DOI:
10.1039/C7RA12927K
(Paper)
RSC Adv., 2018,
8, 5536-5541
An azo-coupling reaction-based surface enhanced resonance Raman scattering approach for ultrasensitive detection of salbutamol†
Received
1st December 2017
, Accepted 11th January 2018
First published on 1st February 2018
Abstract
To date, great achievements with GC-MS, HPLC-MS, and fluorescence biosensing techniques have been made to detect illegal additives of salbutamol (SAL) in swine meat. However, these methods are not suitable for rapid on-site screening due to either costly instruments or rather complicated and/or time consuming sample pretreatments. Herein, a simple, rapid and ultrasensitive approach based on an azo-coupling reaction and surface-enhanced resonance Raman scattering (SERRS) is presented. By combining with a magnetic SERS substrate, an indirect detection for SAL, with a LOD of 1.0 × 10−11 M (2.39 pg mL−1), was realized. Moreover, a colorimetric method for naked eye detection was successfully carried out for rapid screening of SAL in concentrations higher than 2.09 × 10−5 M (5 μg mL−1). In addition, the proposed method was successfully applied for the rapid determination of SAL in real swine meat. The entire process, including pretreatment, coupling reaction and SERRS detection, was performed within 7 min. Moreover, the SERRS fingerprint band being specific to corresponding functional group guarantees the selectivity for the target molecule. Therefore, the proposed strategy in the present study offers a new way to identify trace amounts of analytes, such as SAL as well as other illegal additives in health-related products and food.
Introduction
Salbutamol (SAL) is often used not only as a drug to treat bronchial asthma and smooth muscle but also a type of β-adrenergic agonist to promote protein synthesis in animal body. It can accelerate the transformation and the decomposition of fat,1–3 and is therefore often added to animal feed and easily accumulates in animal body as a residue. Upon consuming animal derived food, humans may suffer from intensified breathing, headache, chest tightness, heart palpitations, increased heart rate, muscle tremors, nausea and other symptoms of poisoning.4,5 Therefore, according to the Bulletin 235 issued by the Ministry of Agriculture of China (MAC), SAL is a strictly prohibited substance and should not be detected in animal meat.6–8
To date, great efforts have been made to detect SAL with high sensitivities. For this, gas chromatography-mass spectrometry (GC-MS),9 liquid chromatography mass spectrometry (LC-MS),6,10 ultra-high-performance liquid chromatography (UHPLC) coupled to quadrupole-linear ion trap tandem mass spectrometry,11 surface molecularly imprinted polymers,12 electro-chemical analysis,13 capillary electrophoresis,14 immunochromatographic assay (ICA),15 fluorescence biosensor16,17 etc. have been reported. However, these methods need either costly instruments or rather complicated, and/or time consuming sample pretreatments. Therefore, it is urgently needed to develop a simple, rapid and ultrasensitive approach for SAL detection.
Surface enhanced Raman scattering (SERS) is a fascinating technique where the Raman signal of the analyte absorbed on a rough metallic surface can be amplified tremendously even for single molecule detection.18,19 SERS has gained a lot of attention on account of its non-destructivity, high selectivity, ultra-sensitivity, reliability and fast operation in testing chemical and/or biological molecules in trace amounts.20,21 For SERS-based SAL detection, a principal challenge is the low binding affinity of these phenolic molecules on silver or gold surfaces,22,23 resulting in poor SERS signals when the analytes are mixed with SERS-active nanoparticles directly (Fig. S1†). To date, several indirect methods such as lateral flow immunochromatographic assay,20 competitive immunochromatographic test24 and competitive immunoassay,25 combined with highly sensitive SERS have been developed to detect SAL. However, these immunochemical methods require complicated preparation of antibody, are costly, and especially, the determination conditions are highly strict. Particularly, when the concentration of SAL is very low, even false results may be obtained, thus greatly restraining their practical application. Therefore, developing a new technique for sample pretreatment that allows cheap, simple, rapid, selective, and sensitive modification on SAL for SERS detection, is pivotal and a great challenge.
