DOI:
10.1039/C7RA11565B
(Paper)
RSC Adv., 2018,
8, 6315-6325
One-step preparation of a novel SrCO3/g-C3N4 nano-composite and its application in selective adsorption of crystal violet
Received
19th October 2017
, Accepted 31st January 2018
First published on 7th February 2018
Abstract
A novel kind of nanoparticle SrCO3/g-C3N4 was prepared using strontium carbonate (SrCO3) and melamine (C3H6N6) as raw materials via one-step calcination. The formation of SrCO3/g-C3N4 was confirmed from the X-ray diffraction (XRD), Fourier transform infrared spectra (FT-IR), Scanning Electron Microscopy (SEM), Transmission Electron Microscopy (TEM), Brunauer–Emmett–Teller (BET) and X-ray photoelectron spectroscopy (XPS) analysis. Its selective adsorption performance was evaluated towards crystal violet (CV), rhodamine B (RhB) and methylene blue (MB). The results showed that the SrCO3/g-C3N4 had selective adsorption ability of CV. Furthermore, adsorption measurements of CV were conducted to investigate the influences of contact time, initial concentration, initial dye solution pH value and adsorbent dosage. The maximum removal rate of CV was 98.56% when the initial concentration was 1600 mg L−1. The kinetic study indicated the adsorption of CV followed the pseudo-second-second model well. The adsorption efficiency of SrCO3/g-C3N4 was greater (97.46%) than that of g-C3N4 (31.30%) and SrCO3 (17.30%). It could be deduced that the synergistic effect of conjugation interaction of g-C3N4 and the electrostatic attraction of SrCO3 might be the main driving force for the superb adsorption of CV.
1 Introduction
Nowadays, graphitic carbon nitride (g-C3N4) has gained considerable research attention because it possesses excellent advantages such as high chemical and thermal stability under ambient temperature, cost-effective, non-toxic and simple preparation.1,2 g-C3N4, as a new intriguing class of graphite analogue, consists of conjugated planes containing highly ordered tri-s-triazine (C6N7) units. The layered structure of the tri-s-triazine is connected by weak forces-van der Waal forces. It has been widely used in the photocatalysis field for it has an energy band gap of 2.7 eV which makes it is capable of the visible adsorption.3–6 As a photocatalyst, it has been widely used in water splitting,7,8 organic pollutants degradation,9,10 CO2 reduction,11 and other fields.12,13
Crystal violet (CV, C25H30ClN3, IUPAC name is N-[4-[bis][4-dimethyl-amino]-phenyl]methylene]-2,5-cyclohexadien-1-ylidine]-n-methylmethanaminium chloride), a typical triphenylmethane dye, is widely used in cell biology, paper, leather and textile industry.14–16 The wastewater containing CV is low biodegradability and high stability (complex aromatic structure) and it could be absorbed through the skin and causing the skin, eye, digestive irritation and even cancer.17–19 From the aspect of environmental safety and health of life, it is vital to develop an effective way to abate CV in wastewater. Various methods have been adopted for eliminating dye pollution from water, including chemical oxidation,20,21 photo-catalytic decomposition,22,23 electro-catalytic degradation24,25 and non-thermal plasma.26–28 These methods usually have some defects, such as slow degradation rate, complex, heavy expenses and usually causing secondary pollution. The adsorption technique is gaining more attention for it is high efficiency, simple design, cost-effective and adaptable.29–35 There have been various kinds of adsorbents developed, such as carbon materials,36–38 natural clay minerals,39–42 and bioadsorbents.43–45 However, certain deficiencies including costly, intricate pre-treatment and low adsorption capability limited the use of some certain adsorbents.46–53
Due to the conjugated region, stacking structure and the weak forces of g-C3N4, it has the potential for aggregating functional groups or materials to form nanocomposite with multiform favorable properties.54–56 This distinctive structure has drawn great interesting in improving the photocatalytic performance.57,58 But the research of using modified g-C3N4 as adsorbent for clearing up dye pollutant is rarely been reported.59,60 As far as we know, there has been no report of using g-C3N4-based composite as an adsorbent in dye wastewater treatment. Strontium carbonate (SrCO3), a typical alkaline earth metal carbonate, has been widely used as additives in industrial production.61–63 Meanwhile, some research has been reported on its adsorption performance attributed to its various architectures.64,65
In this work, we firstly induced SrCO3 to incorporate with g-C3N4 via one-step calcination method to fabricate a novel adsorbent SrCO3/g-C3N4. The morphology and structure of the composite was characterized by XRD, FT-IR, SEM, TEM, BET and XPS, and its adsorptive capacity and selectivity of CV in aqueous solution were investigated. To the best of our knowledge, this work represents the first example employing g-C3N4-based composite for selective adsorption of CV.
2 Experimental
2.1 Synthesis of SrCO3/g-C3N4
SrCO3, melamine and CV are all AR grade and purchased from Chengdu Kelong Chemical Agents (China), without any further purification. SrCO3/g-C3N4 was synthesized by one-step calcination process in a muffle furnace. SrCO3 and melamine (mass ratio = 1
:
1) were dissolved with deionized water in alumina crucible, and then the mixed solution was dispersion with ultrasonic irradiation for 20 min under ambient temperature. The final solution was transferred into muffle furnace and maintained at 600 °C for 4 hours at heating rate of 5 °C min−1 to obtain the prepared nanocomposite. SrCO3 and melamine were also treated as the forward route for making comparison.
2.2 Characterization
X-ray diffraction (XRD, Shimadzu, XRD-6100) patterns were detected with Cu Kα radiation (40 kV, 30 mA, 2θ = 10–80°) to investigate the crystal structures of the samples. Fourier transform infrared spectra (FT-IR, Shimadzu, IR Prestige-21), were recorded in the range of 4500–400 cm−1, using KBr technique to analyze the functional groups on the surface of the composite. Scanning electron microscope (SEM, JEOL, JSM-7800F) and Transmission Electron Microscopy (TEM, JEOL, JEM-2100) were used to observe the morphologies and the microstructures of the samples. The N2 adsorption apparatus (Micromeritics, ASAP 2020) were used to obtain the Brunauer–Emmett–Teller (BET) surface area of the samples. X-ray photoelectron spectroscopy (XPS, Thermo Scientific, ESCALAB 250xi) was used to determine the binding energy.
2.3 Adsorption experiments
The concentration of CV in the solution was determined at the maximum absorbance (λmax = 580 nm) by UV-vis spectrophotometer (UV-vis spectrometer, Tianmei, UV1102). For high concentration, the samples were diluted before measurements. And the initial pH of the dye solution was measured by pH meter (pH meter, Sartorius, PB10).
The adsorption experiments were conducted by a batch method. All experiments were conducted at 7.0 pH value, except those that investigated the effect of initial pH of dye solution. And 0.08 g adsorbent was dispersed in 80 mL CV solution, except that were used to study the effect of the dosage. Similarly, the initial concentration is 1600 mg L−1, except those that investigate the same parameters. The pH of the initial dye solution was adjusted with HCL (0.1 mol L−1) and NaOH (0.1 mol L−1). The kinetic experiments were carried out at the initial concentration of 500, 1000, 1200, 1400, and 1600 mg L−1. It performed on an air bathed shaker. The solution was separated by centrifugation at 5000 rpm for 5 min.
