DOI:
10.1039/C7RA10290A
(Paper)
RSC Adv., 2018,
8, 13274-13283
Two azobenzene derivatives CAB/ACB as reusable sunscreen: UV absorptive capacity and biosafety evaluation†
Received
16th September 2017
, Accepted 19th March 2018
First published on 10th April 2018
Abstract
Exposure to UV radiation may cause harmful effects to the skin such as damage, aging and cancer, which can be prevented by using sunscreens. Here, two azobenzene derivatives, 4-cholesterocarbonyl-4′-(N,N,N-triethylaminebutyloxyl bromide) azobenzene (CAB) and 4-cholesterocarbonyl-4′-(N,N-diethylaminebutyloxyl) azobenzene (ACB) were studied as reusable sunscreen candidates. Biocompatibility studies including apoptosis, cytotoxicity and in vivo phototoxicity revealed that the two compounds were rather safe, except ACB, which showed a weak phototoxicity in vitro. Both CAB and ACB have good UV absorption capacity not only in their solution state (dimethylsulfoxide, DMSO) but also in the cosmetic cream state. A commercial sunscreen, avobenzone was decomposed upon UV irradiation and lost its UV protection ability, while that of CAB and ACB could be quickly recovered upon visible light irradiation, implying that they can serve as a new type of reusable sunscreen.
1. Introduction
As the ozone layer continues to suffer damage, the intensity of ultraviolet (UV) radiation gradually increases. Approximately 44.3% of the solar radiation reaching the earth is visible radiation from 400 to 760 nm, the remaining 49.5% is due to infrared radiation at 760 to 5000 nm and only 6.2% is attributed to UV radiation at 100 to 400 nm.1 UV radiation can be divided into three categories: 200–280 nm short-wave ultraviolet (UVC), 280–320 nm medium wavelength ultraviolet (UVB) and 320–400 nm long wavelength ultraviolet (UVA). Various chromophores in the skin, such as melanin, DNA, RNA, proteins and lipids can absorb UV light, causing photochemical reactions and producing reactive oxygen species (ROS).2 UVC can be completely absorbed by the atmosphere, while UVA and UVB can penetrate the atmosphere and are responsible for inducing the skin pigmentation, horny growth, photo aging and photocarcinogenesis.3 In addition, excessive UVA irradiation can promote the formation of wrinkle and cause ocular diseases.4,5 Therefore, development of efficient sunscreens to reduce the occurrence of such skin diseases has attracted much more attention.
The traditional chemical sunscreens can be listed as para-aminobenzoic acid (PABA), octocrylene, salicylates, anthranilates, and cinnamates.6 tert-Buty-lmethoxydibenzoylmethane (also known as avobenzene, Parsol 1789, etc.) is one of the most common UVA filters in commercial sunscreens with high UV absorption capacity, but it is easy to be decomposed by light.7 Azobenzene derivatives are one of the most widely used photosensitive compounds which can convert from trans-state to cis-state by absorbing UV light and restore to a steady trans-state under visible light irradiation.8–12 Azobenzene owns a stable chemical structure against UV irradiation without photodecomposition. Azobenzene-modified biomolecules can be used to exert spatiotemporal control over a variety of molecular signaling processes in vivo.13–15 However, the biosafety of azo groups needs to be considered when they are used as cosmetic.16,17 Especially, compared with maintenance cosmetics, the phototoxicity of sunscreen is always a necessary test indicator.18–20 Therefore, in addition to the sun protection efficiency, the biosafety including phototoxicity of azobenzene compounds based sunscreen is necessary to be evaluated both in vitro and in vivo.21
Two kinds of photosensitive azobenzene derivatives, 4-cholesterocarbonyl-4′-(N,N,N-triethylaminebutyloxyl bromide) azobenzene (CAB) and 4-cholesterocarbonyl-4′-(N,N-diethylaminebutyloxyl) azobenzene (ACB) were synthesized by our group.10,22 Their chemical structures are shown in Scheme 1. The potential application of ACB in liposomal system as a sunscreen has been evaluated preliminarily,9,23 either UVA or UVB protective effects as indicated by the critical wavelength method and the ultraviolet spectrophotometer method separately was observed. In this study, we compared the multiple indicators of the two azobenzene compounds, CAB and ACB to determine which one is better candidate as sunscreen. Firstly, the UV absorption capacities of CAB and ACB were evaluated by the molar absorption coefficient and photometric ratio. Then, interaction with DNA extracted from NIH 3T3 cells, and ROS formation were analyzed and compared. Finally, the evaluation of skin phototoxicity of the two azo compounds using guinea pigs and the protection abilities against UV irradiation using mice were carried out in vivo.
