mice. The mice in the control group and model group were treated with the same volume of solvent (0.1% carboxymethylcellulose-Na). All mouse groups, besides the control group, received the same radiation. The radiation height from the 20 W UVB lamp to the back of the mice was set at 15 cm. The UVB irradiation experiment was set for 1 h daily for the first 5 days (irradiation dose was 0.22 J cm−2); another 5 days was set for 2 h daily (irradiation dose was 0.44 J cm−2).22 All mice received their corresponding group solvent on their back skin for 30 min before UVB exposure.
Measurement of MDA, CAT, GPx, Hyp, and SOD. The skin samples of the mice under irradiation were collected for further analysis. The homogenized skin samples (1
:
9, w/v) were placed in 0 °C saline solution and centrifuged for 15 min at 6000 × g at 4 °C. Then, the supernatants were collected and used to analyze the levels of MDA, CAT, GPx, Hyp, and SOD using their corresponding reagent kit.
Histopathological analysis. All skin samples were fixed in 10% neutral buffered formalin. Then, they were embedded in paraffin and sliced into 5 μm-thick sections. Hematoxylin and eosin dye (H&E) was used to stain the sections, and a microscope was used to observe the histopathological changes of these sections.
Immunoblotting analysis. All mouse skin samples were homogenized (1
:
9, w/v) in 0 °C radio immunoprecipitation assay (RIPA) lysis buffer containing a protease inhibitor. Each homogenate was centrifuged at 12
000 × g for 10 min at 4 °C, and the supernatants (total protein) were gathered and reserved at −80 °C. Nuclear proteins were isolated using the cytoplasmic and nuclear extraction kit (Wuhan Goodbio Technology Co., Ltd., Wuhan, China). The proteins were isolated by SDS-PAGE and then transferred to a polyvinylidene fluoride membrane. The membrane was then blocked with 5% nonfat milk for one hour in TBS buffer and incubated with corresponding primary antibodies. Results were measured against enhanced chemiluminescence plus western blot (WB) detection reagents.
Statistical analysis
Experimental data were presented as means ± SD in triplicate. Statistical significance was analyzed by least significant difference post hoc testing with SPSS 20.0, and p < 0.05 was regarded significant.
Results and discussion
HPLC analysis
The main chemical components in TPG were detected at 294 nm by HPLC (Fig. 1) and identified by previous studies.10,30 They were syringin (1), magnoloside IIa (2), magnoloside IIb (3), MIa (4), magnoloside IIIa (5), magnoloside IVa (6), magnoloside Ic (7), crassifolioside (8), magnoloside Va (9), and magnoloside Ib (10). They were typical phenylethanoid glycosides. We concluded that phenylethanoid glycosides are the main active compounds in TPG based on the results of HPLC analysis.
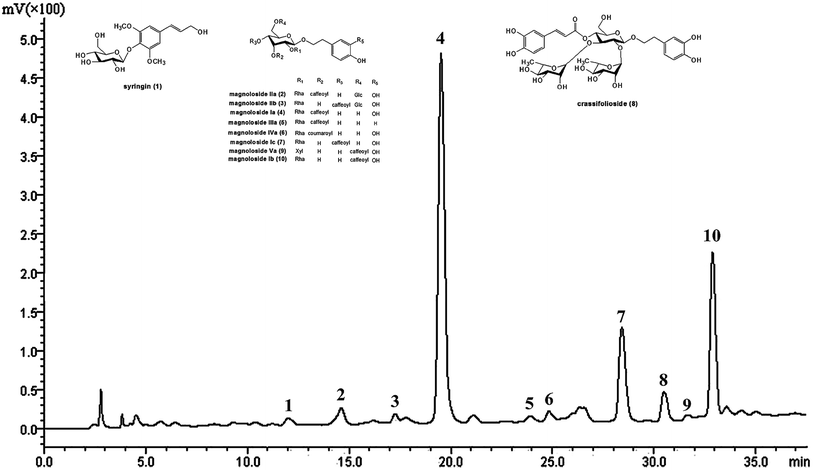 |
| Fig. 1 HPLC chromatogram of TPG at 294 nm. TPG, total phenylethanoid glycosides from M. officinalis var. biloba fruits. | |
The phenylethanoid glycoside content in TPG
The calibration curve of MIa showed strong linearity from 4 μg mL−1 to 24 μg mL−1 with the following regression equation: Y = 40.799X + 0.04 (R2 = 0.9999). The average recovery was 95.7% with relative standard deviation (RSD) = 2.1%. Therefore, the method was simple, accurate, and reproducible. According to the equation, the total phenylethanoid glycoside content in TPG was 549.0 ± 10.9 mg of MIa equivalents per g of dry extract. Results showed that TPG had relatively high phenylethanoid glycoside content.
Free radical scavenging abilities
DPPH, ABTS˙+, and O2−˙, the most common free radicals generated in vitro, have been extensively used for the detection of free radical scavenging ability.1,25,26 Table 1 summarizes the free radical scavenging abilities of TPG and MIa. In the DPPH assay, TPG showed strong DPPH radical scavenging ability with similar IC50 value (19.35 ± 0.42 μg mL−1) to the BHT group (19.79 ± 1.01 μg mL−1). Furthermore, MIa had better activity than TPG, with an IC50 value of 7.35 ± 0.36 μg mL−1 that was near the value (7.20 ± 0.14 μg mL−1) of the Vc group. In the ABTS˙+ assay, the IC50 of TPG and MIa was 3.43 ± 0.19 and 2.19 ± 0.07 μg mL−1, respectively. In the O2−· assay, the O2−˙ scavenging activity of MIa was weaker than that of Vc but equal to that of BHT. At the same time, TPG also showed a high O2−˙ scavenging ability. These data demonstrated that TPG and MIa can inhibit oxidative stress in vitro by scavenging DPPH, ABTS˙+, and O2−˙ free radicals.