The adsorption capacity at time t qt (mg g−1) and removal rate (%) were calculated using the following equation:
|
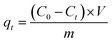 | (1) |
|
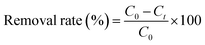 | (2) |
where
C0 and
Ct are the liquid-phase concentrations of the CV (mg L
−1) at initial time and time
t, respectively.
V is the volume of the solution (mL) and
m is the mass of the used adsorbent (mg).
3 Results and discussion
3.1 Characterization of the samples
The XRD patterns of the prepared samples are shown in Fig. 1. The g-C3N4 displayed a typical diffraction peaks at 27.46° and 12.96°, corresponding to the (002) and (100) diffraction planes, which represented the interlayer stacking reflection and in-plane structure of aromatic system, respectively.66,67 This indicated that the g-C3N4 was synthesized by polycondensation approach with pure melamine. Meanwhile, the observed diffraction peaks of calcined SrCO3 are located at 2θ angles = 25.28°, 25.91°, 29.73°, 31.62°, 34.64°, 35.22°, 36.64°, 41.42°, 44.18°, 45.74°, 46.68°, 47.80°, 50.03° which correspond to the planes of (111), (021), (002), (012), (102), (200), (130), (220), (221), (041), (202), (132), (113), respectively. It could be unambiguously indexed to the orthorhombic phase of SrCO3 (JCPDS card no. 05-0418).68,69 There is no observation of the typical diffraction of g-C3N4 in the synthesized composite, the diffraction peaks were mostly the same as the SrCO3, but the feature peaks positions of SrCO3 shifted slightly toward a lower diffraction angle. And the impure peaks of the composite might be the carbonization of the raw materials or the melamine did not condense completely.
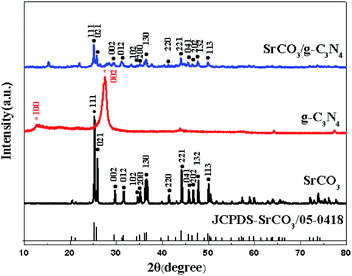 |
| Fig. 1 XRD patterns of the g-C3N4, SrCO3 and SrCO3/g-C3N4. | |
The FT-IR spectra of the prepared materials are shown in Fig. 2. For the calcined melamine, the typical adsorption peaks in the 1200–1700 cm−1 range and at 808 cm−1 of g-C3N4 could be observed. They are assigned to the typical skeletal stretching vibrations of armomatic C–N heterocycles and the out-of-plane bending vibration of tri-s-triazine rings, respectively.70,71 The peaks at 698, 858, 1070, 1458 and 1774 cm−1 corresponding to the CO32− of the calcined SrCO3 were observed.50 In case of the SrCO3/g-C3N4, the typical skeletal stretching vibrations of tri-s-triazine were hardly observed; it might be the adsorption intense of SrCO3 was so strong in order to impede the peaks of g-C3N4. Meanwhile, the peak at 808 cm−1 presented in g-C3N4 shifted to 821 cm−1, the peak at 856 cm−1 presented in SrCO3 shifted to 858 cm−1, the peaks at 1070 cm−1 and 1774 cm−1 were disappeared, these might due to the strong interactions between the carbonate of SrCO3 and tri-s-triazine rings of g-C3N4. Furthermore, the new peak at 2112 cm−1 corresponding to C
N groups appeared after calcination indicating that the incorporation of SrCO3 has intense interaction during the condense process of melamine.72
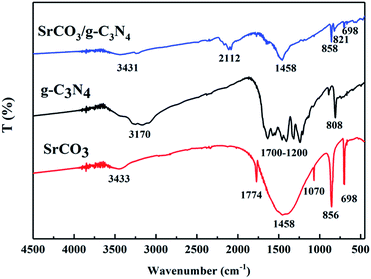 |
| Fig. 2 FT-IR spectra of the g-C3N4, SrCO3 and SrCO3/g-C3N4. | |
To make further investigation of the constitution of the prepared composite, the calcined product was treated with HCl (0.5 mol L−1) and deionized water, because g-C3N4 possessed fascinating acid stability. The treatment was terminated until the pH value was neutral, and then the treated nanocomposite was dried at 60 °C for 24 h. The XRD patterns and FT-IR spectra of the HCl-treated product are characterized in Fig. 3. The feature diffraction peak of g-C3N4, indicating the interlayer stacking, changed from 27.46° to 27.92° is explicitly observed in Fig. 3a. The change of the diffraction peak was corresponded to the stacking distance reduced from 0.325 to 0.319 nm. The above results implied that the interlayer stacking order was improved. And FT-IR spectra of the product were well matched with the pure g-C3N4 except the weak intensity (Fig. 3b). So, it could be indicated that the SrCO3/g-C3N4 was formed with the raw materials SrCO3 and melamine.
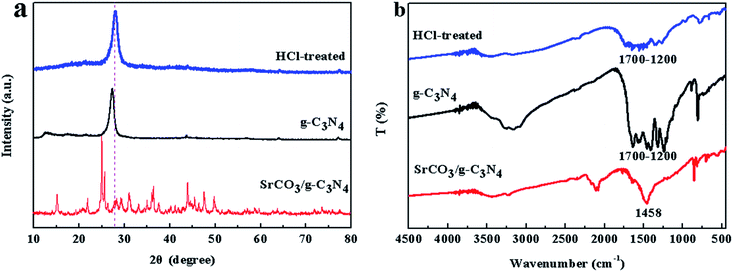 |
| Fig. 3 XRD patterns (a) and FT-IR spectra (b) of the HCl-treated SrCO3/g-C3N4. | |
SEM and TEM images of the calcined samples are displayed in Fig. 4. It revealed the morphology and microstructure of SrCO3/g-C3N4. In Fig. 4a, it could be seen the calcined melamine was predominantly composed of plate-like sheets,73 and the morphology of calcined SrCO3 was irregular polyhedrons with smooth surface is shown in Fig. 4b. In Fig. 4c, it could be seen clearly that the g-C3N4 covered on the SrCO3 incompletely. Further structural details of SrCO3/g-C3N4 are shown in Fig. 4d. There were some step edges of the layered g-C3N4 and rods-like SrCO3 with some parts overlapping. It might be the amount of g-C3N4 was not enough to disperse on the entire surface of SrCO3; and some areas cannot be wrapped by the g-C3N4. The SEM and TEM image could reveal that there existed some interactions between these two materials. Meanwhile, the specific surface areas of the clained samples were measured. As shown in Table 1, compared with g-C3N4 the composite BET surface area gets smaller with the addition of SrCO3, this might be the SrCO3 interacted with the stacking structure of g-C3N4. These results consistent with SEM results which could further prove that there have been some interactions between SrCO3 and g-C3N4.