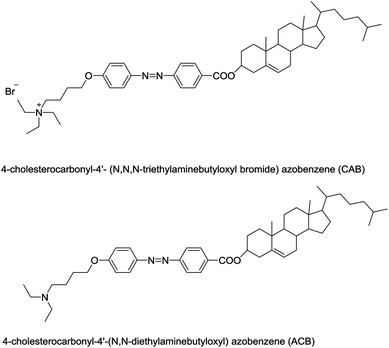 |
| Scheme 1 Structures of CAB and ACB. | |
2. Materials and methods
2.1 Materials
2,7-Dichlorodi-hydrofluorescein diacetate (DCFH-DA) was purchased from Bu Bai Chemical Technology Co., Ltd. (Shanghai, China). 4-tert-Buty-4′-methoxydibenzoylmethane (also known as avobenzene, Parsol 1789, etc.) was purchased from King Jack Int., Hong Kong. The cream substrate was a kind gift from Department of Dermatology, Huashan Hospital (Shanghai, China). Neutral Red (NR) was purchased from Shanghai Source Leaves Biological Co., Ltd. (Shanghai, China). 8-Methoxypsoralen was purchased from Sigma (St Louis, MO, USA). All other regents used were of analytical grade. The synthetic routes of CAB and ACB have been described by our group (Scheme 1).11,22
2.2 Molar absorptivity of CAB and ACB
CAB, ACB and avobenzone (AVO) were dissolved in DMSO at the concentration of 50 μM, respectively. Their UV absorption spectra were detected by UV spectrophotometer (U-3010, Hitachi, Japan). The molar absorptivities of CAB, ACB and AVO in DMSO solution were calculated according to the Lambert–Beer law: molar absorptivity coefficient ε (L mol−1 cm−1) = A/bc, where A is the absorbance at the wavelength of maximum absorbance (λmax), b is the path length, and c is the concentration.
2.3 Photoisomerization of CAB and ACB
Photostability is consider to be a key parameter for evaluation of effectiveness of commercial sunscreen products. The azobenzene derivatives in DMSO have been stood overnight in the dark to ensure the cis isomer in negligible amounts, the absorbance at 365 nm was as (Abs365 nm)dark. 1H spectra on a 400 MHz NMR Spectrometer (Agilent, USA) were used to evaluate the amount of cis and trans isomers in photostationary state before irradiation, after irradiation using 365 nm UVA and then by heating at 37 °C overnight. Moreover, CAB, ACB and AVO either dissolved in DMSO or cream were irradiated by a 365 nm light emitting diode (LED, M365L2, Thorlabs, USA) to achieve complete trans-to-cis isomerization. After that, they were irradiated by 420 nm LED (M420L3, Thorlabs, USA) to induce cis-to-trans isomerization. The UV-Vis spectrum of the samples after various periods of illumination was measured. And the reversible isomerization degree was measured according to the following formula: %transsample = [(Abs365 nm)sample/(Abs365 nm)dark] × 100, where (Abs365 nm)sample/(Abs365 nm)dark was the absorbance of the sample at wavelength 365 nm after irradiation/dark.
