DOI:
10.1039/C7RA12072A
(Paper)
RSC Adv., 2018,
8, 13649-13655
Bio-guided isolation of plant growth regulators from allelopathic plant-Codonopsis pilosula: phyto-selective activities and mechanisms†
Received
4th November 2017
, Accepted 25th March 2018
First published on 12th April 2018
Abstract
Natural pesticides are the subject of growing interest, as the overuse of synthetic pesticides severely threatens the safety of humans and the eco-environment. Allelopathic plants can release plentiful secondary metabolites as natural plant growth regulators to affect the growth of neighboring plants. Bio-guided isolation of the aerial waste part of typical allelopathic plant-Codonopsis pilosula led to six active compounds being produced, including ginsenoside Rg1 (1), ginsenoside Re (2), luteolin (3), luteolin-5-O-glucoside (4), ginsenoside Rb1 (5) and lobetyolin (6). Ginsenosides and luteolin-5-O-glucoside were firstly found in Codonopsis. Phyto-activity tests showed that all compounds showed inhibiting effects toward C. pilosula, and compounds 2, 4, 5 and 6 were also inhibitors of Amaranthus retroflexus. By contrast, the compounds promoted the seedling growth of wheat, rice and Setaria viridis. At certain concentrations, compounds 1, 4, 5 and 1, 2, 4 could observably promote the growth of wheat and rice seedlings, respectively, exceeding Setaria viridis. The different effects toward the two weeds might be related to the different ROS levels induced by the compounds. The ROS amounts in the root tips of S. viridis were as low as those in the control test, and the ROS content in the root tips increased with aggravation of the inhibition effect. In summary, successful isolation of phyto-selective chemicals from allelopathic plants may provide a promising method for natural herbicide screening. The compounds isolated could potentially be applied as inhibitors of dicotyledon weeds and promoters of monocotyledon crops for weed management in agriculture.
Introduction
Pesticides are chemicals that protect crops from weeds, insects and microorganisms.1 Humans have benefited from pesticides in ways such as reducing labor, repelling pests and increasing crop yields. Statistics reveal that 2.4 million tons of pesticides are released into the surroundings worldwide each year. Since the start of the century, their massive usage has threatened the eco-environment and human safety.2–6 Synthetic pesticides may exist in the soil for many years, gradually accumulate in organisms and finally reach human beings through the food chain. Pesticide residue is becoming a key factor affecting human safety. A high incidence of cancer, reproductive and neurodegenerative developmental disorders and human chronic disease are closely associated with exposure to pesticides.7–11
Other than designing safer pesticides and searching for an effective degradation method,12,13 the discovery of natural bioactive compounds is a great alternative with fairly good application prospects in agriculture.14 Biopesticides are gradually emerging with features of low toxicity and easy degradation.15 Many plants have a natural defense system against specific pests, weeds or microorganisms. Varieties of crude extracts or active compounds were recently reported for pesticide usage. From Calceolaria integrifolia s.l., a series of bioactive compounds were isolated showing anti-bacterial, anti-fungal and insect growth regulatory effects.16 Sophora flavescens alkaloid (SFA) is a registered commercial botanical pesticide in China for insect control. Recently, SFA has been found to improve the growth and development of tomato plants.17 Statistically, half of the biopesticides aim to control insects, but valid biopesticides for weed management are very limited.18
Allelopathic plants can release allelochemicals to promote or inhibit the growth of neighboring plants.19,20 Additionally, the aqueous or solvent extracts of allelopathic plants can also show this effect. Therefore, the tissues of allelopathic plants are probably natural reservoirs of plant growth promoters or herbicides.14,21
In northwestern China, widely cultivated Chinese traditional herbs can supply plentiful plant sources for botanical herbicides. Codonopsis pilosula, one of the five main medicinal herbs in Gansu Province, is a representative allelopathic plant showing a severe autotoxic effect. Local peasants all know that the cultivated soil of C. pilosula can no longer be used to cultivate the same plant, but they must choose Gramineae as rotation plants. All of this evidence reveals that C. pilosula may be rich in phyto-active compounds. The dried root of C. pilosula is notable for replenishing vitality-nourishing compounds in the blood and having a health-care effect. However, the aerial part that is abandoned as plant waste also contains abundant secondary metabolites. The improper treatment of the discarded part of the herbal medicine would affect the growth of crops. Therefore, the high-value utilization of the aerial part of the herbal medicine is now an urgent research area. The aerial part was then chosen as the plant source for natural agrochemical screening. In this work, a mass of active substances as potential plant growth regulators were reported for the first time from the aerial waste part of C. pilosula.