Table 1 Free radical scavenging activities of TPG and MIaa
Compound |
IC50 (μg mL−1) |
Kb (×10−4 A s−1) |
DPPH |
ABTS |
Superoxide anion |
Values were the means ± SD (n = 3); TPG, total phenylethanoid glycosides from M. officinalis var. biloba fruits; MIa, magnoloside Ia; Vc, ascorbic acid; BHT, butylated hydroxytoluene. Significant differences (p < 0.05) between means are indicated by different letters above the histogram bars. The same letters indicate no significant difference among the different samples. |
TPG |
19.35 ± 0.42a |
3.43 ± 0.19c |
14.20 ± 0.15f |
MIa |
7.35 ± 0.36b |
2.19 ± 0.07d |
10.37 ± 0.33g |
Vc |
7.20 ± 0.14b |
2.03 ± 0.18d |
0.09 ± 0.02h |
BHT |
19.79 ± 1.01a |
1.60 ± 0.07e |
10.40 ± 0.55g |
Blank |
— |
— |
15.35 ± 0.19i |
In vitro protective activity during repeated UVB exposures
Repeated UVB exposures may lead to mitochondrial damage and LPO. MDA and LOOH are two unstable products of LPO.6,31 These toxic products cause oxidative stress in the mitochondria, accelerating further damage.4 To evaluate the protective activity of TPG against phototoxicity caused by UVB, the inhibitory activities of TPG on MDA and LOOH formation were evaluated (Fig. 2). After UVB irradiation for four hours, in comparison with those of the control group (p < 0.001), the levels of MDA and LOOH in the model group were remarkably increased. However, in the TPG groups, GA groups, and BHT groups, the MDA and LOOH levels significantly lower in all concentrations (p < 0.001) (Fig. 2A and B). Similarly, the MIa groups containing a phenylethanoid glycoside content equivalent to that of the TPG groups also had lower MDA and LOOH levels (Fig. 3). Furthermore, no significant difference was observed between the TPG groups and MIa groups with the equal content of phenylethanoid glycoside (p > 0.05), as shown in Fig. 3. This finding indicated that TPG played an effective role in preventing photosensitization-induced LPO at various stages, and phenylethanoid glycoside contributed to the protective effect of TPG against UVB-induced phototoxicity.
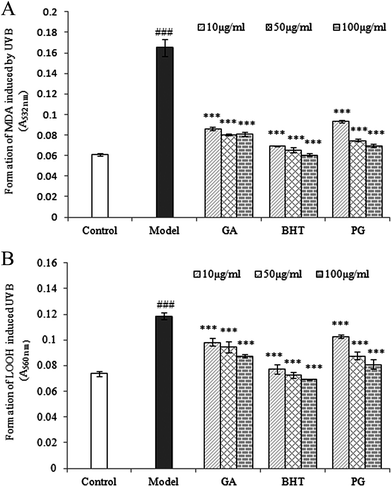 |
| Fig. 2 Effects of TPG on UVB-induced changes in MDA (A) and LOOH (B) levels; data are presented as the mean ± SD (n = 3). TPG, total phenylethanoid glycosides from M. officinalis var. biloba fruits; GA, gallic acid; BHT, butylated hydroxytoluene; MDA, malondialdehyde; LOOH, lipid hydroperoxide. Symbols represent statistical significance. ###p < 0.001, compared with the control group; ***p < 0.001, compared with the model group. | |
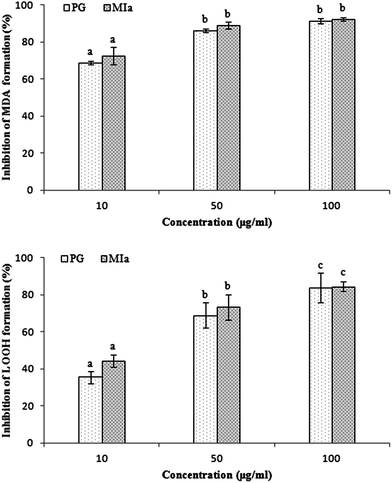 |
| Fig. 3 Comparison of protection of TPG and MIa on UVB-induced phototoxicity in mitochondria of rat liver (the total phenylethanoid glycoside content in TPG was 55%). (A) Inhibition of MDA formation; (B) inhibition of LOOH formation. Data are presented as the mean ± SD (n = 3). TPG, total phenylethanoid glycosides from M. officinalis var. biloba fruits; MIa, magnoloside Ia; MDA, malondialdehyde; LOOH, lipid hydroperoxide. Significant differences (p < 0.05) between means are indicated by different letters above the histogram bars. The same letters indicate no significant difference among the different treatments. | |
In vivo protective activity during repeated UVB exposures
MIa inhibited the loss of body weight caused by repeated UVB exposures. The body weight variations before and after the experiment are shown in Table 2. On the first day of the experiment, no significant difference was observed among the body weights of each mouse group (p > 0.05). On the eleventh day, in comparison with that of the control group (23.71 ± 0.84 g) (p < 0.001), the body weight of the UVB model group (18.75 ± 0.96 g) significantly reduced. The loss of body weight was significantly inhibited after treatment with MIa (p < 0.01). In particular, the GA group at a concentration of 200 mg kg−1 (20.07 ± 0.98 g) and MIa group at a concentration of 110 mg kg−1 (20.43 ± 1.70 g) showed significant inhibition capacity against the body weight loss caused by UVB irradiation (p < 0.05). This finding indicated that repeated UVB exposures led to loss of body weight in mice, whereas MIa efficiently suppressed such loss in mice.