Pauly's reaction is also known as the azo-coupling reaction, where the diazonium ion couples with either a phenol, amine or hetero-cyclic compound, finally producing azo dyes.26 Pauly's reagent has been reported to be used for the determination of imidazole or phenol-containing molecules,27 based on the optical absorption of the azo products. Their propensity toward binding to silver nanoparticles (AgNPs) as substrates makes it possible to supply a strong Raman signal for the analytes.28 Therefore, by combining with the surface-enhanced resonance Raman scattering (SERRS) technique,29,30 we will propose an azo-coupling reaction-based SERRS approach for SAL determination. In comparison to the antibody-based immunoprobe, this method will avoid the use of expensive biological reagents and a tedious and time-consuming process for sample pretreatment. By using just a simple chemical reaction with color change, SAL measurement in high sensitivity and selectivity can be made possible. Moreover, in order to improve the test efficiency, magnetic nanoparticles (Fe3O4@Ag, Fig. S2†) were adopted to enrich the response to the target molecule, thus avoiding time-consuming centrifugation repeatedly. Finally, the proposed method is successfully applied to detect SAL in real samples within extremely short times, and provides a rapid and ultra-sensitive identification approach to detect illegal additives in meat products.
Experimental section
Materials
Ethyleneglycol, anhydrous ethanol, sodium acetate anhydrous, iron(III) chloride hexahydrate, tetraethyl orthosilicate (TEOS), concentrated ammonium aqueous solution (25 wt%), sodium citrate, silver nitrate, sodium nitrite, hydrochloric acid, sodium carbonate, acetonitrile, potassium chloride and sodium chloride were purchased from the Sinopharm Chemical Reagent Co., Ltd. (Shanghai, China). Salbutamol (SAL), sulfanilic acid, 2,2′-benzidinedisulfonic acid, clenbuterol hydrochloride, L-lysine monohydrate, aminoacetic acid, glycerol and phosphate-buffered saline (PBS, pH 7.4) contained NaCl (100 mM) and 10 mM Na2HPO4/KH2PO4, were bought from Aladdin Reagent Co. Ltd. (Shanghai, China). Deionized water (Millipore) with a resistivity of 18 MΩ cm was used in all experiments.
2.39 mg SAL was dissolved in 10 mL methanol (1.0 × 10−3 M) and kept in the dark at 4 °C as stock solution. Then its standard solution was obtained by diluting the stock solution to the desired concentration with distilled water.
Preparation of Fe3O4@Ag particles
The microspheres of Fe3O4 were firstly synthesized following a reported method.31 The silver nanoparticles (AgNPs, ∼80 nm) were prepared according to the synthesis method reported by Lee and Meisel,32 which is summarized as follows: 27 mg AgNO3 was dissolved in 150 mL ultrapure water. After being heated to boiling temperature, sodium citrate (1%, 3 mL) was quickly added into the solution, and then it was kept boiling for 40 min under reflux condition to get a thorough reaction. After free cooling to room temperature, the obtained AgNPs were ready to be assembled on the surface of Fe3O4 microspheres.
For that, the as-prepared Fe3O4 microspheres (50 mg) should be mixed firstly with polyethyleneimine (PEI) (100 mL, 5.0 mg mL−1) to obtain surface modification with amino groups. Then the freshly prepared AgNPs were mixed with the amino-functionalized Fe3O4 to produce Fe3O4@Ag via electrostatic interaction.33
Pauly reagent used for SAL
The azo-reaction was performed between the modified Pauly's reagent (diazonium salt) and SAL. Different species of aromatic amines, including (1) sulfanilic acid and (2) 2,2′-benzidinedisulfonic acid (20 mM), were used to investigate and optimize the absorption of SAL-derivatives, finally to produce a better resonance Raman signal.
Optimization on the excitation wavelength for Raman resonance
The laser light at 514, 532, 633 and 785 nm was used, respectively, as an excitation wavelength for the detection of azo dye. The final purpose was to give the strongest SERRS signal for SAL detection.
Azo-coupling reaction
Reagents A, B and C were prepared as follows, respectively, and then stored at 4 °C ready for use. A mixture of 20 mM 2,2′-benzidinedisulfonic acid and 12 M HCl (0.1 mL) in 10 mL solution was used for reagent A; reagent B was 0.8% NaNO2, and reagent C was 4% Na2CO3 in water. The coupling reaction was performed by mixing reagents A, B, C, and SAL together. For that, reagent B was first added into reagent A, and then the sample and reagent C were rapidly added into with manual vortex reaction for 1 min at room temperature. After that, the obtained solution was mixed with a solution containing Fe3O4@Ag (50 mg mL−1, 20 μL) for Raman spectral measurement.