Materials and methods
Chemical reagents and instruments
Three column chromatographic (CC) packings were used: silica gel (200–300 mesh, Qingdao Haiyang Chemical Co., Ltd., China), MCI gel (CHP20/P120 75–150 μm, Mitsubishi Chemical Co., Japan) and Sephadex LH-20 gel (Pharmacia, Germany). Silica gel 60 F254 plates used for thin layer chromatography (TLC) were purchased from Qingdao Haiyang Chemical Co., Ltd. TLC spots were viewed under UV light (254 nm) or with the naked eye after a sulfuric acid color reaction (5% v/v in C2H5OH). Silica gel (GF254, 10–40 μm) was used in preparative TLC. All solvents used were redistilled before use. Nuclear magnetic resonance (NMR) spectra were measured on a Bruker AM-400BB instrument (400 MHz for 1H NMR, 100 MHz for 13C NMR), and high-resolution mass data were measured on ESI-Q-TOF MS equipment (Bruker micro TOF Q II). ROS production was viewed using a fluorescence microscope (Olympus FV 1200) at an excitation wavelength of 488 nm and an emission wavelength of >520 nm. A Nexus 870 instrument was used for infrared measurement with the KBr pellet method.
HPLC analysis
HPLC analysis was carried out on a Waters 1525 system with a Waters 2998 PDA detector and a 4.6 mm × 250 mm, 5.0 μm, symmetry C18 column (Milford, Massachusetts, USA). The solvent of methanol for HPLC analysis was of HPLC gradient grade purchased from Tintometer, GmbH. UV spectra were recorded between 209.8 and 400 nm. The mobile phase, composed of methanol and water, was gradually changed from 10% to 100% methanol over the period of 0–30 min and then maintained at 100% methanol until 50 min. The flow rate of the mobile phase was 1.0 mL min−1 and the column temperature was 35 °C.
Plant materials
The aerial part and seeds of C. pilosula were collected in Tanchang country of Gansu Province in northwestern China and identified by Professor Jin Ling from the Gansu University of Chinese Medicine. Seeds of Amaranthus retroflexus, Triticum aestivum (wheat) and Setaria viridis were purchased from the Gansu Academy of Agricultural Sciences. Seeds of Oryza sativa (rice, Daliang 202) were purchased from the Linyi Daliang Seeds Company.
Extraction and isolation
The aerial part of C. pilosula was dried in the dark and chopped into small sticks, immersed in 90% ethanol 4 times for 7 days each time, and the lixivium was collected. The lixivium was concentrated to obtain a crude extract (200 g). Subsequently, the crude extract was subjected to silica-gel CC elution using petroleum ether/acetone (v/v 100
:
1, 20
:
1, 10
:
1, 5
:
1, 1
:
1) and methanol to get Fr.1–Fr.11. Fr.1, Fr.4, Fr.5, Fr.8, and Fr.9 were discarded based on TLC analysis. The inhibition effects of Fr.2, Fr.3, Fr.6, Fr.7, Fr.10, and Fr.11 on C. pilosula seedlings were tested. The most effective fraction (Fr.11, 80 g) was separated via MCI gel CC eluting using methanol-water (1
:
10, 1
:
5, 1
:
1, 3
:
1, 5
:
1) and acetone to get fractions (Fr.11-1–Fr.11-11). Fr.11-3 was separated via preparative thin layer chromatography (PTLC) developed with chloroform/methanol (3
:
1) to obtain compound 6 (14 mg). Fr.11-6 was submitted to LH-20 CC and further purified using PTLC to obtain compound 1 (100 mg), 2 (10 mg), and 3 (120 mg). Fr.11-9 was purified using PTLC to yield compound 5 (20 mg). Fr.11-11 was fractioned using LH-20 gel CC and purified using PTLC to yield compound 4 (30 mg).
Ginsenoside Rg1 (1). White powder; 1H NMR (400 MHz, CD3OD) δ 5.10 (1H, t, 6.6 Hz, H-24), 4.61 (1H, d, 7.6 Hz, H-1′′), 4.35 (1H, d, 8 Hz, H-1′), 4.09 (1H, td, 10.4, 2.4 Hz, H-6), 3.79 (2H, m, H-6′), 3.64 (3H, m, H-6′′, 12), 3.05–3.40 (9H, m, H-3, 2′–5′, 2′′–5′′), 2.28 (1H, m, H-17), 2.07 (3H, m, H-23, 7), 1.68 (3H, s, H-26), 1.62 (3H, s, H-27), 1.34 (3H, s, H-21), 1.33 (3H, s, H-28), 1.10 (3H, s, H-18), 1.00 (3H, s, H-29), 0.99 (3H, s, H-19), 0.95 (3H, s, H-30). C42H72O14. HR-ESI-MS m/z 823.4834 [M + Na]+ (calcd for C42H72NaO14, 823.4814). HPLC purity: 95.10%. IR (cm−1): 3416.03, 2930.16, 1727.98, 1637.55, 1457.74, 1385.78, 1308.32, 1075.53, 1031.09, 890.50, 528.37.