Table 2 Effects of TPG and MIa on body weight in UVB-induced damage micea
Groups |
Body weight (g) |
Day 1 |
Day 11 |
Values were mean ± SD (n = 8 animals in each group). Symbols represent statistical significance. TPG, total phenylethanoid glycosides from M. officinalis var. biloba fruits; MIa, magnoloside Ia; GA, gallic acid. ###p < 0.001, compared with the control group; *p < 0.05, compared with the model group; **p < 0.01, compared with the model group. |
Control group |
19.07 ± 0.98 |
23.71 ± 0.84 |
Model group |
19.32 ± 1.11 |
18.75 ± 0.96### |
GA group 200 (mg kg−1) |
18.86 ± 1.47 |
20.07 ± 0.98* |
TPG-L group 50 (mg kg−1) |
19.06 ± 1.40 |
17.74 ± 0.93 |
TPG-M group 100 (mg kg−1) |
18.92 ± 1.95 |
19.11 ± 1.86 |
TPG-H group 200 (mg kg−1) |
19.27 ± 1.37 |
19.71 ± 1.63 |
MIa group 110 (mg kg−1) |
18.91 ± 1.86 |
20.43 ± 1.70** |
MIa inhibited skin hyperplasia caused by repeated UVB exposures. Repeated UVB exposures lead to the dilation of dermal blood vessels, leukocyte infiltration, cutaneous edema, erythema, hyperplasia, and vascular hyperpermeability.32 H&E staining (Fig. 4) revealed that the epithelial thickness of the mouse skin was definitely increased after repeated UVB exposures. Interestingly, this skin hyperplasia caused by repeated UVB exposures was improved after treatment with MIa.
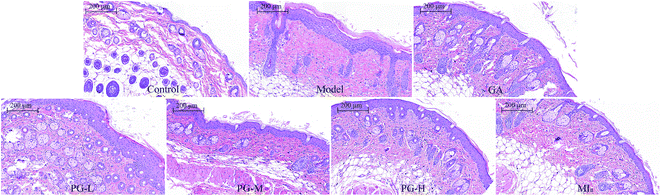 |
| Fig. 4 Sections of back skin tissue of UVB-induced damage in mice (H&E staining, ×100). TPG, total phenylethanoid glycosides from M. officinalis var. biloba fruits; MIa, magnoloside Ia; GA, gallic acid; control group; model group; GA group 200 (mg kg−1); TPG-L group 50 (mg kg−1); TPG-M group 100 (mg kg−1); TPG-H group 200 (mg kg−1); MIa group 110 (mg kg−1). | |
TPG or MIa inhibited skin oxidative damage caused by repeated UVB exposures. Repeated UVB exposures generate a lot of ROS and lead to oxidative skin damage and thus further generate oxidative stress. MDA caused by ROS is regarded as one of the most significant secondary oxidation products of oxidative stress.33 Meanwhile, antioxidant enzymes, including CAT, GPx, and SOD, are used to protect against oxidative stress and tissue damage. These enzymes play an important role in the defense against the adverse impacts caused by free radicals and ROS in biological systems.22,34–36 Many studies demonstrated that free radical scavengers have the potential to effectively lessen UVB-induced phototoxicity.37,38 The influence of TPG and MIa on the levels of MDA, CAT, GPx, SOD and Hyp in mice repeatedly exposed to UVB was evaluated in this study (Table 3). The UVB model group exhibited a remarkable decrease in their levels of CAT (14.97 ± 4.75 U g−1 protein), GPx (61.61 ± 14.35 U mg−1 protein), and SOD (205.63 ± 27.25 U mg−1 protein), together with a remarkable rise in the content of MDA (8.53 ± 0.85 nmol mg−1 protein) in compared the control group (p < 0.001). In the presence of MIa and higher doses of TPG levels of enzymes were better heightened compared to the model group. Our results clearly demonstrated that TPG or MIa possessed favorable protective effects against UVB-induced oxidative damage by increasing the levels of CAT, GPx, and SOD and by decreasing MDA formation.