SERRS detection for real samples
The process of detection of SAL from homogenized swine meat was composed of the following three steps: SAL extraction, coupling reaction and SERRS detection. (1) SAL extraction: 1.0 g SAL and 5 mL PBS were mixed in a centrifuge tube and scrolled in a vortex for 2 min, then it was filtered by a microporous aperture membrane (0.45 μm) to obtain the filtrate for further analysis. (2) Coupling reaction: the diazonium ion of aromatic amine was synthesized by adding 0.8% NaNO2 (200 μL) into 20 mM aromatic amines (200 μL), then the extract of SAL (200 μL) and 4% Na2CO3 (200 μL) were added to the solution containing diazonium ions in a centrifuge tube (1.5 mL), finally followed by a manual vortex reaction for 1 min at room temperature. (3) SERRS detection: the particles of Fe3O4@Ag (50 mg mL−1, 20 μL) were dispersed in the above solution and scrolled 1 min for full adsorption of azo dyes, and were then separated and enriched by a 16 hole magnetic rack. SERRS measurement of the SAL-derived azo dye was performed by using a BWTEK Raman spectrometer with a portable optical fiber probe and 532 nm excitation.
Characterizations
The morphology of magnetic particles was characterized using a JEOL JSM-6700F field emission scanning electron microscope (FE-SEM) operating at 3.0 kV. High performance liquid chromatography (HPLC) analysis was carried out on a Shimadzu LC-20AB system (Kyoto, Japan) equipped with a CTO-10AS column oven and an RF-10Axl fluorescence detector. The chromatographic separation of analytes was performed on a VP-ODS C18 column (5 μm, 4.6 mm × 150 mm i.d.). The mobile phase used in HPLC was acetonitrile/water (0.1% H3PO4, pH 2.5) = 25/75. Its flow rate was fixed at 1.0 mL min−1, and SAL the excitation wavelength was set at 276 nm and the emission wavelength was 310 nm. The injection volume was 20 μL for each performance. UV-Vis absorption spectra were taken at 200–800 nm using a Shimadzu UV-2550 spectrometer. The SERS spectrum of the analyte was recorded using a BWTEK Raman spectrometer equipped with different excitation wavelengths, finally fixed at 532 nm after optimization. All the Raman spectra were collected with a laser power of 5.00 mW, while an integration time of 5 s and an accumulation of 5 times was selected for each spectrum.
Results and discussions
Coupling reaction on SAL
SAL has a low cross section for Raman scattering and also is not easily adsorbed on the substrate, so direct SERS detection on SAL is a critical challenge for samples dissolved in solution. Owing to the structural characteristic of SAL as a phenolic compound, an indirect strategy was designed for detection to convert it into an azo dye, which fortunately supports high SERS activity.26,34 The procedure follows the Pauly reaction (Fig. 1), which can be summarized briefly as: the –NH2 group of the agent interacted with the freshly prepared HNO2 (originated from NaNO2 and HCl) to generate the diazonium ions (–N
N+) under acidic conditions; which was then reacted with SAL in basic solution and adjusted by adding Na2CO3. According to the mechanism of the coupling reaction, SAL generates azo dye at the ortho-position of phenolic hydroxyl by electrophilic substitution. This procedure is fast and can be completed within 1 min.
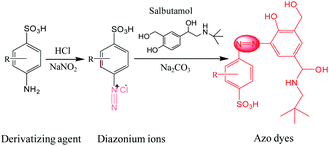 |
| Fig. 1 Schematic for azo-coupling reaction between diazonium ions and SAL. | |
Selection of Pauly reagent for SAL
Then, to investigate the resonance absorption of the target molecules at excitation light, SAL-derived azo dyes were obtained by adding different aromatic amines as (1) sulfanilic acid and (2) 2,2′-benzidinedisulfonic acid (Fig. 2). As exhibited in the photographs of Fig. 2, both processes showed obvious color reaction supplying orange solution finally, although the intermediate diazonium salts were colorless (inset of Fig. 2). In addition, neither product of diazonium salt showed a UV-Vis absorption peak at 450 nm (green line), indicating that there was no interference of it on the subsequent Raman detection. However, when using (2) 2,2′-benzidinedisulfonic acid as a derivative agent, the absorbance strength of the azo dyes was relatively higher than that of (1) sulfanilic acid (pink line) under identical concentrations. Therefore, finally (2) 2,2′-benzidinedisulfonic acid is selected as the derivative agent for SAL to provide a more sensitive SERRS response in the following process.