Ginsenoside Re (2). White powder; 1H NMR (400 MHz, CD3OD) δ 5.27 (1H, brs, H-1′′′), 5.06 (1H, t, 6.6 Hz, H-24), 4.59 (1H, d, 6.8 Hz, H-1′′), 4.56 (1H, d, 9.6 Hz, H-1′), 4.30 (1H, td, 3.2, 10.8 Hz, H-6), 4.04 (1H, dt, 6.4, 9.6 Hz, H-5′′), 2.23 (1H, m, H-17), 2.02 (2H, m, H-23), 1.63 (3H, s, H-26), 1.58 (3H, s, H-27), 1.30 (3H, s, H-21), 1.29 (3H, s, H-28), 1.19 (3H, d, 6.0 Hz, H-6′′), 1.05 (3H, s, H-18), 0.92 (3H, s, H-29), 0.91 (3H, s, H-19), 0.89 (3H, s, H-30). HR-ESI-MS: m/z 969.5386 [M + Na]+ (calcd for C48H82NaO18, 969.5393). HPLC purity: 95.51%. IR (cm−1): 3378.33, 2937.55, 1638.55, 1452.94, 1390.35, 1317.96, 1074.91, 1046.58, 890.06, 841.23, 813.65, 657.79, 618.02.
Luteolin (3). Yellow powder; 1H NMR (400 MHz, DMSO) δ 7.43–7.38 (2H, m, H-6′, 2′), 6.89 (1H, d, 8.4 Hz, H-5′), 6.67 (1H, s, H-3), 6.44 (1H, d, 2.0 Hz, H-8), 6.19 (1H, d, 2.0 Hz, H-6). HR-ESI-MS: m/z 287.0550 [M + H]+ (calcd for C15H11O6, 287.0550). HPLC purity: 99.91%. IR (cm−1): 3421.45, 3071.02, 2694.22, 1655.47, 1611.61, 1576.51, 1501.83, 1442.17, 1367.07, 1266.12, 1163.62, 1031.75, 839.18, 685.18, 565.37.
Luteolin-5-O-glucoside (4). Yellow powder; 1H NMR (400 MHz, DMSO) δ 10.95, 7.39 (2H, m, H-6′, 2′), 6.88 (1H, d, 8 Hz, H-5′), 6.80 (1H, d, 2.4 Hz, H-6), 6.70 (1H, d, 2.0 Hz, H-8), 6.55 (1H, s, H-3), 4.71 (1H, d, 7.2 Hz, H-1′′), 3.76 (1H, d, 10.8 Hz, H-6′′), 3.56 (1H, m, H-6′′), 3.33–3.34 (3H, m, H-2′′, 3′′, 5′′), 3.22 (1H, m, H-4′′). HR-ESI-MS: m/z 471.0896 [M + Na]+ (calcd for C21H20NaO11, 471.0898). HPLC purity: 95.21%. IR (cm−1): 3305.05, 2957.63, 1727.64, 1656.42, 1598.38, 1496.09, 1446.49, 1374.73, 1262.28, 1179.23, 1070.72, 1034.44, 1016.44, 843.16, 822.21, 629.22.
Ginsenoside Rb1 (5). White powder; 1H NMR (400 MHz, CD3OD) δ 5.09 (1H, t, 6 Hz, H-24), 4.63 (1H, d, 7.6 Hz, H-1′′′′), 4.55 (1H, d, 8 Hz, H-1′), 4.39 (1H, t, 6.4 Hz, H-1′′′), 4.31 (1H, d, 7.6 Hz, H-1′′), 1.64 (3H, s, H-26), 1.58 (3H, s, H-27), 1.32 (3H, s, H-21), 1.02 (3H, s, H-28), 0.96 (3H, s, H-29), 0.87 (6H, s, H-18, 30), 0.81 (3H, s, H-19). HR-ESI-MS m/z 1131.5886 [M + Na]+ (calcd for C54H92NaO23 1131.5922). HPLC purity: 91.36%. IR (cm−1): 3414.87, 2929.60, 1730.17, 1648.77, 1456.61, 1386.44, 1272.59, 1076.88, 890.73, 574.50.
Lobetyolin (6). Yellow powder; 1H NMR (400 MHz, CD3OD) δ 6.33 (1H, m, H-2), 5.91 (1H, m, H-11), 5.57 (1H, d, 14.0 Hz, H-3), 5.46 (1H, m, H-10), 4.43 (1H, d, 6.4 Hz, H-8), 4.32 (1H, d, 7.2 Hz, H-1′), 4.27 (1H, m, H-9), 3.85–3.65 (2H, m, H-6′), 3.57 (2H, t, 6.4 Hz, H-14), 3.12–3.20 (4H, m, H-2′-5′), 2.18 (2H, dt, 15.2, 7.4 Hz, H-12), 1.80 (3H, dd, 6.8, 1.6 Hz, H-1), 1.65 (2H, m, H-13). HR-ESI-MS m/z 419.1702 [M + Na]+ (calcd for C20H28NaO8, 419.1676). HPLC purity: 99.97%. IR (cm−1): 3389.53, 2917.58, 2234.25, 1730.02, 1668.30, 1626.17, 1441.75, 1292.07, 1159.73, 1078.22, 1041.59, 631.96.