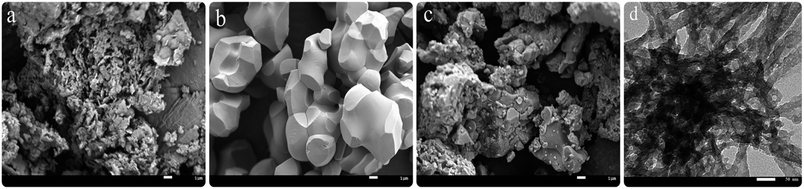 |
| Fig. 4 SEM images of the g-C3N4 (a), SrCO3 (b) and SrCO3/g-C3N4 (c); TEM images of the SrCO3/g-C3N4 (d). | |
Table 1 Textural properties of g-C3N4, SrCO3 and SrCO3/g-C3N4a
Sample |
SBET (m2 g−1) |
Vtotal (cm3 g−1) |
D (nm) |
SBET: BET surface area, Vtotal: total pore volume, D: average pore diameter calculated using BJH method. |
g-C3N4 |
27.4 |
0.126 |
17.5 |
SrCO3 |
1.93 |
0.003 |
8.72 |
SrCO3/g-C3N4 |
5.86 |
0.016 |
9.69 |
To further study the chemical state of SrCO3/g-C3N4, the XPS measurements were carried out. Fig. 5a is the general XPS spectra of calcined materials, indicating the presence of C, N, O and Sr in SrCO3/g-C3N4. The XPS spectra of C 1s are shown in Fig. 5b. For pristine g-C3N4 the peaks at 284.2 eV, 287.7 eV were corresponding to sp2-hybridized carbon in C–C group or the adventitious hydrocarbon74 and sp2-bond carbon in form of C–C
N.75 But after the blended calcinations, the peak at 284.2 eV shifted to 284.6 eV. The N 1s spectra are shown in Fig. 5c. The peak at 398.2 eV was attributed to sp2-hybridized nitrogen in N atom aromatic rings in form of C–N
C,76 and the nearly peaks at 399.3 eV and 401.0 eV were regarded as tertiary nitrogen (N-(C)3) and amino functional groups, respectively.77 After incorporation, the binding energy peaks slightly shifted. The peaks at 531.1 eV and 533.2 eV of O 1s spectra corresponding to the SrCO3 have no significant change in Fig. 5d. The XPS spectra of Sr 3d at 133.1 eV and 134.6 eV for calcined SrCO3 were observed in Fig. 5e. These peaks was corresponding to the Sr 3d5/2 and Sr 3d3/2, and the peak at 134.6 eV shifted to 134.7 eV after calcination. All these changes after co-calcination suggested that there were strong interactions between the two raw materials. Therefore, according to the analysis of XPS and the above results of XRD, FT-IR, SEM, TEM and BET measurements, it could be concluded that the SrCO3/g-C3N4 was synthesized using SrCO3 and melamine by one-step calcination.
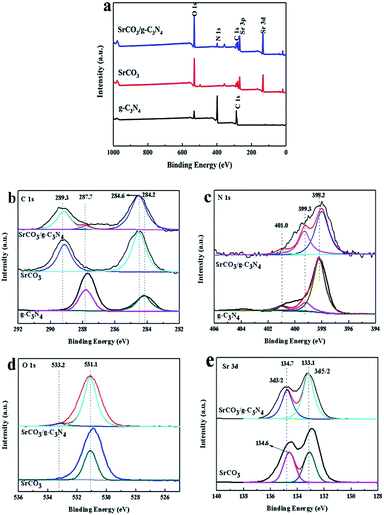 |
| Fig. 5 XPS survey spectra of g-C3N4, SrCO3 and SrCO3/g-C3N4 (a) and high-resolution XPS spectrum: C 1s (b), N 1s (c), O 1s (d) and Sr 3d (e). | |
3.2 The selective adsorption of CV
In our previous study, cationic dyes MB, RhB and CV were chosen as the target dye to conduct the adsorption experiments. We found that SrCO3/g-C3N4 appeared efficient adsorption capacity for CV except for the other dye solutions. To make further investigation of the selectivity of SrCO3/g-C3N4, mixed solutions of CV/MB, CV/RhB and CV/MB/RhB with equal volume ratio were prepared. The concentration of each dye solution was 10 mg L−1, and dosage ratio of SrCO3/g-C3N4 and mixed solutions was 1 g L−1. The adsorption experiments were carried out under ambient temperature and different pH value for 120 min.
The optical pictures and UV-vis measurements of the adsorption process are shown in Fig. 6. It could be apparently seen that CV was decolorized after the test (Fig. 6b), but in the MB and RhB groups the color slightly changed (Fig. 6a–c). In Fig. 6d, the color of CV/MB mixed solution was changed from dark blue to incipient blue (the color corresponding to the MB). For the CV/RhB group, the end color is very close to RhB (Fig. 6e). It suggested that CV in these two mixtures was selectively captured. However, the color in group CV/MB/RhB did not change, because the color of MB and RhB mixed solution is the same as that of CV. To further investigate the selective adsorption of CV, the UV-vis spectra were conducted for the tested groups. In the case of CV/MB, the UV-vis adsorption peaks of CV and MB were at 580 nm and 664 nm before adsorption, respectively. After adsorption, the peak of CV was dropped down drastically while the MB peak was almost unchanged (Fig. 6f). The same situation happened in the CV/RhB group (Fig. 6g). Although the optical of CV/MB/RhB group did not change, but the UV-vis adsorption curve appeared the same situation with the other two mixed groups (Fig. 6h). It could be concluded that the SrCO3/g-C3N4 performed selective adsorption of CV from CV/MB/RhB.
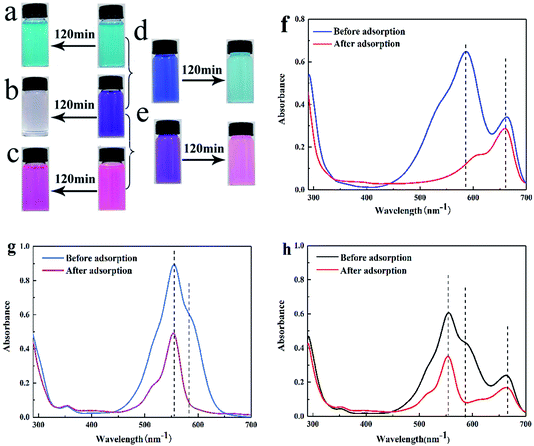 |
| Fig. 6 Optical photographs of single adsorption of MB (a), CV (b) and RhB (c); the selective adsorption of CV from CV/MB (d) and CV/RhB (e) mixture, UV-vis spectra of CV/MB (f),CV/RhB (g) and CV/MB/RhB (h) mixture before and after adsorption. | |
Furthermore, we also evaluated the effect of the initial pH of the dye solution. Fig. 7 shows the tested results. It could be seen that the initial pH (ranged from 4 to 10) of mixed dye solution did not significantly influence the adsorption process in each tested group.