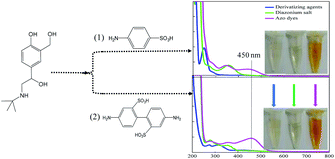 |
| Fig. 2 UV-Vis absorption spectra of diazonium salts and azo dyes, which are achieved by using two different derivative agents of (1) sulfanilic acid and (2) 2,2′-benzidinedisulfonic acid, respectively. | |
The UV-Vis absorption spectra of SAL, diazonium salt (blank), SAL-derived azo dyes and Fe3O4@Ag are illustrated respectively in Fig. 3A, which indicate neither SAL or diazonium salt has an absorption band in the visible range except the SAL-derivative shows a new band at about 450 nm. So, neither of three will interfere with the detection of SAL-derived azo dyes even when the SAL, diazonium salt and/or derivative reagent are largely in excess. Fortunately, the product of the Pauly reaction, azo compound exhibits both a high SERS cross-section and an absorption band centered at 450 nm. As it is very close to the localized surface plasmon resonance (LSPR) absorption of Fe3O4@Ag nanoparticles (415 nm), it is possible to be resonated by excitation to the Raman signal. Therefore, the Fe3O4@Ag-based SERRS probe for the ultrasensitive and selective indirect detection of SAL is established by detecting SAL-derived azo dyes. Consequently, the SERRS spectra of diazonium salt (black) and SAL-derived azo dyes (red) are shown and compared in Fig. 3B, where the non-SERS signal for diazonium salt indicates that neither the derivation agent (2,2′-benzidinedisulfonic acid) nor the Pauly reagent (diazonium salt) will give background interference on the following SERRS detection.
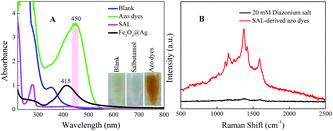 |
| Fig. 3 (A) UV-Vis absorption spectra and (B) SERRS spectra of SAL before and after azo-reaction by using 2,2′-benzidinedisulfonic acid, respectively. | |
Selection of excitation wavelength
It has been demonstrated that the SERRS enhancement factor is closely related to the plasmon resonance of the nanoparticle, laser excitation wavelength, and the molecular resonance.35 If the target molecule contains an absorption band overlapping with the laser excitation, an additional order of magnitude in Raman signal will be achieved.36,37 The SERRS spectra of the azo compound under different incident laser wavelengths of 514, 532, 633, 785 nm are shown in Fig. 4A. By comparing the SERRS intensities, which are close to each other under the excitation wavelengths of 514 and 532 nm, we see that both are relatively higher than the other two wavelengths. Fig. 4B records the histogram of the SERRS peaks intensity at 1591 cm−1 of SAL-derivative under different excitation. As shown, the best enhanced SERRS signal is obtained at the excitation of 514 nm because of the extensive localized surface plasmon resonance (LSPR) absorption. However, in considering the stronger fluorescence interference induced by 514 nm, finally 532 nm is chosen as the excitation wavelength in the following detection.
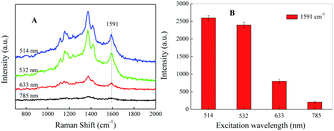 |
| Fig. 4 (A) SERRS spectrum and (B) the corresponding peak intensity at 1591 cm−1 of SAL-derived azo dyes under different incident laser wavelength, respectively. | |
Sensitivity of SAL detection
The indirect SERS analysis is performed after target SAL is changed to azo dye, based on an azo-coupling reaction. As shown in Fig. 5A, the main characteristic peaks of SAL-derived azo dyes are located at 1117, 1160, 1375, 1420, 1591 cm−1, being attributed to ν(C–N), ν(C–C) from phenol groups, δ(C–H), ν(N
N), and ν(C
C) from phenyl rings, respectively.28,34 By comparing all curves, the existence of Raman peaks for SAL even at a concentration as low as 1.0 × 10−11 M is speculated, which confirms that this method can be used to achieve trace detection of SAL. Fig. 5B shows the calibration curves by plotting the SERRS intensities of SAL at 1591 cm−1 versus the log concentration of SAL. Additionally, the color of SAL-derived azo dyes in solution is diminished and became colorless (or light yellow) with the concentration decrease of SAL, as shown in Fig. S3.† Therefore, we can determine the concentrations of SAL by naked eyes based upon the color changes of the solution when it is higher than 2.09 × 10−5 M (5 μg mL−1). However, when the concentration of SAL is less than 5 μg mL−1, there is no obvious color difference among the different concentrations of SAL. To improve the sensitivity of this azo-coupling reaction, Raman spectrometer is required.