Bioassays
The seeds of the target plants were germinated on wet filter paper at 25 °C in the dark (four days for C. pilosula, three days for rice, and one day for A. retroflexus, S. viridis and wheat). The crude extract and the different fractions (4 mg) were dissolved in DMSO (100 μL) and diluted with distilled sterile water to prepare processed solutions (400 μg mL−1). The separated compounds (4.8 mg) were dissolved in DMSO (240 μL) and diluted with distilled sterile water to prepare processed solutions (200, 100, 50, 25, 10 and 1 μg mL−1). The control solutions were prepared by adding the same volume of organic solvents to distilled water (1% DMSO). Compared with blank tests (distilled water), the control tests did not exhibit significant differences. Proper volumes of the solutions were added, and uniformly growing seedlings cultured in a 6-well-plate at 25 °C placed in a constant temperature dark chamber were chosen. The root and stem lengths of the seedlings were measured after 2 days (excluding C. pilosula seedlings, which were measured after 4 days due to their slow growth). Every test was repeated three times.
ROS production
ROS production was measured using Yamamoto’s method with slight modifications.20 2′,7′-Dichlorofluorescein diacetate was applied to label the ROS. Treated seedlings were washed 5 times with distilled water and dyed for 15 min in the dark using 2′,7′-dichlorofluorescein diacetate (20 μmol L−1, 1% DMSO). Then the dye residues were washed off in water, and the root tips were carefully excised to be viewed using the fluorescence microscope (Olympus FV 1200).
Statistical analysis
Fisher’s least significant difference (LSD) test and one-way analysis of variance (ANOVA) were used to mark the significant differences between the tested group and the control group via SPSS 18.0. The inhibition ratio of seedling growth (%) was calculated using the formula [1 − treated length/control length] × 100%. A negative figure showed that the compound could accelerate the seedling growth.
Results
Bio-guided isolation and identification
The phytotoxic effects of the crude extract and the different fractions of the aerial part of C. pilosula were tested on C. pilosula seedlings at 400 μg mL−1 (Fig. 1). The inhibition ratios of the crude extract on root and stem elongation of C. pilosula seedlings were 64% and 21%, respectively. The high polarity fraction eluted using methanol gained the maximum weight and was most active. The inhibition ratios of Fr.11 on root and stem growth were 80% and 62%, respectively, which were much higher than those of other fractions. Six active compounds were isolated from this fraction and identified as (Fig. 2): ginsenoside Rg1 (compound 1),22,23 ginsenoside Re (compound 2),22–24 luteolin (compound 3), luteolin-5-O-glucoside (compound 4),25 ginsenoside Rb1 (compound 5),24 and lobetyolin (compound 6).26
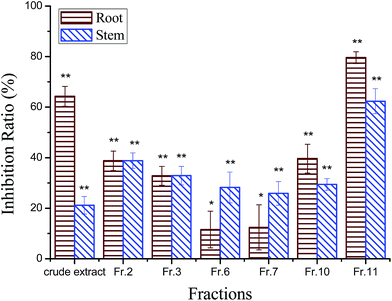 |
| Fig. 1 Autotoxic effect of the crude extract and different fractions of the aerial part of C. pilosula. Values are presented as a percentage of the mean compared to the control. Means significantly lower than the control are indicated with one asterisk (*) (one way ANOVA, p < 0.05) or two asterisks (**) (p < 0.01). Error bars are one standard error of the mean. N = 3. | |
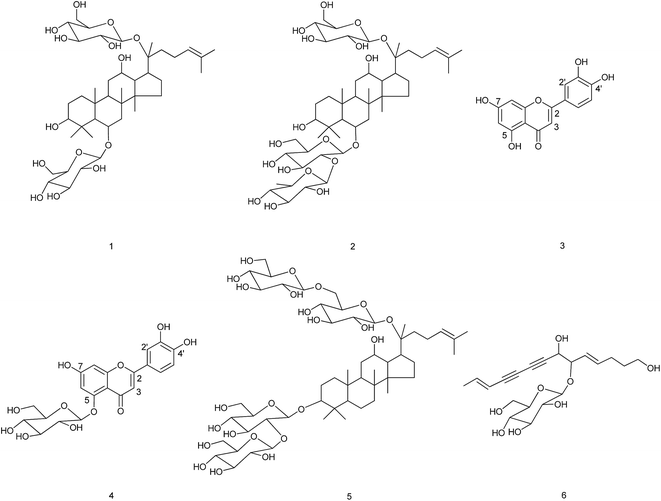 |
| Fig. 2 Compounds isolated from the aerial part of cultivated C. pilosula. | |
Activities
Two dicotyledons (C. pilosula and A. retroflexus) and three monocotyledons (T. aestivum, O. sativa and S. viridis) were chosen as target plants to evaluate the phyto-activity of the isolated compounds via a plate-culture method. T. aestivum (common wheat) and O. sativa (cultivated rice) are representative crops of gramineae worldwide. A. retroflexus and S. viridis are common weeds in the farmland of China. The compounds showed differentiated activities toward different target plants (Fig. 3). As shown in Fig. 3(a), ginsenoside Rg1 (compound 1) inhibited root and stem elongation of C. pilosula seedlings roughly in the ratios of 40% and 40–60% (excluding 27% inhibition of stem elongation at 25 μg mL−1). Ginsenoside Rg1 selectively affected the radicle growth of the four other tested plants. At low concentrations, ginsenoside Rg1 promoted radicle growth of wheat (10–25 μg mL−1) and rice (1–50 μg mL−1), both in a ratio of about 20%. At concentrations from 100 to 200 μg mL−1, the compound promoted radicle growth of S. viridis and the promoting ratio was about 20%.