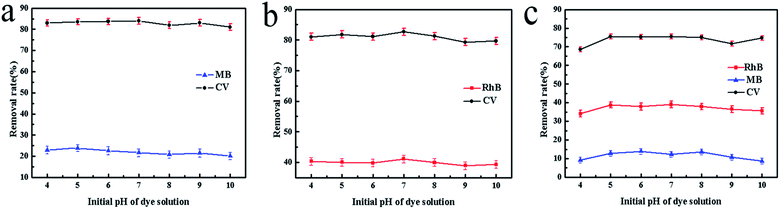 |
| Fig. 7 Effect of the initial pH of mixed dye solution CV/MB (a), CV/RhB (b) and CV/MB/RhB (c). | |
3.2.1 Effect of contact time. Fig. 8 shows the effect of different contact time (0–160 min) in the adsorption process. The initial concentration of CV is 1600, 1800 and 2000 mg L−1 and the dosage ratio of SrCO3/g-C3N4 and CV solution was 1 g L−1. For all concentrations, the removal rate of CV became constant after 120 min. Meanwhile, the group of 1600 mg L−1 reached the equilibrium firstly and the removal rate was up to the other concentration. So, the contact time of 120 min and the initial concentration of CV of 1600 mg L−1 were chosen for the following investigation.
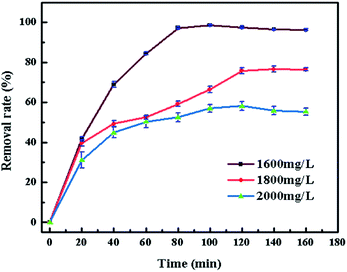 |
| Fig. 8 Effect of contact time. | |
3.2.2 Effect of initial concentration of CV. The effect of initial concentration (50–2000 mg L−1) of CV is shown in Fig. 9. From the obtained results, it was found that the removal efficiency kept steadily before the initial dye concentration up to 1800 mg L−1. This might be attributed to the higher initial concentration offered more numbers of dye molecules that could contact easily with SrCO3/g-C3N4. However, the removal rate was reduced at higher initial concentration indicating the saturation of SrCO3/g-C3N4.
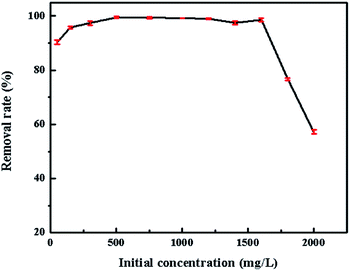 |
| Fig. 9 Effect of initial CV concentration. | |
3.2.3 Effect of adsorbent dosage. The dosage of the adsorbents is one of the major parameter which influences the adsorption process; an appropriate amount of adsorbents is in favor of the real industrial treatment. Fig. 10 shows the effect of the adsorbent dosage (0.25, 0.50, 0.75, 1.00, 1.25, 1.50 g L−1) of the CV. The removal rate increased with the increased amount of the adsorbent. When the dosage ups to 1.00 g L−1, the removal efficiency did not increase with the increasing dosage. It might due to the binding of almost CV ions onto SrCO3/g-C3N4, which made the equilibrium between solution and adsorbents. So the amount of adsorbent 1.00 g L−1 was the suitable dosage for the adsorption of CV.
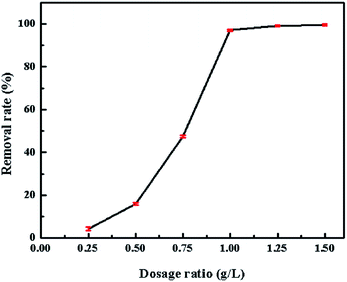 |
| Fig. 10 Effect of SrCO3/g-C3N4 dosage. | |
3.2.4 Effect of pH. The pH of initial dye solution is a key factor in adsorption process, which could influence the interaction between adsorbent and dye. In order to find out the pH effect, the adsorption test was conducted at initial solution pH ranged from 4 to 10. The results in Fig. 11 show that the initial pH of CV solution had little effect on the adsorption capacity. This observation could demonstrate that SrCO3/g-C3N4 is suitable for removing CV in wastewater in a wide pH range, and there is no need to adjust the initial solution pH before treatment.
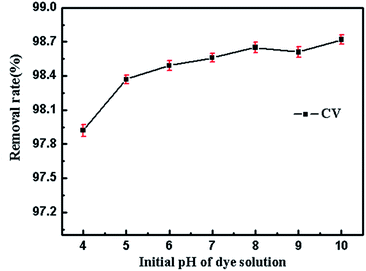 |
| Fig. 11 Effect of the initial pH of CV. | |
3.3 Adsorption kinetics
In order to study the characteristics of the adsorption process, pseudo-first-order and pseudo-second-order kinetics models were carried out with different concentration (500, 1000, 1200, 1600 mg L−1). The pseudo-first-order and pseudo-second-order are expressed by eqn (3) and (4).78 |
ln(qe − qt) = ln qe − k1t
| (3) |
|
 | (4) |
where k1 (min−1) and k2 (g (mg−1 min−1)) are the rate constant of pseudo-first-order and pseudo-second-order model, respectively; qe (mg g−1) and qt (mg g−1) are the adsorbed amount at equilibrium time and time t.
The rate constant of adsorption could be calculated from the slope of the plot in Fig. 12 and was listed in Table 2. R2 of pseudo-second-order model is much higher than the other, indicating that the pseudo-second order equation is more suitable to describe the CV adsorption with SrCO3/g-C3N4. So the chemisorptions or chemical sorption was the rate-controlling step for the adsorption process.79
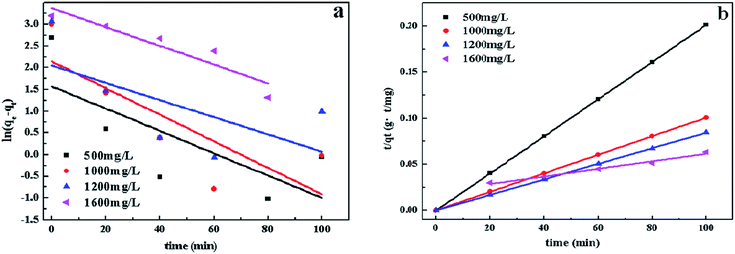 |
| Fig. 12 Pseudo-first-order (a), and pseudo-second-order (b) kinetic plots for adsorption. | |
Table 2 Kinetic parameters of different initial CV concentration
C0 (mg L−1) |
Pseudo-first-order |
Pseudo-second-order |
qe (cal) (mg g−1) |
k1 (min−1) |
R2 |
qe (exp) (mg g−1) |
qe (cal) (mg g−1) |
k2 (g (mg−1 min−1)) × 10−5 |
R2 |
1600 |
16.91 |
0.014 |
0.5357 |
1576.9 |
1666.7 |
3.67 |
0.9425 |
1800 |
28.33 |
0.016 |
0.7481 |
1378.6 |
1428.5 |
3.53 |
0.9577 |
2000 |
29.53 |
0.024 |
0.7822 |
1143.4 |
1250.0 |
8.10 |
0.9902 |
3.4 Desorption and reuse
In order to evaluate the reusability of SrCO3/g-C3N4, the regeneration process was performed. Adsorption experiment was conducted at the optimal condition as ascribed above in a 250 mL CV solution firstly, after equilibrium the used adsorbent was regenerated by centrifugation and washed several times with methanol and water at room temperature. Finally, the regenerated composite was dried for 24 h at 60 °C for reusing.
It could be seen that the removal rate gradually decreased after regeneration in Fig. 13. It might conclude that the adsorption mechanism of this process is not due to the physical adsorption.