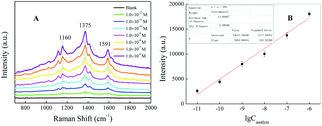 |
| Fig. 5 (A) Concentration-dependent SERRS spectra of the corresponding SAL-derived azo dyes. (B) Intensity of the band at 1591 cm−1 versus the log concentration of SAL. | |
To confirm the reproducibility of the proposed SERRS method, the standard solutions of SAL from 10 batches under the identical concentrations of 1.0 × 10−10 M, were tested. For each sample, the SERRS spectrum and its histogram are shown in Fig. S4,† which are obtained on randomly selected spots on the substrate, respectively. By comparing to the sample-to-sample variation at 1591 cm−1 in the SERRS spectra, remarkable reproducibility is exhibited.
Detection of SAL in real samples
To further demonstrate the practical application of the azo-coupling reaction based SERRS method for SAL detection in real samples, we purchased 5 packages meat randomly from 5 different shops in farm-product markets, in numbering as #1–5. These samples need pretreatments including liquid–solid extraction, coupling reaction and SERRS detection in turn. The spike-and-recovery results are listed in Table 1. None of the original sample is evaluated to contain SAL as tested by SERRS and HPLC (chromatograms and standard curves are shown in Fig. S5†). Then, the original samples are spiked SAL to 1.0 × 10−6 M by using its standard solutions for subsequent extraction and detection, and the results show recoveries in the range of 78–117%, demonstrating the practical application of the azo-coupling reaction based SERRS method and confirming it would be applicable to the rapid detection of SAL in homogenized swine meat. The detection limit in real sample is displayed in Fig. S6,† which can be estimated as 1.0 × 10−10 M. In comparison to other reported methods for SAL detection (Table S1†), the present study exhibits significant advantages to realize rapid and ultra-sensitive detection.
Table 1 Application of azo-coupling reaction based SERRS method for the determination of SAL in homogenized swine meat
Sample no. |
Detection by SERRS and HPLC before spiking |
Spike value (×10−6 M) |
Detection by SERRS (×10−6 M) |
Recovery (%) |
1 |
N/A |
1.00 |
0.78 |
78 |
2 |
N/A |
1.00 |
1.17 |
117 |
3 |
N/A |
1.00 |
0.86 |
86 |
4 |
N/A |
1.00 |
0.94 |
94 |
5 |
NA |
1.00 |
1.05 |
105 |
Interference assay for SAL detection
In order to evaluate anti-interference of the assay to SAL detection, different possible interferences such as clenbuterol, lysine, glycine, glycerol, KCl and NaCl at a concentration of 1.0 × 10−3 M, is mixed with SAL (1.0 × 10−4 M) under the optimal condition. After mixing with magnetic particles, each of them is tested based on the proposed azo-coupling SERRS method. The results show achromatic colour only for the control, while orange for all other solutions containing SAL (Fig. 6A), exhibiting no effect of either on the detection of SAL. Similarly, Fig. 6B gives the SERRS intensity of the SAL derivative and different interference after adding into the magnetic particles as above. It shows a stable intensity at 1591 cm−1, indicating that the mixture of SAL with different interference does not affect the detection of SAL.