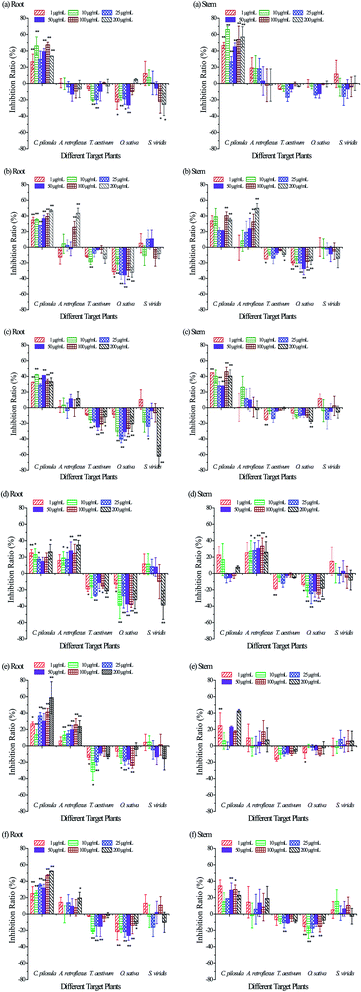 |
| Fig. 3 Phyto-activities of the compounds isolated from the aerial part of C. pilosula. (a)–(f) respectively represent the activities of compounds 1–6 on root or stem growth. Values are presented as a percentage of the mean compared to the control. Means significantly lower than the control are indicated with one asterisk (*) (one way ANOVA, p < 0.05) or two asterisks (**) (p < 0.01). Error bars are one standard error of the mean. N = 3. | |
As shown in Fig. 3(b), the inhibition ratios of ginsenoside Re (compound 2) on C. pilosula seedlings were about 40% and 35% for root and stem elongation, respectively. Ginsenoside Re showed weak activity toward the seedling growth of wheat with the promotion ratio below 20%. The seedling growth of rice was markedly accelerated with the ratios increasing to 35% and 28% for root and stem lengths at the optimum concentration. Notably, ginsenoside Re could suppress seedling growth of A. retroflexus by the ratio of about 43% for root length and 50% for stem length at the maximum concentration.
Except for the similar inhibitory effect on C. pilosula, luteolin (compound 3) could markedly accelerate radicle growth of the three monocotyledons, as shown in Fig. 3(c): 25% for wheat at 50 μg mL−1, 40% for rice at 25 μg mL−1, and 62% for S. viridis at 200 μg mL−1. Compared to luteolin, the inhibitory effect of luteolin-5-O-glucoside on C. pilosula was slightly weakened, but the inhibition ratios on A. retroflexus were increased to 35% and 26% for root and stem length at 200 μg mL−1, as shown in Fig. 3(d). The promoting effect of luteolin-5-O-glucoside on wheat and rice was similar to that of luteolin but the promoting ratio on the S. viridis radicle was reduced to 39%.
As shown in Fig. 3(e), ginsenoside Rb1 (compound 5) inhibited seedling growth of C. pilosula in the ratios of 59% and 43% at 200 μg mL−1 for radicle and caulicle growth, respectively. Ginsenoside Rb1 showed slight inhibition on A. retroflexus. The maximum promoting ratios on the radicle growth of wheat and rice were 32% and 24%, respectively. Lobetyolin (compound 6) showed moderate inhibition on C. pilosula and slight promotion on wheat and rice as well, as shown in Fig. 3(f).
ROS production was measured in the root tips of A. retroflexus and S. viridis after being treated with the compounds for 48 h at 200 μg mL−1 (Fig. 4). Compounds 2 and 4 markedly induced accumulation of ROS in A. retroflexus root tips, and compounds 5 and 6 led to a slight increase in A. retroflexus root tips. ROS amounts in the root tips of S. viridis seedlings treated with the compounds were very low and showed no differences to the control test.