Table 3 The variation of MDA, CAT, GPx, SOD and Hyp in skin tissue of mice exposed to UVB in the presence and absence of TPG and MIaa
Groups |
Skin tissue |
MDA (nmol mg−1 protein) |
CAT (U g−1 protein) |
GPx (U mg−1 protein) |
SOD (U mg−1 protein) |
Hyp (μg mg−1 protein) |
Values were mean ± SD (n = 8 animals in each group). Symbols represent statistical significance. TPG, total phenylethanoid glycosides from M. officinalis var. biloba fruits; MIa, magnoloside Ia; GA, gallic acid; MDA, malondialdehyde; CAT, catalase; GPx, glutathione peroxidase; SOD, superoxide dismutase; Hyp, hydroxyproline. ###p < 0.001 compared with the control group; *p < 0.05 compared with the model group, **p < 0.01 compared with the model group, ***p < 0.001 compared with the model group. |
Control group |
6.43 ± 0.93 |
47.67 ± 10.26 |
90.82 ± 21.60 |
368.68 ± 54.31 |
2.23 ± 0.43 |
Model group |
8.53 ± 0.85### |
14.97 ± 4.75### |
61.61 ± 14.35### |
205.63 ± 27.25### |
1.70 ± 0.25### |
GA group 200 (mg kg−1) |
5.69 ± 1.72*** |
37.80 ± 3.03*** |
88.99 ± 5.22*** |
335.68 ± 32.98*** |
2.09 ± 0.18*** |
TPG-L group 50 (mg kg−1) |
7.37 ± 0.77* |
15.51 ± 1.99 |
80.62 ± 5.56** |
220.59 ± 18.63 |
1.75 ± 0.15 |
TPG-M group 100 (mg kg−1) |
6.98 ± 1.20** |
24.74 ± 3.81*** |
90.10 ± 5.92*** |
281.48 ± 18.34*** |
1.83 ± 0.12 |
TPG-H group 200 (mg kg−1) |
6.48 ± 0.62*** |
38.75 ± 3.81*** |
93.43 ± 6.50*** |
316.10 ± 33.87*** |
1.97 ± 0.14* |
MIa group 110 (mg kg−1) |
6.21 ± 1.18*** |
41.12 ± 5.70*** |
95.82 ± 12.93*** |
332.29 ± 30.41*** |
2.07 ± 0.15** |
The level of Hyp can be measured to estimate the level of collagen. Collagen is one of the most crucial structural proteins of the skin. The reduction in the content of Hyp is seen as wrinkle formation in photoaged skin.28 As shown in Table 3, in comparison with that of the control group (p < 0.001), the model group showed a remarkable decrease in Hyp level (1.70 ± 0.25 μg mg−1 protein), and the change was remarkably reversed when the mice were treated with GA (200 mg kg−1), TPG (200 mg kg−1), or MIa (110 mg kg−1) (p < 0.05). Our results demonstrated that treatment with TPG or MIa effectively prevented wrinkle formation caused by repeated UVB exposures.
TPG or MIa inhibited inflammation caused by repeated UVB exposures. ROS is generated by UVB irradiation in a direct and straightforward way. Moreover, the body's antioxidant defense system is depleted, and an aggrandized oxidative damage emerges due to the effect of the inflammatory response.39 ROS is responsible for many diversified oxidative-stress-related diseases as well as inflammation, including skin cancer and aging.40 Reduplicated UVB exposures brings about the overexpression of proinflammatory enzymes iNOS and COX-2 and cytokines TNF-α and IL-6, as mentioned in previous studies.32,41 COX-2 plays an important role in UVB-induced inflammation because it catalyzes the production of PGE2 from prostanoid precursors.42 Furthermore, a few animal models on inflammation have verified the overexpression of COX-2.43,44 The influence of TPG and MIa on the UVB-induced expression of COX-2 protein in skin tissue was assessed during this study. WB analysis revealed that repeated UVB exposures led to a remarkable increase in the overexpression of COX-2 protein in the control group (Fig. 5A). However, the expression of COX-2 protein in TPG or MIa groups was decreased in contrast with that of mice whose skin was exposed to UVB irradiation alone. Therefore, we concluded that TPG or MIa inhibited inflammation by reducing the level of COX-2 protein.
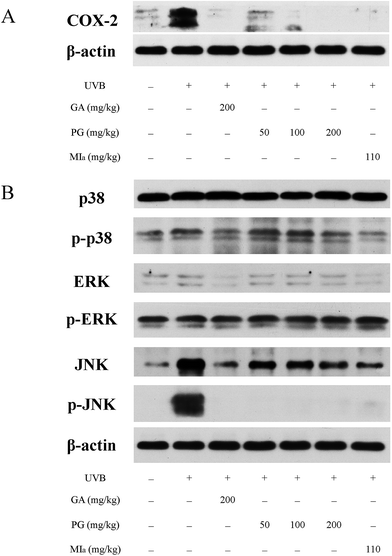 |
| Fig. 5 Effects of TPG and MIa on protein expressions of COX-2 (A) and MAPKs (B) in the back-skin tissue of mice with UVB-induced damage. TPG, total phenylethanoid glycosides from M. officinalis var. biloba fruits; MIa, magnoloside Ia; GA, gallic acid. | |
TPG or MIa inhibited the phosphorylation of MAPK protein caused by repeated UVB exposures. MAPK proteins are made up of three family members, including Jun N-terminal kinases (JNK), p38 kinase, and extracellular signal-regulated protein kinase (ERK).45,46 Oxidative stress caused by UVB irradiation plays an important role in the activation of phosphorylated MAPK protein, including ERK, JNK, and p38. These phosphorylated-MAPK (p-MAPK) proteins are involved in phototoxicity.47,48 Furthermore, MAPK and p-MAPK proteins are proven to modulate NF-κB activation.32 In this study, we also analyzed the MAPK and p-MAPK expressions upon treatment with TPG and MIa. As shown in Fig. 5B, WB analysis elucidated that repeated UVB exposures to mouse skin led to increasing levels of phosphorylation of ERK, JNK, and p38. Meanwhile, TPG high-dose group and MIa groups inhibited the activation of p-p38 and p-JNK. This study demonstrated that TPG or MIa inhibited the UVB-induced activation of MAPK and p-MAPK on mouse skin, thereby reducing the risk of phototoxicity.