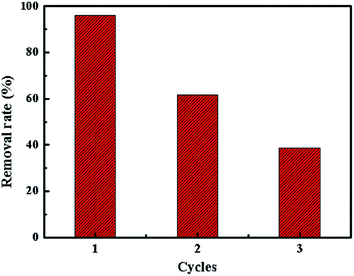 |
| Fig. 13 Reusability of the adsorbent. | |
3.5 Adsorption mechanism of crystal violet
To further study the adsorption performance of CV, the adsorption experiments were conducted with the calcined SrCO3, g-C3N4 and SrCO3/g-C3N4 firstly. 0.08 g tested materials were dispersed in 80 mL CV solution (10 mg L−1), respectively. After 2 h adsorption process, the removal rate of CV for tested materials is exhibited in Fig. 14. It could be seen obviously that the removal ability of g-C3N4 and SrCO3 to CV is much smaller than that of the nanocomposite. The removal rate of g-C3N4, SrCO3 and SrCO3/g-C3N4 were 31.30%, 17.30% and 97.46%, respectively. The adsorption mechanism of g-C3N4 might be attributed to π–π interaction between the g-C3N4 and CV, and that for the SrCO3, the electrostatic attraction between the carbonate generated from the hydrolytic of SrCO3 and the cationic chromogenic groups of CV. And for the SrCO3/g-C3N4, the synergistic effect of π–π interaction and electrostatic attraction highly improved the adsorption properties of the nanocomposite on CV.50 According to the above results and the crystal structures, we could deduce that superb adsorption capacity of CV might attribute to the synergistic interaction of the conjugation of g-C3N4 and the electrostatic attraction of SrCO3.
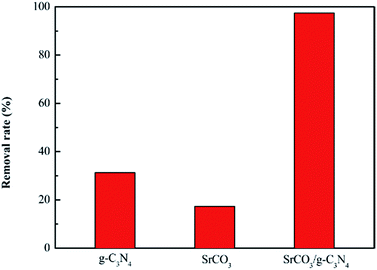 |
| Fig. 14 Comparisons of the adsorption effect of the SrCO3, g-C3N4, and SrCO3/g-C3N4. | |
Furthermore, the FT-IR spectra of SrCO3/g-C3N4 before and after CV adsorption were recorded. As shown in Fig. 15, the peak at 2112 cm−1 assigned to the nitrile groups shifted to the 2107 cm−1 and the peak at 1319 cm−1 assigned to aromatic C–N heterocycles observed clearly. These results indicate that the previously mentioned groups are involved in the adsorption process. Moreover, the intensity of the peak 1458 cm−1 corresponding to C–N heterocycle aromatic became stronger, that was for the CV was adsorbed onto the SrCO3/g-C3N4. All the changes of the FT-IR spectra of the SrCO3/g-C3N4 before and after adsorption could indicate that there have been intense interactions between dye molecules and SrCO3/g-C3N4. Furthermore, the result of desorption test might indicate that there has been intense chemical adsorption between the composite and CV. It could deduced that SrCO3/g-C3N4 performed selective adsorption of CV might due to the chemical structure of CV is more symmetrical and conjugation degree is higher than MB and RhB. On the basis of the above analysis, the conjugation and electrostatic interaction would be the driving force for the selective adsorption of CV.
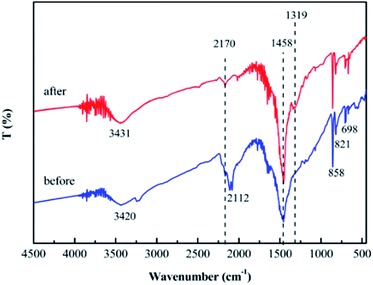 |
| Fig. 15 FT-IR spectra of SrCO3/g-C3N4 before and after adsorption. | |
4 Conclusions
In summary, a new SrCO3/g-C3N4 composite adsorbent was firstly synthesized with SrCO3 and melamine via a simple one-step calcination method. The SrCO3/g-C3N4 performed high and specific selective adsorption for CV. The experiment data was well described by the pseudo-second-order model. According to the crystal structural feature of SrCO3/g-C3N4 and the FT-IR analysis of SrCO3/g-C3N4 before and after adsorption of CV, it could be deduced that the synergies of π conjugation and electrostatic interaction would be the mechanism for the selective adsorption of CV. Our findings indicate that g-C3N4 based composite SrCO3/g-C3N4 could be a promising adsorbent which can potentially be applied for the removal of CV pollutants from aqueous solution.
Conflicts of interest
There are no conflicts to declare.
Acknowledgements
The authors are grateful for the financial support from the National Natural Science Foundation of China (No. 51778082, No. 21502012), Scientific and Technological Research Program of Chongqing Municipal Education Commission (No. KJ1500615, No. KJ130704), Chongqing University Postgraduates Innovation Project (No. CYB15039) and the Natural Science Foundation of Chongqing (No. cstc-2017shmsA20019, No. cstc2017jcyjAX0404).
Notes and references
- Z. W. Zhao, Y. J. Sun and F. Dong, Graphitic carbon nitride based nanocomposites: a review, Nanoscale, 2015, 7, 15–37 RSC.
- F. Ding, D. Yang, Z. W. Tong, Y. H. Nan, Y. J. Wang, X. Y. Zou and Z. Y. Jiang, Graphitic carbon nitride-based nanocomposites as visible-light driven photocatalysts for environmental purification, Environ. Sci.: Nano, 2017, 4, 1455–1469 RSC.
- X. C. Wang, K. Maeda, A. Thomas, K. Takanabe, G. Xin, J. M. Carlsson, K. Domen and M. Antonietti, A metal-free polymeric photocatalyst for hydrogen production from water under visible light, Nat. Mater., 2009, 8, 76–80 CrossRef CAS PubMed.
- A. Y. Shi, H. H. Li, S. Yin, B. Liu, J. C. Zhang and Y. H. Wang, Effect of conjugation degree and delocalized π-system on the photocatalytic activity of single layer g-C3N4, Appl. Catal., B, 2017, 218, 137–146 CrossRef CAS.
- M. Wu, J. M. Yan, X. W. Zhang and M. Zhao, Synthesis of g-C3N4 with heating acetic acid treated melamine and its photocatalytic activity for hydrogen evolution, Appl. Surf. Sci., 2015, 354, 196–200 CrossRef CAS.
- G. Mamba and A. K. Mishra, Graphitic carbon nitride (g-C3N4) nanocomposite: A new and exciting generation of visible light driven photocatalysts for environmental pollution remediation, Appl. Catal., B, 2016, 198, 347–377 CrossRef CAS.
- Y. Zheng, L. H. Lin, B. Wang and X. C. Wang, Graphitic carbon nitride polymers toward sustainable photoredox catalysis, Angew. Chem., Int. Ed., 2015, 54, 12868–12884 CrossRef CAS PubMed.
- J. Li, Y. C. Yin, E. Z. Liu, Y. N. Ma, J. Wan, J. Fan and X. Y. Hu, In situ growing Bi2MoO6 on g-C3N4 nanosheets with enhanced photocatalytic hydrogen evolution and disinfection of bacteria under visible light irradiation, J. Hazard. Mater., 2017, 321, 183–192 CrossRef CAS PubMed.