2.4 Interaction of DNA with CAB or ACB
Mouse NIH/3T3 fibroblasts were purchased from Cell Bank in Shanghai Institute of Cell Biology, Chinese Academy of Sciences (Shanghai, China). NIH 3T3 cells were cultured in DMEM medium supplemented with 10% heat-inactivated new born calf serum under humidified conditions at 37 °C and 5% CO2. The cells were seeded in a 12-well plate at a density of 2.5 × 105 cells per mL. After 24 h incubation, the culture medium of each well was replaced with medium containing CAB or ACB at the concentration of 0, 20, 40, 80 and 100 μg mL−1 for 2 h. Then DNA of cultured cells was extracted with Apoptosis DNA Ladder Rapid Extraction Kit (Bioteke Corporation, China). DNA damage caused by CAB or ACB was observed by analyzing band change using agarose gel electrophoresis. 500 μM H2O2 for 24 h incubation was used as the positive control. Briefly, the isolated DNA was mixed with the loading buffer (6×) and loaded on a 2% agarose gel. The voltage was set at 40 V. The NIH 3T3 cells treated with 100 μg mL−1 CAB or ACB for 0, 2, 4, 8, 12 or 24 h were also studied with the same procedure.
2.5 Effects of CAB or ACB on apoptosis of NIH 3T3 cells
The effect of CAB or ACB on the apoptosis of NIH 3T3 cells was detected by TUNEL method. The cells were seeded and cultured as described in Section 2.4. After treated with 100 μg mL−1 CAB or ACB for 0, 4, 8 and 24 h, the cells were washed twice with PBS at 37 °C. The freshly prepared 4% formaldehyde solution was added and incubated at room temperature for 1 h. Apoptosis was detected by the In Situ Cell Death Detection Kit (Roche, Switzerland), and the procedure was carried out according to the requirements of the kit. Fluorescence microscope (IX71, Olympus, Japan) was used to observe apoptosis.
2.6 Effect of CAB or ACB on UVA induced ROS in NIH 3T3 cells
NIH 3T3 cells were seeded and cultured as described above. After 24 h incubation, the culture medium of each well was replaced with medium containing CAB or ACB at the concentration of 0, 25, 50, 100 and 200 μM. Then the cells were irradiated by 365 nm LED at affluence rate of 1.5 mW cm−2. 500 μM H2O2 for 5 min incubation was used as the positive control. After that, 100 μL DCFH-DA (50 μM in PBS) was added to each well for 30 min. The 488 nm Ar/ArKr laser at 18 mW was used to excite the samples and the fluorescence centered at 520 nm was recorded for further analysis using a spectrophotometer (EnSpire2300, PerkinElmer, USA).
2.7 In vitro phototoxicity test of CAB or ACB
In vitro NIH 3T3 neutral red uptake (NRU) phototoxicity test was carried out as described in the OECD 432 guideline with minor modifications (OECD, 2004). Briefly, NIH 3T3 cells were seeded in a 96-well plate and incubated for 24 h to form monolayers. Then the culture medium was removed, and the medium containing different concentrations of CAB or ACB was added. After 1 h incubation, the cells were either subjected to UVA irradiation of 5 J cm−2 (+Irr) or not (−Irr) followed by washing twice with PBS, and added fresh medium for another 24 h incubation. After that, the medium containing 50 μg mL−1 Neutral Red (NR) was used to further incubate cells for 3 h. Then the NR medium was removed and the cells were lysed with NR desorbing solution (water
:
ethanol
:
acetic acid = 49
:
50
:
1). The absorption was measured at 540 nm by a spectrophotometer (EnSpire2300, PerkinElmer, USA). The photo-irritation-factor (PIF) was calculated using the following formula: PIF = IC50(−Irr)/IC50(+Irr). Anthracene was used as the positive control in this experiment.
2.8 In vivo phototoxicity test of CAB or ACB
British albino guinea pigs weighing between 239 and 284 g were used in this study. They were purchased from Shanghai Sheng Wang Experimental Animal Culture Co., Ltd. (Shanghai, China) and kept at 19–26 °C with relative humidity of 40–70%. Animal experiments were performed according to the Health Ministry of People's Republic of China Hygienic Standard for Cosmetics (2007). Four test sites (4 cm2) were selected on the back of each albino guinea pigs. The UV source for the test was a light therapy instrument (SS-03AB, Sigma, China) which had affluence rate of 8.6 mW cm−2 and the total UVA irradiation received by each sample was 10 J cm.−2 The test samples were applied at an amount of 1 mg mL−1 CAB or ACB. The irradiation time was 20 min at a distance of 25 cm from the test sites. The positive control group 8-methoxypsoralen (8-MOP, Sigma, USA) was administered in the same method. Photo-damage grade was calculated by using evaluation parameters describe in Table 1, adapted from Hygienic Standard for Cosmetics (2007).