TPG or MIa inhibited the activation of NF-κB pathway caused by repeated UVB exposures. Repeated UVB exposures lead to the activation of NF-κB pathway,49 and p65 is the downstream target of this pathway.50 UVB irradiation triggers the activation of IKK, which causes the phosphorylation of serine residues in IκBα and then leads to the degradation of IκBα protein, followed by translocation and activation of p65 to the nucleus.51,52 The activation of NF-κB pathway caused by repeated UVB exposures stimulates inflammatory cytokine expressions that contribute to skin inflammation.49 As shown in Fig. 6A, the cytoplasmic levels of activated IKKα were lower in the TPG high-dose group and MIa group in comparison with those of the UVB model group, and the cytoplasmic levels of IκBα protein were the opposite. At the same time, our results also indicated that TPG markedly reduced UVB-induced p65 activation, and MIa also had such inhibition effect (Fig. 6B). To sum up, we concluded that TPG and MIa inhibited the nuclear translocation of UVB-induced p65 protein by preventing the activation of IKKα and the succeeding degradation of IκBα protein in mouse skin.
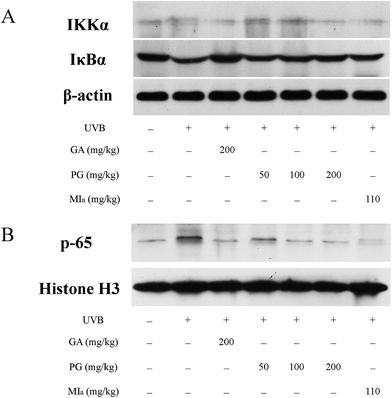 |
| Fig. 6 Effects of TPG and MIa on protein expressions of IKKα, IκBα (A) and p65 (B) in the back-skin tissue of mice with UVB-induced damage. TPG, total phenylethanoid glycosides from M. officinalis var. biloba fruits; MIa, magnoloside Ia; GA, gallic acid. | |
Conclusion
In conclusion, TPG and MIa efficiently scavenged free radicals and prevented the UVB-induced LPO at its various stages in vitro. MIa treated mice had less skin thickening, lower MDA levels, maintained better GPx, CAT, SOD, and Hyp levels, and increased body weights indicating enhanced protection against UVB-induced phototoxicity. Furthermore, TPG and MIa also prevented inflammation by inhibiting the activation of MAPK/NF-κB signaling pathways. The proposed mechanism involved the reduction of p-MAPK levels and dephosphorylation of serine residues in IκBα by IKK, thereby resulting in the deactivation of NF-κB and COX-2 (Fig. 7). Furthermore, our experimental results also demonstrated that the protective effect of TPG against UVB-induced phototoxicity was generally associated with the presence of phenylethanoid glycoside. Based on this study, we suggested that TPG and MIa can be used as photoprotective agents in food products due to their free radical scavenging and anti-inflammatory activities. This study is expected to provide additional value to M. officinalis var. biloba fruits and may be helpful for its applications in the scientific field.
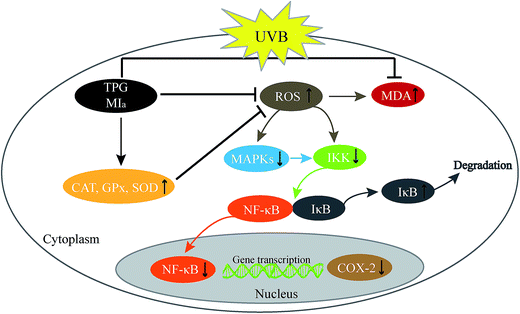 |
| Fig. 7 Proposed mechanism of photoprotection by TPG and MIa. TPG, total phenylethanoid glycosides from M. officinalis var. biloba fruits; MIa, magnoloside Ia. | |
Conflicts of interest
The authors declare no competing financial interest.
Acknowledgements
This work was supported by the Project of the National Twelve-Five Year Research Program of China (2011BAI06B06; 2012BAI29B03), the Commonweal Specialized Research Fund of China Agriculture (201103016), and the Nanjing 321 Plan for Bringing in Technological Leading Talents (2013A12011).
References
- B. X. Ma, L. Zhu, X. Y. Zang, Y. X Chen, D. Li and Y. W. Wang, Coptis chinensis inflorescence and its main alkaloids protect against ultraviolet-B-induced oxidative damage, J. Funct. Foods, 2013, 5, 1665–1672 CrossRef CAS.
- V. A. Trabosh, A. Daher, K. A. Divito, K. Amin, C. M. Simbulan-Rosenthal and D. S. Rosenthal, UVB upregulates the bax promoter in immortalized human keratinocytes via ROS induction of Id3, Exp. Dermatol., 2009, 18, 387–395 CrossRef CAS PubMed.