- K. Katsumata, R. Motoyoshi, N. Matsushita and K. Okada, Preparation of graphitic carbon nitride (g-C3N4)/WO3 composites and enhanced visible-light-driven photodegradation of acetaldehyde gas, J. Hazard. Mater., 2013, 260, 475–482 CrossRef CAS PubMed.
- J. F. Zhang, Y. F. Hu, X. L. Jiang, S. F. Chen, S. G. Meng and X. L. Fu, Design of a direct Z-scheme photocatalyst: Preparation and characterization of Bi2O3/g-C3N4 with high visible light activity, J. Hazard. Mater., 2014, 280, 713–722 CrossRef CAS PubMed.
- D. Masih, Y. Y. Ma and S. Rohani, Graphitic C3N4 based noble-metal-free photocatalyst systems: A review, Appl. Catal., B, 2017, 206, 556–588 CrossRef CAS.
- S. W. Liu, F. Chen, S. T. Li, X. X. Peng and Y. Xiong, Enhanced photocatalytic conversion of greenhouse gas CO2 into solar fuels over g-C3N4 nanotubes with decorated transparent ZIF-8 nanoclusters, Appl. Catal., B, 2017, 211, 1–10 CrossRef CAS.
- F. Wang, G. Wang, J. C. Zhang, B. Q. Li, J. Zhang, J. Deng, J. W. Chen and R. L. Wang, Novel sulfonated poly (ether ether ketone)/oxidized g-C3N4 composite membrane for vanadium redox flow battery applications, J. Electroanal. Chem., 2017, 797, 107–112 CrossRef CAS.
- A. Mittal, J. Mittal, A. Malviya, D. Kaur and V. K. Gupta, Adsorption of hazardous dye crystal violet from wastewater by waste materials, J. Colloid Interface Sci., 2010, 343, 463–473 CrossRef CAS PubMed.
- L. Y. Zhang, H. Y. Zhang, W. Guo and Y. Tian, Removal of malachite green and crystal violet cationic dyes from aqueous solution using activated sintering process red mud, Appl. Clay Sci., 2014, 93–94, 85–93 CrossRef CAS.
- Y. Y. Pei, M. Wang, D. Tian, X. F. Xu and L. J. Yuan, Synthesis of core–shell SiO2@MgO with flower like morphology for removal of crystal violet in water, J. Colloid Interface Sci., 2015, 453, 194–201 CrossRef CAS PubMed.
- V. Sabna, S. G. Thampi and S. Chandrakaran, Adsorption of crystal violet onto functionalized multi-walled carbon nanotubes: Equilibrium and kinetic studies, Ecotoxicol. Environ. Saf., 2016, 134, 390–397 CrossRef CAS PubMed.
- S. Charkraborty, S. Chowdhury and P. D. Saha, Adsorption of Crystal Violet from aqueous solution onto NaOH-modified rice husk, Carbohydr. Polym., 2011, 86, 1533–1541 CrossRef.
- R. Kumar and R. Ahmad, Biosorption of hazardous crystal violet dye from aqueous solution onto treated ginger waste (TGW), Desalination, 2011, 265, 112–118 CrossRef CAS.
- K. N. Vinod, Puttaswamy and K. N. Ninge Gowda, Oxidative decolorization of triphenylmethane dyes by chloramine-T in alkaline medium catalyzed by Pd(II): A comparative spectrophotometric kinetic and mechanistic approach, J. Mol. Catal. A: Chem., 2009, 298, 60–68 CrossRef CAS.
- J. Y. Yin, J. J. Cai, C. Yin, L. F. Gao and J. C. Zhou, Degradation performance of crystal violet over CuO@AC and CeO2–CuO@AC catalysts using microwave catalytic oxidation degradation method, J. Environ. Chem. Eng., 2016, 4, 958–964 CrossRef CAS.
- F. T. Chen, P. F. Fang, Y. P. Gao, Z. Liu, Y. Liu and Y. Q. Dai, Effective removal of high-chroma crystal violet over TiO2-based nanosheet by adsorption–photocatalytic degradation, Chem. Eng. J., 2012, 204–206, 107–113 CrossRef CAS.
- Y. R. Jiang, H. P. Lin, W. H. Chung, Y. M. Dai and C. C. Chen, Controlled hydrothermal synthesis of BiOxCly/BiOmIn composite exhibiting visible-light photocatalytic degradation of crystal violet, J. Hazard. Mater., 2015, 283, 787–805 CrossRef CAS PubMed.
- S. Popli and U. D. Patel, Mechanistic aspects of electro-catalytic reduction of Reactive Black 5 dye in a divided cell in the presence of silver nano-particles, Sep. Purif. Technol., 2017, 179, 494–503 CrossRef CAS.
- X. Y. Zhang, H. Q. Luo and N. B. Li, Crystal violet as an i-motif structure probe for reversible and label-free pH-driven electrochemical switch, Anal. Biochem., 2014, 455, 55–59 CrossRef CAS PubMed.
- A. Khataee, P. Gholami, B. Vahid and S. W. Joo, Heterogeneous sono-Fenton process using pyrite nanorods prepared by non-thermal plasma for degradation of an anthraquinone dye, Ultrason. Sonochem., 2016, 32, 357–370 CrossRef CAS PubMed.
- B. Jiang, J. T. Zheng, Q. Liu and M. B. Wu, Degradation of azo dye using non-thermal plasma advanced oxidation process in a circulatory airtight reactor system, Chem. Eng. J., 2012, 204–206, 32–39 CrossRef.
- J. Y. Chen, Y. L. Du, Z. J. Shen, S. S. Lu, K. Z. Su, S. J. Yuan, Z. H. Hu, A. Y. Zhang and J. W. Feng, Non-thermal plasma and BiPO4 induced degradation of aqueous crystal violet, Sep. Purif. Technol., 2017, 179, 135–144 CrossRef CAS.
- V. Nair, A. Panigrahy and R. Vinu, Development of novel chitosan–lignin composite for adsorption of dyes and metal ions from wastewater, Chem. Eng. J., 2014, 254, 491–502 CrossRef CAS.
- S. X. Yang, L. Y. Wang, X. D. Zhang, W. J. Yang and G. L. Song, Enhanced adsorption of Congo red dye by functionalized carbon nanotube/mixed metal oxides nanocomposite derived from layer doubled hydroxide precursor, Chem. Eng. J., 2015, 275, 315–321 CrossRef CAS.
- V. O. Njoku, K. Y. Foo, M. Asif and B. H. Hameed, Preparation of activated carbons from rambutan (Nepheliumlappaceum) peel by microwave-induced KOH activation for acid yellow 17 dye adsorption, Chem. Eng. J., 2014, 250, 198–204 CrossRef CAS.
- X. X. Liu, W. P. Gong, J. Luo, C. T. Zou, Y. Yang and S. J. Yang, Selective adsorption of cationic dyes from aqueous solution by polyoxometalate-based metal-organic framework composite, Appl. Surf. Sci., 2016, 362, 517–524 CrossRef CAS.