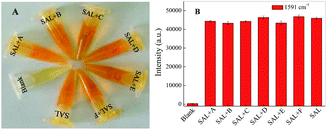 |
| Fig. 6 (A) The photographic images and (B) the SERRS intensity of SAL (1.0 × 10−4 M) and in mixing with the magnetic particles and different interference (1.0 × 10−3 M) as A: clenbuterol, B: lysine, C: glycine, D: glycerol, E: KCl and F: NaCl, respectively. | |
Conclusions
In summary, aiming to rapidly and ultrasensitively detect illegal SAL in swine meat, we propose a new approach in the present study based on an azo-coupling reaction and surface enhanced resonance Raman scattering (SERRS). By combining with a magnetic SERS substrate, as well for separation at the same time, an indirect detection on SAL in solution with a LOD of 1.0 × 10−11 M by SERRS and 2.09 × 10−5 M by naked eye is realized firstly. In addition, with improvement the proposed method is successfully applied to the determination of SAL in real swine meat and offers a simple, practicable, high sensitive and high anti-interference approach. Particularly, the proposed strategy offers a new opportunity for trace amounts of not only SAL but also other illegal additives involved in health products and food, when choosing the corresponding Raman band as specific.
Conflicts of interest
There are no conflicts between the authors to declare.
Acknowledgements
We greatly appreciate the financial support from the projects of NSFC (No. 21373101, 91027027) and the Innovation Program of the State Key Laboratory of Supramolecular Structure and Materials, Jilin University.
Notes and references
- E. Rytting, M. Bur, R. Cartier, T. Bouyssou, X. Wang, M. Kruger, C. M. Lehr and T. Kissel, J. Controlled Release, 2010, 141, 101–107 CrossRef CAS PubMed
. - Y. Zhou, P. Wang, X. Su, H. Zhao and Y. He, Talanta, 2013, 112, 20–25 CrossRef PubMed
. - M. R. Dousti, M. R. Sahar, R. J. Amjad, S. K. Ghoshal and A. Awang, J. Lumin., 2013, 143, 368–373 CrossRef CAS
. - M. Rajkumar, Y. S. Li and S. M. Chen, Colloids Surf., B, 2013, 110, 242–247 CrossRef CAS PubMed
. - S. H. Yazdi, K. L. Giles and I. M. White, Anal. Chem., 2013, 85, 10605–10611 CrossRef CAS PubMed
. - C. Li, Y. L. Wu, T. Yang, Y. Zhang and W. G. Huang-Fu, J. Chromatogr. A, 2010, 1217, 7873–7877 CrossRef CAS PubMed
. - X. D. Du, Y. L. Wu, H. J. Yang and T. Yang, J. Chromatogr. A, 2012, 1260, 25–32 CrossRef CAS PubMed
. - D. Chen, M. Yang, N. Zheng, N. Xie, D. Liu, C. Xie and D. Yao, Biosens. Bioelectron., 2016, 80, 525–531 CrossRef CAS PubMed
. - M. Caban, P. Stepnowski, M. Kwiatkowski, N. Migowska and J. Kumirska, J. Chromatogr. A, 2011, 1218, 8110–8122 CrossRef CAS PubMed
. - X. L. Wang, T. Guo, S. S. Wang, J. P. Yuan and R. S. Zhao, J. Anal. Toxicol., 2015, 39, 213–218 CrossRef PubMed
. - C. C. Guo, F. Shi, L. P. Gong, H. J. Tan, D. F. Hu and J. L. Zhang, J. Pharm. Biomed. Anal., 2015, 107, 526–534 CrossRef CAS PubMed
. - C. Chai, G. Liu, F. Li, X. Liu, B. Yao and L. Wang, Anal. Chim. Acta, 2010, 675, 185–190 CrossRef CAS PubMed
. - X. Lin, Y. Ni and S. Kokot, J. Hazard. Mater., 2013, 260, 508–517 CrossRef CAS PubMed