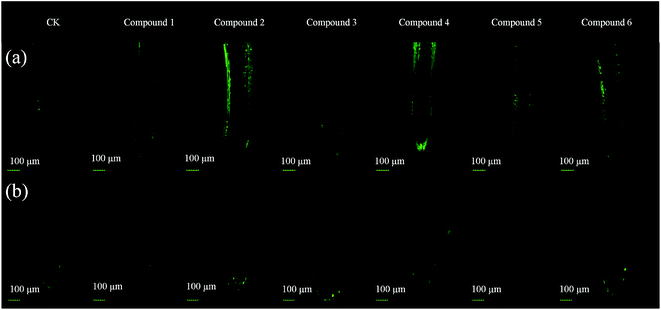 |
| Fig. 4 Representative pictures of ROS production in A. retroflexus (a) and S. viridis (b) root tips after treatment with compounds 1–6. The seedlings were treated using the same method as in the activities test at 200 μg mL−1, then stained with DCFH-DA. Pictures were taken using a fluorescence microscope. The bright green fluorescence shows the ROS. | |
Discussion
Phyto-selective activities
Triterpenoids, polysaccharides, polyacetylenes, phenylpropanoids, flavones, alkaloids, etc. were the main active constituents of C. pilosula.27 Ginsenosides, typical representatives of the panax genus, were firstly isolated from the aerial part of C. pilosula. This result revealed the chemical reason for the similar effect of C. pilosula in some aspects with ginseng. Excluding pharmacological properties such as anti-tumor effects, the strengthening of immunity and other health-care effects, recently ginsenosides were reported to show phyto-activities as well.28–32 It was found that ginsenosides Rg1 and Re showed autotoxicity toward Panax notoginseng by inhibiting germination and seedling growth,32 but the phyto-activities of these two ginsenosides towards other plants were unclear. In this work, three ginsenosides Rg1, Re and Rb1, purified from the aerial part of C. pilosula, were tested for activity toward common crops and weeds. Ginsenosides, dammarane-type triterpene glycosides, can be classified as protopanaxadiol (PPD) or protopanaxatriol (PPT) types. Ginsenosides Rg1 and Re are both classified as PPT-type ginsenosides. Ginsenoside Rb1 is classified as a PPD-type ginsenoside with four sugar moieties. The protopanaxatriol skeleton and three sugar moieties endow Re with excellent properties. Ginsenoside Re (compound 2) showed the strongest inhibition effect on A. retroflexus, followed by Rb1 (compound 5) with a slight inhibition effect. In addition to inhibiting the growth of the dicotyledon weed, ginsenoside Re (compound 2) behaved as a promising growth promoter of rice as well. The promotion effect of ginsenoside Rg1 (compound 1) on the tested monocotyledon crops exceeded that on the weed S. viridis at 1–10 μg mL−1. Ginsenoside Rb1 (compound 5) could selectively promote the growth of wheat at 1–25 μg mL−1 among the tested plants. This is the first report about the selective activities of ginsenosides toward monocotyledons and dicotyledons. Previous investigations reported that hederagenin saponins with 16-alpha-hydroxy protobassic acid glycoside isolated from Sapindus mukorossi Gaertn and Diploknema butyracea were defined as growth promoters of rice and maize.33 These results are in good accordance with the previous studies and enrich the phyto-activities of the triterpene saponins.
Luteolin-5-O-glucoside (compound 4) was isolated from C. pilosula for the first time. Previous investigations showed that luteolin and its glycoside could reduce the growth of Lemna gibba plants by reducing the chlorophyll content and frond number.34 In this work, compared to its aglycone, luteolin-5-O-glucoside led to reduction of the inhibition effect on C. pilosula and promotion effect on S. viridis, but resulted in an increase of the inhibition effect on A. retroflexus. Under the tested conditions, luteolin-5-O-glucoside behaved as an inhibitor on A. retroflexus and an accelerator on monocotyledons crops with no significant promotion on S. viridis at 1–50 μg mL−1. Lobetyolin (compound 6) is a representative polyacetylene of the genus Codonopsis. The phyto-activity of this compound was reported for the first time and it was defined as another growth inhibitor of C. pilosula. Although lobetyolin slightly inhibited the seedling growth of A. retroflexus, it showed a promotion effect on the seedling growth of S. viridis which would prevent it from usage as a herbicide.
General trends of activities
Six active compounds were isolated, of which ginsenosides (compounds 1, 2, and 5) and luteolin-5-O-glucoside (compound 4) were purified for the first time from C. pilosula. The phyto-activities of the compounds basically followed a similar trend. Firstly, the compounds showed an inhibition effect on C. pilosula. The inhibitory ratios of the active substances on C. pilosula were 40–60% at all tested concentrations, except for a slight reduction of luteolin-5-O-glucoside (compound 4) to 26%. Secondly, some compounds showed a dose-dependent inhibition effect on A. retroflexus. Ginsenoside Re (compound 2) and luteolin-5-O-glucoside (compound 4) markedly reduced the seedling growth of A. retroflexus. Ginsenoside Rb1 (compound 5) only showed a slight inhibition effect. The other three compounds did not show a significant effect on A. retroflexus. Thirdly, the compounds displayed a promotion effect on wheat, rice and S. viridis at the treated concentrations. The promotion effects on wheat and rice generally work at low concentrations but the effects on S. viridis increased with concentration. The maximum effective concentrations on wheat and rice were generally lower than those on S. viridis. Therefore, proper concentrations could be chosen to regulate the seedling growth of crops and weeds. Ginsenoside Rg1 (compound 1) and luteolin-5-O-glucoside (compound 4) promoted the seedling growth of wheat and rice at low concentrations without significant promotion on S. viridis. Ginsenoside Rb1 (compound 5) could be used as a growth stimulator of wheat at 1–25 μg mL−1. Additionally, the growth-promoting effect of ginsenoside Re (compound 2) on rice was much stronger than that on S. viridis over the whole tested range of concentrations. It is worth noting that the promotion effect on the root of wheat or rice was stronger than that on the stem. In conclusion, at the proper concentrations, the natural products in the aerial part of C. pilosula showed an inverse effect toward monocotyledons and dicotyledons, so are promising growth regulators of plants.