- S. Faradi, M. M. Amini, M. Dusek, M. Kucerakova and F. Mahmoudi, A new nanohybrid material constructed from Keggin-type polyoxometalate and Cd(II) semicarbazone
Schiff base complex with excellent adsorption properties for the removal of cationic dye pollutants, J. Mol. Struct., 2017, 1130, 592–602 CrossRef.
- Z. G. Jia, Z. Y. Li, T. Ni and S. B. Li, Adsorption of low-cost absorption materials based on biomass (Cortaderiaselloana flower spikes) for dye removal: Kinetics, isotherms and thermodynamic studies, J. Mol. Liq., 2017, 229, 285–292 CrossRef CAS.
- A. Bhattacharyya, D. Mondal, I. Roy, G. Sarkar, N. R. Saha, D. Rana, T. K. Ghosh, D. Mandal, M. Chakraborty and D. Chattopadhyay, Studies of the kinetics and mechanism of the removal process of proflavine dye through adsorption by grapheme oxide, J. Mol. Liq., 2017, 230, 696–704 CrossRef CAS.
- A. Regti, M. R. Laamari, S. E. Stiriba and M. E. Haddad, Use of response factorial design for process optimization of basic dye adsorption onto activated carbon derived from Persea species, Microchem. J., 2017, 130, 129–135 CrossRef CAS.
- D. H. Carrales-Alvarado, R. Ocampo-Perez, R. Leyva-Ramos and J. Rivera-Utrilla, Removal of the antibiotic metronidazole by adsorption on various carbon materials from aqueous phase, J. Colloid Interface Sci., 2014, 436, 276–285 CrossRef CAS PubMed.
- H. Li, N. H. An, G. Liu, J. L. Li, N. Liu, M. J. Jia, W. X. Zhang and X. L. Yuan, Adsorption behaviors of methyl orange dye on nitrogen-doped mesoporous carbon materials, J. Colloid Interface Sci., 2015, 455, 343–351 Search PubMed.
- L. Y. Zhang, H. Y. Zhang, W. Guo and Y. L. Tian, Removal of malachite green and crystal violet cationic dyes from aqueous solution using activated sintering process red mud, Appl. Clay Sci., 2014, 93–94, 85–93 CrossRef CAS.
- G. K. Sarma, S. S. Gupta and K. G. Bhattacharyya, Adsorption of Crystal violet on raw and acid-treated montmorillonite, K10, in aqueous suspension, J. Environ. Manage., 2016, 171, 1–10 CrossRef CAS PubMed.
- V. K. Gupta, S. Agarwal, A. Olgun, H. Demir, M. L. Yola and N. Atar, Adsorptive properties of molasses modified boron enrichment waste based nanoclay for removal of basic dyes, J. Ind. Eng. Chem., 2016, 34, 244–249 CrossRef CAS.
- R. L. Zhu, Q. Z. Chen, H. Y. Liu, F. Ge, L. F. Zhu, J. X. Zhu and H. P. He, Montmorillonite as a multifunctional adsorbent can simultaneously remove crystal violet, cetyltrimethylammonium, and 2-naphthol from water, Appl. Clay Sci., 2014, 88–89, 33–38 CrossRef CAS.
- Z. G. Jia, Z. Y. Li, T. Ni and S. B. Li, Adsorption of low-cost absorption materials based on biomass (Cortaderiaselloana flower spikes) for dye removal: Kinetics, isotherms and thermodynamic studies, J. Mol. Liq., 2017, 229, 285–292 CrossRef CAS.
- S. Shoukat, H. N. Bhatti, M. Iqbal and S. Noreen, Mango stone biocomposite preparation and application for crystal violet adsorption: A mechanistic study, Microporous Mesoporous Mater., 2017, 239, 180–189 CrossRef CAS.
- P. F. Sun, C. Hui, S. Wang, L. Wan and Y. H. Zhao, Bacillus amyloliquefaciens biofilm as a novel biosorbent for the removal of crystal violet from solution, Colloids Surf., B, 2016, 139, 164–170 CrossRef CAS PubMed.
- S. S. Lam, R. K. Liew, Y. M. Wong, P. N. Y. Yek, N. L. Ma, C. L. Lee and H. A. Chase, Microwave-assisted pyrolysis with chemical activation, an innovative method to convert orange peel into activated carbon with improved properties as dye adsorbent, J. Cleaner Prod., 2017, 162, 1376–1387 CrossRef CAS.
- E. Lakovleva, M. Sillanpaä, P. Maydannik, J. T. Liu, S. Allen, A. B. Albadarin and C. Mangwandi, Manufacturing of novel low-cost adsorbent: Co-granulation of limestone and coffee waste, J. Environ. Manage., 2017, 203, 853–860 CrossRef PubMed.
- R. P. Chicinaş, H. Bedelean, R. Stefan and A. Măicăneanu, Ability of a montmorillonitic clay to interact with cationic and anionic dyes in aqueous solutions, J. Mol. Struct., 2018, 1154, 187–195 CrossRef.
- P. S. Thue, A. C. Sophia, E. C. Lima, A. G. N. Wamba, W. S. de Alencar, G. S. dos Reis and F. S. Rodembusch, Synthesis and characterization of a novel organic-inorganic hybrid clay adsorbent for the removal of acid red 1 and acid green 25 from aqueous solutions, J. Cleaner Prod., 2018, 171, 30–44 CrossRef CAS.
- Y. Zheng, D. Y. Chen, N. J. Li, Q. F. Xu, H. Li, J. H. He and J. M. Lu, Highly efficient simultaneous adsorption and biodegradation of a highly-concentrated anionic dye by a high-surface-area carbon-based biocomposite, Chemosphere, 2017, 179, 139–147 CrossRef CAS PubMed.
- N. Tahir, H. N. Bhatti, M. Lqbal and S. Noreen, Biopolymers composites with peanut hull waste biomass and application for Crystal Violet adsorption, Int. J. Biol. Macromol., 2017, 94, 210–220 CrossRef CAS PubMed.
- S. Shoukat, H. N. Bhatti, M. Lqbal and S. Noreen, Mango stone biocomposite preparation and application for crystal violet adsorption: A mechanistic study, Microporous Mesoporous Mater., 2017, 239, 180–189 CrossRef CAS.
- N. Tahir, H. N. Bhatti, M. Iqbal and S. Noreen, Biopolymers composites with peanut hull waste biomass and application for Crystal Violet adsorption, Int. J. Biol. Macromol., 2017, 94, 210–220 CrossRef CAS PubMed.
- X. G. Cai, J. Y. He, L. Chen, Y. L. Li, K. S. Zhang, Z. Jin, J. Y. Liu, C. M. Wang, X. G. Wang and L. T. Kong, A 2D-g-C3N4 nanosheet as an eco-friendly adsorbent for various environmental pollutants in water, Chemosphere, 2017, 17, 192–201 CrossRef PubMed.
- X. R. Ding, J. Zhu, Y. Zhang, Q. Xia, W. T. Bi, X. D. Yang and J. F. Yang, Separation and concentration of natural products by fast forced adsorption using well-dispersed velvet-like graphitic carbon nitride with response surface methodology optimization, Talanta, 2016, 154, 119–126 CrossRef CAS PubMed.