- M. Abu Zaid, F. Afaq, D. N. Syed, M. Dreher and H. Mukhtar, Inhibition of UVB mediated oxidative stress and markers of photoaging in immortalized HaCaT keratinocytes by pomegranate polyphenol extract POMx, Photochem. Photobiol., 2007, 83, 882–888 CrossRef CAS PubMed.
- L. Zhu, B. Huang, X. Q. Ban, J. S. He, Y. X. Chen, L. Han and Y. W. Wang, Coptis chinensis inflorescence extract protection against ultraviolet-B-induced phototoxicity, and HPLC-MS analysis of its chemical composition, Food Chem. Toxicol., 2012, 50, 2584–2588 CrossRef CAS PubMed.
- S. Choi, S. K. Lee, J. E. Kim, M. H. Chung and Y. I. Park, Aloesin inhibits hyperpigmentation induced by UV radiation, Clin. Exp. Dermatol., 2002, 27, 513–515 CrossRef CAS PubMed.
- H. M. Park, E. Moon, A. J. Kim, M. H. Kim, S. Lee, J. B. Lee, Y. K. Park, H. S. Jung, Y. B. Kim and S. Y. Kim, Extract of Punica granatum inhibits skin photoaging induced by UVB irradiation, Int. J. Dermatol., 2010, 49, 276–282 CrossRef CAS PubMed.
- Y. Sano and J. M. Park, Loss of epidermal p38α signaling prevents ultraviolet radiation-induced inflammation via acute and chronic mechanisms, J. Invest. Dermatol., 2014, 134, 2231–2240 CrossRef CAS PubMed.
- Chinese Pharmacopoeia Commission, Pharmacopoeia of the People's Republic China, China Medical Science Press, Beijing, China, 2015, vol. 1, pp. 251–252 Search PubMed.
- J. S. He, L. Chen, Y. Si, B. Huang, X. Q. Ban and Y. W. Wang, Population structure and genetic diversity distribution in wild and cultivated populations of the traditional Chinese medicinal plant Magnolia officinalis subsp. biloba (Magnoliaceae), Genetica, 2009, 135, 233–243 CrossRef PubMed.
- L. L. Ge, W. H. Zhang, G. Zhou, B. X. Ma, Q. G. Mo, Y. X. Chen and Y. W. Wang, Nine phenylethanoid glycosides from Magnolia officinalis var. biloba fruits and their protective effects against free radical-induced oxidative damage, Sci. Rep., 2017, 7, 45342 CrossRef CAS PubMed.
- M. Greenberg, P. Urnezis and M. Tian, Compressed mints and chewing gum containing magnolia bark extract are effective against bacteria responsible for oral malodor, J. Agric. Food Chem., 2007, 55, 9465–9469 CrossRef CAS PubMed.
- L. Li, R. Tsao, R. Yang, C. M. Liu, J. C. Young and H. H. Zhu, Isolation and purification of phenylethanoid glycosides from Cistanche deserticola by high-speed counter-current chromatography, Food Chem., 2008, 108, 702–710 CrossRef CAS PubMed.
- Y. Li, G. Zhou, Y. Peng, P. Tu and X. Li, Screening and identification of three typical phenylethanoid glycosides metabolites from Cistanches Herba by human intestinal bacteria using UPLC/Q-TOF-MS, J. Pharm. Biomed. Anal., 2016, 118, 167–176 CrossRef CAS PubMed.
- H. Bardakci, H. Skaltsa, T. Milosevic-Ifantis, D. Lazari, D. Hadjipavlou-Litina, E. Yesilada and H. Kırmızıbekmez, Antioxidant activities of several Scutellaria taxa and bioactive phytoconstituents from Scutellaria hastifolia L., Ind. Crops Prod., 2015, 77, 196–203 CrossRef CAS.
- Y. Jiang, S. Mao, W. Huang, B. Lu, Z. Cai, F. Zhou, M. Li, T. Lou and Y. Zhao, Phenylethanoid glycoside profiles and antioxidant activities of Osmanthus fragrans Lour. flowers by UPLC/PDA/MS and simulated
digestion model, J. Agric. Food Chem., 2016, 64, 2459–2466 CrossRef CAS PubMed.
- Y. L. Liu, W. J. He, L. Mo, M. F. Shi, Y. Y. Zhu, S. Pan, X. R. Li, Q. M. Xu and S. L. Yang, Antimicrobial, anti-inflammatory activities and toxicology of phenylethanoid glycosides from Monochasmasa vatieri Franch. ex Maxim., J. Ethnopharmacol., 2013, 149, 431–437 CrossRef CAS PubMed.
- Y. G. Liu, X. X. Li, D. C. Xiong, B. Yu, X. Pu and X. S. Ye, Synthetic phenylethanoid glycoside derivatives as potent neuroprotective agents, Eur. J. Med. Chem., 2015, 95, 313–323 CrossRef CAS PubMed.
- Q. G. Ma, Y. C. Guo, W. M. Liu, Z. Q. Wang, W. T. Mao, X. Zhang, L. T. Yu, Q. C. Yang and R. R. Wei, Phenylethanoid glycosides from Houttuynia cordata and their hepatoprotective activities, Chem. Nat. Compd., 2016, 52, 761–763 CrossRef CAS.
- J. N. Oyourou, S. Combrinck, T. Regnier and A. Marston, Purification, stability and antifungal activity of verbascoside from Lippia javanica and Lantana camara leaf extracts, Ind. Crops Prod., 2013, 43, 820–826 CrossRef CAS.