Mechanisms
The isolated compounds showed dose-dependent inverse effects on two weeds: inhibition on A. retroflexus and promotion on S. viridis. Therefore the two weeds were chosen to investigate the action mechanisms of these compounds at the maximum concentration. In previous studies, it was reported that ROS were involved in seedling growth inhibition of phytotoxic chemicals.19,35–37 The amount of reactive oxygen species in normal root tips is very low. Phytotoxic chemicals would lead to accumulations of ROS. As shown in Fig. 4, the ROS amounts approximately followed the same trend as the inhibition activities. Compounds 2 and 4 caused a significant increase of the ROS content in the root tips of A. retroflexus, followed by compounds 5 and 6. The ROS production in the root tips of S. viridis showed no differences to the control. The ROS content would increase when under environmental stress and excessive ROS would disturb the balance in the plant, cause lipid peroxidation, cell viability reduction, and physiological process disorder, resulting in growth inhibition of the target plants. The same compounds induced different levels of ROS accumulation between A. retroflexus and S. viridis, which might result in the phyto-selective activities.
Conclusion
The active compounds from tonic herbs are environmentally friendly as they have a low price and low toxicity, and they are easy to degrade.15,16,28–31 Bio-guided isolation of the discarded aerial part of C. pilosula led to a group of components with phyto-selective activities, which are promising in applications as natural herbicides and growth promoters. The phyto-selective effects might involve different amounts of ROS in different plants. These findings enriched the bioactivities of the secondary metabolites in C. pilosula and extended the applications of waste plant materials as potential resources to be developed in agriculture.
Conflicts of interest
There are no conflicts to declare.
Acknowledgements
This work was supported by the National Natural Science Foundation of China (No. 31570354, 21775154, 21302195, 31300290, 31772668 and 31560037), the CAS ‘‘Light of West China’’ Program, the Youth Science Foundations of Gansu Province (No. 1506RJYA294), and around five top priority programs of the “One-Three-Five” Strategic Planning of the Lanzhou Institute of Chemical Physics, Chinese Academy of Sciences.
Notes and references
- S. Mostafalou and M. Abdollahi, Arch. Toxicol., 2017, 91, 549–599 CrossRef CAS PubMed.
- J. E. Casida and K. A. Durkin, Chem. Res. Toxicol., 2017, 30, 94–104 CrossRef CAS PubMed.
- A. M. Cimino, A. L. Boyles, K. A. Thayer and M. J. Perry, Environ. Health Perspect., 2017, 125, 155–162 Search PubMed.
- H. M. M. T. B. Herath, S. P. Pahalagamage, N. Yogendranathan, M. D. M. S. Wijayabandara and A. Kulatunga, BMC Pharmacol. Toxicol., 2017, 18, 6 CrossRef CAS PubMed.
- V. Storck, D. G. Karpouzas and F. Matin-Laurent, Sci. Total Environ., 2017, 575, 1027–1033 CrossRef CAS PubMed.
- S. Yazicioglu, G. Bankaoglu and O. Aydemir, in Geoconference on Ecology, Economics, Education And Legislation, Sgem, 2013, vol. 1, pp. 1107–1112 Search PubMed.
- T. VoPham, K. A. Bertrand, J. E. Hart, F. Laden, M. M. Brooks, J. M. Yuan, E. O. Talbott, D. Ruddell, C. C. H. Chang and J. L. Weissfeld, Cancer, Causes Control, Pap. Symp., 2017, 28, 177–190 CrossRef PubMed.
- S. Gangemi, E. Miozzi, M. Teodoro, G. Briguglio, A. De Luca, C. Alibrando, I. Polito and M. Libra, Mol. Med. Rep., 2016, 14, 4475–4488 CrossRef CAS PubMed.
- K. H. Kim, E. Kabir and S. A. Jahan, Sci. Total Environ., 2017, 575, 525–535 CrossRef CAS PubMed.
- M. Ye, J. Beach, J. W. Martin and A. Senthilselvan, J. Environ. Sci., 2017, 51, 361–370 CrossRef PubMed.
- A. Sengupta, K. Manna, S. Datta, U. Das, S. Biswas, N. Chakrabarti and S. Dey, RSC Adv., 2017, 7, 13957–13970 RSC.
- Y. Fu, M. X. Wang, D. Zhang, Y. W. Hou, S. Gao, L. X. Zhao and F. Ye, RSC Adv., 2017, 7, 46858–46865 RSC.
- X. Y. Zhao, L. Wang, L. N. Du, J. X. Yang, J. Dong and F. Ma, RSC Adv., 2017, 7, 33177–33184 RSC.
- F. A. Macias, J. C. G. Galindo, J. M. G. Molinillo and D. Castellano, Phytochemicals, 2000, 54, 165–171 CrossRef CAS.