- N. Bao, X. D. Hu, Q. Z. Zhang, X. H. Miao, X. Y. Jie and S. Zhou, Synthesis of porous carbon-doped g-C3N4 nanosheets with enhanced visible-light photocatalytic activity, Appl. Surf. Sci., 2017, 403, 682–690 CrossRef CAS.
- G. Mamba and A. K. Mishra, Graphitic carbon nitride (g-C3N4) nanocomposites: A new and exciting generation of visible light driven photocatalysts for environmental pollution remediation, Appl. Catal., B, 2016, 198, 347–377 CrossRef CAS.
- S. W. Cao, J. X. Low, J. G. Yu and M. Jaroniec, Polymeric photocatalysts based on graphitic carbon nitride, Adv. Mater., 2015, 27, 2150–2176 CrossRef CAS PubMed.
- G. Q. Tan, L. N. She, T. Liu, C. Xu, H. J. Ren and A. Xia, Ultrasonic chemical synthesis of hybrid mpg-C3N4/BiPO4 heterostructured photocatalysts with improved visible light photocatalytic activity, Appl. Catal., B, 2017, 207, 120–133 CrossRef CAS.
- C. Y. Liu, Y. H. Zhang, F. Dong, A. H. Reshak, L. Q. Ye, N. Pinna, C. Zeng, T. R. Zhang and H. W. Huang, Chlorine intercalation in graphitic carbon nitride for efficient photocatalysis, Appl. Catal., B, 2017, 203, 465–474 CrossRef CAS.
- X. B. He, F. X. Yin, J. N. Chen and C. Y. Ye, Co-SrCO3/N-doped carbon: a highly efficient hybrid electrocatalyst for the oxygen reduction reaction and Zn–air batteries, Inorg. Chem. Front., 2017, 4, 1073–1086 RSC.
- W. C. Zhu, G. L. Zhang, J. Li, Q. Zhang, X. L. Piao and S. L. Zhu, Hierarchical mesoporous SrCO3 submicron spheres derived from reaction-limited aggregation induced “rod-to-dumbbell-to-sphere” self-assembly, CrystEngComm, 2010, 12, 1795–1802 RSC.
- J. M. Du, Z. M. Liu, Z. H. Li, B. X. Han, Y. Huang and J. L. Zhang, Synthesis of mesoporous SrCO3 spheres and hollow CaCO3 spheres in room-temperature ionic liquid, Microporous Mesoporous Mater., 2005, 83, 145–149 CrossRef CAS.
- W. C. Zhu, Z. Z. Liang, X. F. Liu, H. Zhang, Y. J. Zheng, X. L. Piao and Q. Zhang, Soft-template self-assembly of hierarchical mesoporous SrCO3 by low-temperature hydrothermal route and their application as adsorbents for methylene blue and heavy metal ions, Powder Technol., 2012, 226, 165–172 CrossRef CAS.
- S. S. Wu, S. F. Yin, H. Q. Cao, Y. X. Lu, J. F. Yin and B. J. Li, Glucosan controlled biomineralization of SrCO3 complex nanostructures with superhydrophobicity and adsorption properties, J. Mater. Chem., 2011, 21, 8734–8741 RSC.
- X. Bai, L. Wang, R. Zong and Y. Zhu, Photocatalytic activity enhanced via g-C3N4 nanoplates to nanorods, J. Phys. Chem., 2013, 117, 9952–9961 CAS.
- W. N. Xing, C. M. Li, G. Chen, Z. H. Han, Y. S. Zhou, Y. D. Hu and Q. Q. Meng, Incorporating a novel metal-free interlayer into g-C3N4 frame work for efficiency enhanced photocatalytic H2 evolution activity, Appl. Catal., B, 2017, 203, 65–71 CrossRef CAS.
- S. B. Ni, W. L. Yang and T. Li, Hydrothermal synthesis and photoluminescence properties of SrCO3, Mater. Lett., 2011, 65, 766–768 CrossRef CAS.
- A. Tahmasian, V. Safarifard, A. Morsali and S. W. Joo, Sonochemical syntheses of a new fibrous-like nano-scale strontium(II) 3D coordination polymer; precursor for the fabrication of a strontium carbonate nanostructure, Polyhedron, 2014, 67, 81–88 CrossRef CAS.
- Z. F. Huang, J. J. Song, L. Pan, Z. M. Wang, X. Q. Zhang, J. J. Zou, W. B. Mi, X. W. Zhang and L. Wang, Carbon nitride with simultaneous porous network and O-doping for efficient solar-energy-driven hydrogen evolution, Nano Energy, 2015, 12, 646–659 CrossRef CAS.
- W. K. Jo and C. S. Selvam, Enhanced visible light-driven photocatalytic performance of ZnO-g-C3N4 coupled with graphene oxide as a novel ternary nanocomposite, J. Hazard. Mater., 2015, 299, 462–470 CrossRef CAS PubMed.
- D. M. Chen, J. J. Yang and H. Ding, Synthesis of nanoporous carbon nitride using calcium carbonate as templates with enhanced visible-light photocatalytic activity, Appl. Surf. Sci., 2017, 391, 384–391 CrossRef CAS.
- I. Papailias, T. Giannakopoulou, N. Todorova, D. Demotukali, T. Vaimakis and C. Trapalis, Effect of processing temperature on structure and photocatalytic properties of g-C3N4, Appl. Surf. Sci., 2015, 358, 278–286 CrossRef CAS.
- Y. X. Zhang, J. Wu, Y. Y. Deng, Y. J. Xin, H. L. Liu, D. Ma and N. Bo, Synthesis and visible-light photocatalytic property of Ag/GO/g-C3N4 ternary composite, J. Mater. Sci. Eng. B, 2017, 211, 1–9 Search PubMed.
- M. Wu, J. M. Yan, X. N. Tang, M. Zhao and Q. Jiang, Synthesis of potassium-modified graphitic carbon nitride with high photocatalytic activity for hydrogen evolution, ChemSusChem, 2014, 7, 2654–2658 CrossRef CAS PubMed.
- M. Wu, J. M. Yan, X. W. Zhang and M. Zhao, Synthesis of g-C3N4 with heating acetic acid treated melamine and its photocatalytic activity for hydrogen evolution, Appl. Surf. Sci., 2015, 354(44), 195–200 Search PubMed.
- J. L. Lv, K. Dai, J. F. Zhang, Q. Liu, C. H. Liang and G. P. Zhu, Facile constructing novel 2D porous g-C3N4/BiOBr hybrid with enhanced visible-light-driven photocatalytic activity, Sep. Purif. Technol., 2017, 178, 6–17 CrossRef CAS.
- S. Álvarez-Torrellas, R. S. Ribeiro, H. T. Gomes, G. Ovejero and J. García, Removal of antibiotic compounds by adsorption using glycerol-based carbon materials, Chem. Eng. J., 2016, 295, 277–288 CrossRef.
- Y. S. Ho and G. McKay, Pseudo-second order model for sorption processes, Process Biochem., 1999, 34, 451–465 CrossRef CAS.
|
This journal is © The Royal Society of Chemistry 2018 |
Click here to see how this site uses Cookies. View our privacy policy here.