- X. Song, C. Y. Li, Y. Zeng, H. Q. Wu, Z. Huang, J. Zhang, R. S. Hong, X. X. Chen, L. Y. Wang, X. P. Hu, W. W. Su, Y. Li and Z. D. He, Immunomodulatory effects of crude phenylethanoid glycosides from, J. Ethnopharmacol., 2012, 144, 584–591 CrossRef CAS PubMed.
- Z. Xue and B. Yang, Phenylethanoid glycosides: Research advances in their phytochemistry, pharmacological activity and pharmacokinetics, Molecules, 2016, 21, 991–1016 CrossRef PubMed.
- B. Huang, L. Zhu, S. Liu, D. Li, Y. X. Chen, B. X. Ma and Y. W. Wang, In vitro and in vivo evaluation of inhibition activity of lotus (Nelumbo nucifera Gaertn.) leaves against ultraviolet B-induced phototoxicity, J. Photochem. Photobiol., B, 2013, 121, 1–5 CrossRef CAS PubMed.
- M. Perde-Schrepler, G. Chereches, I. Brie, C. Tatomir, I. D. Postescu, L. Soran and A. Filip, Grape seed extract as photochemopreventive agent against UVB induced skin cancer, J. Photochem. Photobiol., B, 2013, 118, 16–21 CrossRef CAS PubMed.
- L. N. Wang, J. Chen, M. H. Yang, S. L. Chen, Y. Shi, Y. Qi and T. N. Liu, Quantitative determination of total phenylethanoid glycosides in Cistanche desertiola, Northwest Pharm. J., 2008, 2, 67–69 Search PubMed.
- S. M. M. R. Mawalagedera, Z. Q. Ou, A. McDowell and K. L. Gould, Effects of boiling and in vitro gastrointestinal digestion on the antioxidant activity of Sonchus oleraceus leaves, Food Funct., 2016, 7, 1515–1522 CAS.
- G. Zhou, Y. X. Chen, S. Liu, X. C. Yao and Y. W. Wang, In vitro and in vivo hepatoprotective and antioxidant activity of ethanolic extract from Meconopsis integrifolia (Maxim.) Franch, J. Ethnopharmacol., 2013, 148, 664–670 CrossRef CAS PubMed.
- N. Z. Jaffar, T. S. Javad, B. H. Ines and P. W. Simon, Measurement of hydroperoxides in edible oils using the ferrous oxidation in xylenol orange assay, J. Agric. Food Chem., 1995, 43, 17–21 CrossRef.
- H. Hou, B. F. Li, Y. L. Zhuang, G. Y. Ren, M. Y. Yan, Y. P. Cai, X. K. Zhang and L. Chen, The effect of pacific cod (Gadus macrocephalus) skin gelatin polypeptides on UV radiation-induced skin photoaging in ICR mice, Food Chem., 2009, 115, 945–950 CrossRef CAS.
- M. Sumiyoshi and Y. Kimura, Effects of olive leaf extract and its main component oleuroepin on acute ultraviolet B irradiation-induced skin changes in C57BL/6J mice, Phytother. Res., 2010, 24, 995–1003 CAS.
- S. X. Yu, R. Y. Yan, R. X. Liang, W. Wang and B. Yang, Bioactive polar compounds from stem bark of Magnolia officinalis, Fitoterapia, 2012, 83, 356–361 CrossRef CAS PubMed.
- S. Bhattacharya, J. P. Kamat, S. K. Bandyopadhyay and S. Chattopadhyay, Comparative inhibitory properties of some Indian medicinal plant extracts against photosensitization-induced lipid damage, Food Chem., 2009, 113, 975–979 CrossRef CAS.
- S. D. Sharma and S. K. Katiyar, Dietary grape seed proanthocyanidins inhibit UVB induced cyclooxygenase-2 expression and other inflammatory mediators in UVB exposed skin and skin tumors of SKH-1 hairless mice, Pharm. Res., 2010, 27, 1092–1102 CrossRef CAS PubMed.
- N. Khan, D. N. Syed, H. C. Pal, H. Mukhtar and F. Afaq, Pomegranate fruit extract inhibits UVB-induced inflammation and proliferation by modulating NF-κB and MAPK signaling pathways in mouse skin, Photochem. Photobiol., 2012, 88, 1126–1134 CrossRef CAS PubMed.
- J. Eliza, P. Daisy and S. Ignacimuthu, Antioxidant activity of constunolide and eremanthin isolated from Costus speciosus (Koen ex Retz) Sm, Chem.-Biol. Interact., 2010, 188, 467–472 CrossRef CAS PubMed.
- F. C. Lidon and J. C. Ramalho, Impact of UV-B irradiation on photosynthetic performance and chloroplast membrane components in Oryza sativa L., J. Photochem. Photobiol., B, 2011, 104, 457–466 CrossRef CAS PubMed.
- Z. F. Skalická, F. Zölzer, L. Beránek and J. Racek, Indicators of oxidative stress after ionizing and/or non-ionizing radiation: superoxide dismutase and malondialdehyde, J. Photochem. Photobiol., B, 2012, 117, 111–114 CrossRef PubMed.