- J. Deravel, F. Krier and P. Jacques, Agron. Soc., 2014, 18, 220–232 Search PubMed.
- C. L. Cespedes, J. R. Salazar, A. Ariza-Castolo, L. Yamaguchi, J. G. Avila, P. Aqueveque, I. Kubo and J. Alarcon, Environ. Res., 2014, 132, 391–406 CrossRef CAS PubMed.
- X. Xiong, M. Yao, L. L. Fu, Z. Q. Ma and X. Zhang, Ind. Crops Prod., 2016, 92, 13–18 CrossRef CAS.
- S. Attia, K. L. Grissa, G. Lognay, E. Bitume, T. Hance and A. C. Mailleux, J. Pestic. Sci., 2013, 86, 361–386 Search PubMed.
- X. Z. Li, Z. Q. Yan, L. Pan, H. Jin, X. Y. Yang, J. D. Liu, X. F. He, X. Ren, M. Xie, K. Guo and Q. Bo, Phytochem. Lett., 2017, 20, 208–213 CrossRef CAS.
- M. Xie, Z. Q. Yan, X. Ren, X. Z. Li and B. Qin, J. Agric. Food Chem., 2017, 65, 2032–2038 CrossRef CAS PubMed.
- K. Jabran, G. Mahajan, V. Sardana and B. S. Chauhan, Crop Prot., 2015, 72, 57–65 CrossRef.
- G. Y. Zhu, Y. W. Li, D. K. P. Hau, Z. H. Jiang, Z. L. Yu and W. F. Fong, J. Agric. Food Chem., 2011, 59, 200–205 CrossRef CAS PubMed.
- D. Lu, P. Li and J. Liu, Nat. Prod. Res., 2012, 26, 1395–1401 CrossRef CAS PubMed.
- Y. S. Wang, Y. P. Jin, W. Gao, S. Y. Xiao, Y. W. Zhang, P. H. Zheng, J. Wang, J. X. Liu, C. H. Sun and Y. P. Wang, J. Ginseng Res., 2016, 40, 245–250 CrossRef PubMed.
- X. J. Zhou, L. L. Yan, P. P. Yin, L. L. Shi, J. H. Zhang, Y. J. Liu and C. Ma, Food Chem., 2014, 164, 150–157 CrossRef CAS PubMed.
- J. Jing, J. F. Zhang, H. Li, S. Huang, L. H. Shan and X. L. Zhou, Lishizhen Med. Mater. Med. Res., 2013, 24, 2340–2342 CAS.
- J. Y. He, N. Ma, S. Zhu, K. Komatsu, Z. Y. Li and W. M Fu, J. Nat. Med., 2015, 69, 1–21 CrossRef CAS PubMed.
- T. Ahmed, S. H. Raza, A. Maryam, W. N. Setzer, N. Braidy, S. F. Nabavi, M. R. de Oliveira and S. M. Nabavi, Brain Res. Bull., 2016, 125, 30–43 CrossRef CAS PubMed.
- S. Y. Nah, Front. Physiol., 2014, 5, 98 Search PubMed.
- L. Peng, S. Sun, L. H. Xie, S. M. Wicks and J. T. Xie, Cardiovasc. Ther., 2012, 30, 183–188 CrossRef PubMed.
- L. Song, M. B. Xu, X. L. Zhou, D. P. Zhang, S. L. Zhang and G. Q. Zheng, Oxid. Med. Cell. Longevity, 2017, 2163053 Search PubMed.
- M. Yang, X. D. Zhang, Y. G. Xu, X. Y. Mei, B. B. Jiang, J. J. Liao, Z. B. Yin, J. F. Zheng, Z. Zhao, L. M. Fan, X. H. He, Y. Y. Zhu and S. S. Zhu, PLoS One, 2015, 10, 0118555 Search PubMed.
- S. Saha, S. Walia, J. Kumar and B. S. Parmar, J. Appl. Bot. Food Qual., 2010, 83, 189–195 CAS.
- C. W. Beninger and J. C. Hall, Biochem. Syst. Ecol., 2005, 33, 103–111 CrossRef CAS.
- Y. Ueda, N. Uehara, H. Sasaki, K. Kobayashi and T. Yamakawa, Plant Physiol. Biochem., 2013, 70, 396–402 CrossRef CAS PubMed.
- Z. Q. Yan, D. D. Wang, L. Ding, H. Y. Cui, H. Jin, X. Y. Yang, J. S. Yang and B. Qin, Plant Physiol. Biochem., 2015, 88, 53–59 CrossRef CAS PubMed.
- L. Pan, X. Z. Li, Z. Q. Yan, H. R. Guo and B. Qin, Plant Physiol. Biochem., 2015, 97, 272–277 CrossRef CAS PubMed.
Footnote |
† Electronic supplementary information (ESI) available. See DOI: 10.1039/c7ra12072a |
|
This journal is © The Royal Society of Chemistry 2018 |
Click here to see how this site uses Cookies. View our privacy policy here.