. - T. A. Nguyen, T. N. Pham, T. T. Doan, T. T. Ta, J. Saiz, T. Q. Nguyen, P. C. Hauser and T. D. Mai, J. Chromatogr. A, 2014, 1360, 305–311 CrossRef CAS PubMed
. - H. Gao, J. Han, S. Yang, Z. Wang, L. Wang and Z. Fu, Anal. Chim. Acta, 2014, 839, 91–96 CrossRef CAS PubMed
. - P. Raksawong, K. Chullasat, P. Nurerk, P. Kanatharana, F. Davis and O. Bunkoed, Anal. Bioanal. Chem., 2017, 409, 4697–4707 CrossRef CAS PubMed
. - J. Tang, Z. Liu, J. Kang and Y. Zhang, Anal. Bioanal. Chem., 2010, 397, 3015–3022 CrossRef CAS PubMed
. - Y. Q. Zhang, J. F. Shen, Z. W. Xie, X. J. Dou, C. J. Min, T. Lei, J. Liu, S. W. Zhu and X. C. Yuan, Nanoscale, 2017, 9, 10694–10700 RSC
. - S. C. Vijayakumar, K. Venkatakrishnan and B. Tan, ACS Appl. Mater. Interfaces, 2017, 9, 5077–5091 Search PubMed
. - X. Q. Fu, Y. X. Chu, K. Zhao, J. G. Li and A. P. Deng, Microchim. Acta, 2017, 184, 1711–1719 CrossRef CAS
. - C. Muehlethaler, M. Leona and J. R. Lombardi, Anal. Chem., 2016, 88, 152–169 CrossRef CAS PubMed
. - R. O. Lezna, N. R. D. Tacconi, S. A. Centeno and A. J. Arvia, Langmuir, 1991, 7, 1241–1246 CrossRef CAS
. - L. Rodríguez-Lorenzo, R. A. Álvarez-Puebla, F. J. G. D. Abajo and L. M. Liz-Marzán, J. Phys. Chem. C, 2010, 114, 7336–7340 Search PubMed
. - X. Zhang, Y. X. Chu, H. Yang, K. Zhao, J. G. Li, H. J. Du, P. She and A. P. Deng, Food Anal. Method, 2016, 9, 3396–3406 CrossRef
. - C. Wei, Y. Zong, Q. H. Guo, M. M. Xu, Y. X. Yuan and J. L. Yao, RSC Adv., 2017, 7, 3388–3397 RSC
. - I. Szele and H. Zollinger, Azo coupling reactions structures and mechanisms, Springer, Berlin, Heidelberg, 1983, vol. 112, pp. 1–66 Search PubMed
. - H. Sui, Y. Wang, Z. Yu, Q. Cong, X. X. Han and B. Zhao, Talanta, 2016, 159, 208–214 CrossRef CAS PubMed
. - X. X. Han, P. Pienpinijtham, B. Zhao and Y. Ozaki, Anal. Chem., 2011, 83, 8582–8588 CrossRef CAS PubMed
. - B. B. Zhou, X. Y. Li, X. H. Tang, P. Li, L. B. Yang and J. H. Liu, ACS Appl. Mater. Interfaces, 2017, 9, 7772–7779 CAS
. - W. G. Qu, L. Q. Lu, L. Lin and A. W. Xu, Nanoscale, 2012, 4, 7358–7361 RSC
. - D. Hong, L. Xiaolin, P. Qing, W. Xun, C. Jinping and L. Yadong, Angew. Chem., Int. Ed., 2005, 44, 2782–2785 CrossRef PubMed
. - P. C. Lee and D. Meisel, J. Phys. Chem., 1982, 86(17), 3391–3395 CrossRef CAS
. - X. H. Tang, R. L. Dong, L. B. Yang and J. H. Liu, J. Raman Spectrosc., 2015, 46, 470–475 CrossRef CAS
. - G. Mcanally, C. Mclaughlin, R. Brown, D. C. Robson, K. Faulds, D. R. Tackley, W. E. Smith and D. Graham, Analyst, 2002, 127, 838–841 RSC
. - J. Zhao, L. Jensen, J. Sung, S. Zou, G. C. Schatz and R. P. Duyne, J. Am. Chem. Soc., 2007, 129, 7647 CrossRef CAS PubMed
. - J. Zhao, J. A. Dieringer, X. Y. Zhang, G. C. Schatz and R. P. Van Duyne, J. Phys. Chem. C, 2008, 112, 19302–19310 CAS
. - A. J. Haes, S. Zou, J. Zhao, G. C. Schatz and R. P. Van Duyne, J. Am. Chem. Soc., 2006, 128, 10905–10914 CrossRef CAS PubMed
.
Footnote |
† Electronic supplementary information (ESI) available. See DOI: 10.1039/c7ra12927k |
|
This journal is © The Royal Society of Chemistry 2018 |