- C. W. Lee, H. H. Ko, C. Y. Chai, W. T. Chen, C. C. Lin and F. L. Yen, Effect of artocarpus communis extract on UVB irradiation-induced oxidative stress and inflammation in hairless mice, Int. J. Mol. Sci., 2013, 14, 3860–3873 CrossRef CAS PubMed.
- M. Sumiyoshi and Y. Kimura, Effects of a turmeric extract (Curcuma longa) on chronic ultraviolet B irradiation-induced skin damage in melanin-possessing hairless mice, Phytomedicine, 2009, 16, 1137–1143 CrossRef PubMed.
- A. Petrova, L. M. Davids, F. Rautenbach and J. L. Marnewick, Photoprotection by honeybush extracts, hesperidin and mangiferin against UVB-induced skin damage in SKH-1 mice, J. Photochem. Photobiol., B, 2011, 103, 126–139 CrossRef CAS PubMed.
- F. Afaq, V. M. Adhami and N. Ahmad, Prevention of short-term ultraviolet B radiation-mediated damages by resveratrol in SKH-1 hairless mice, Toxicol. Appl. Pharmacol., 2003, 186, 28–37 CrossRef CAS PubMed.
- J. A. Lee, B. G. Jung, T. H. Kim, S. G. Lee, Y. S. Park and B. J. Lee, Dietary feeding of Opuntia humifusa inhibits UVB radiation-induced carcinogenesis by reducing inflammation and proliferation in hairless mouse model, Photochem. Photobiol., 2013, 89, 1208–1215 CrossRef CAS PubMed.
- S. M. Fischer, A. Pavone, C. Mikulec, R. Langenbach and J. E. Rundhaug, Cyclooxygenase-2 expression is critical for chronic UV-induced murine skin carcinogenesis, Mol. Carcinog., 2007, 46, 363–371 CrossRef CAS PubMed.
- P. Pratheeshkumar and G. Kuttan, Cardiospermumhalicacabum inhibits cyclophosphamide induced immunosuppression and oxidative stress in mice and also regulates iNOS and COX-2 gene expression in LPS stimulated macrophages, Asian Pac J Cancer Prev., 2010, 11, 1245–1252 Search PubMed.
- P. Pratheeshkumar and G. Kuttan, Modulation of immune response by Vernonia cinerea L. inhibits the proinflammatory cytokine profile, iNOS, and COX-2 expression in LPS-stimulated macrophages, Immunopharmacol. Immunotoxicol., 2011, 33, 73–83 CrossRef CAS PubMed.
- J. G. Einspahr, B. G. Timothy, D. S. Alberts, N. McKenzie, K. Saboda and J. Warneke, Cross-validation of murine UV signal transduction pathways in human skin, Photochem. Photobiol., 2008, 84, 463–476 CrossRef CAS PubMed.
- M. Moriyama, H. Moriyama, J. Uda, H. Kudo, Y. Nakajima, A. Goto, T. Moria and T. Hayakawa, BNIP3 upregulation via stimulation of ERK and JNK activity is required for the protection of keratinocytes from UVB-induced apoptosis, Cell Death Dis., 2017, 8, e2576 CrossRef CAS PubMed.
- S. E. Dickinson, E. R. Olson, J. Zhang, S. J. Cooper, T. Melton, P. J. Criswell, A. Casanova, Z. Dong, C. Hu, K. Saboda, E. T. Jacobs, D. S. Alberts and G. T. Bowden, P38 MAP kinase plays a functional role in UVB-induced mouse skin carcinogenesis, Mol. Carcinog., 2011, 50, 469–478 CrossRef CAS PubMed.
- H. P. Li, Z. J. Li, L. Q. Peng, Q. Jiang, E. T. Zhang, B. H. Liang, R. X. Li and H. L. Zhu, Lycium barbarum polysaccharide protects human keratinocytes against UVB-induced photo-damage, Free Radical Res., 2017, 51, 200–210 CrossRef CAS PubMed.
- F. T. M. C. Vicentini, T. He, Y. Shao, M. J. V. Fonseca, W. A. Verri Jr, G. J. Fisher and J. Xu, Quercetin inhibits UV irradiation-induced inflammatory cytokine production in primary human keratinocytes by suppressing NF-κB pathway, J. Dermatol. Sci., 2011, 61, 162–168 CrossRef CAS PubMed.
- S. D. Sharma, S. M. Meeran and S. K. Katiyar, Dietary grape seed proanthocyanidins inhibit UVB-induced oxidative stress and activation of mitogen-activated protein kinases and nuclear factor-κB signaling in vivo SKH-1 hairless mice, Mol. Cancer Ther., 2007, 6, 995–1005 CrossRef CAS PubMed.
- S. K. Mantena and S. K. Katiyar, Grape seed proanthocyanidins inhibit UV-radiation induced oxidative stress and activation of MAPK and NF-κB signaling in human epidermal keratinocytes, Free Radicals Biol. Med., 2006, 40, 1603–1614 CrossRef CAS PubMed.
- P. Pratheeshkumar and G. Kuttan, Vernolide-A, a sesquiterpene lactone from Vernonia cinerea, induces apoptosis in B16F-10 melanoma cells by modulating p53 and caspase-3 gene expressions and regulating NF-κB-mediated Bcl-2 activation, Drug Chem. Toxicol., 2011, 34, 261–270 CrossRef CAS PubMed.
|
This journal is © The Royal Society of Chemistry 2018 |
Click here to see how this site uses Cookies. View our privacy policy here.