Table 1 Grading scale for evaluation of skin phototoxicity24
Grading |
Evaluation criteria |
Erythema |
Edema |
0 |
No |
No |
1 |
Slight (bare visible) |
Slight (bare visible) |
2 |
Moderate (visible) |
Moderate (apparent swelling edge higher than surrounding skin) |
3 |
Severe |
Severe (1 mm of swelling and clear contour) |
4 |
Purple erythema to mild eschar |
Extremely severe (over 1 mm of swelling or ulceration) |
2.9 In vivo UV protective efficacy test of CAB or ACB
Many methods have been used to estimate the UV protection ability of sunscreens in vivo.25 Here, SPF (specific pathogen free) female Kunming mice (20–25 g), aged about 10 weeks, were purchased from Shanghai Jiesi Experimental Animal Co., Ltd. China. All animal experiments were approved by the Institutional Animal Care and Use Committee (IACUC) of Shanghai Jiaotong University (Shanghai, China). A light emitting diode (M365L2, Thorlabs, USA) with a dose of 172.8 J cm−2 was used to irradiate the dorsal region after removing hair. To compare UV protective efficacy and photostability characteristics of CAB, ACB and AVO, the reversible cis–trans isomerism characteristics of azobenzene compounds were performed. 1 cm2 skin of mouse was treated as described in Table 2. Erythema and edema were observed every day after the irradiation. Skin samples were taken (1 cm2) as frozen sections for subsequent histopathological examination after hematoxylin-eosin (HE) staining (IX71, Olympus, Japan).
Table 2 Treatment groups of UV protection
Group |
Treatment schedule |
1 |
No treatment, as the normal control |
2 |
UV irradiation exposure of 172.8 J cm−2, as the negative control |
3 |
Treated with 2 mg cream at UV exposure of 172.8 J cm−2 |
4 |
Treated with 2 mg cream with 0.06 mg CAB at UV exposure of 172.8 J cm−2 |
5 |
Treated with 2 mg cream with 0.06 mg ACB at UV exposure of 172.8 J cm−2 |
6 |
Treated with 2 mg cream with 0.06 mg AVO at UV exposure of 172.8 J cm−2 |
7 |
On the basic of group 4, irradiated with 420 nm LED for 20 min and then at UV exposure of 81.4 J cm−2 |
8 |
On the basic of group 5, irradiated with 420 nm LED for 20 min and then at UV exposure of 81.4 J cm−2 |
9 |
On the basic of group 6, irradiated with 420 nm LED for 20 min and then at UV exposure of 81.4 J cm−2 |
3. Results and discussion
3.1 Molar absorptivity of CAB/ACB
Molar absorptivity coefficient is considered to be a key parameter for evaluation of UV absorption capacity of commercial sunscreen products. The greater the coefficient is, the stronger the ability to protect.26 As seen in Table 3, the molar absorptivity coefficient of CAB in DMSO was 20
840 L mol−1 cm−1 at the maximum absorption peak (λmax) of 363.5 nm, and that of ACB was 21
480 L mol−1 cm−1 at λmax = 363.0 nm. Although both the molar absorptivities of CAB and ACB were lower than that of AVO (37
540 L mol−1 cm−1), yet compared with some general sunscreen chemicals such as p-aminobenzoic, dioxybenzone and oxybenzone, which have the molar absorptivities between 7600 L mol−1 cm−1 to 18
900 L mol−1 cm−1,27 both CAB and ACB may be considered as good candidates for sunscreens.
Table 3 The molar absorptivities of CAB, ACB and AVO in DMSO
Compound |
Solvent |
λmax (nm) |
C (mM) |
E (L mol −1 cm−1) |
CAB |
DMSO |
363.5 |
0.05 |
20 840 |
ACB |
DMSO |
363.0 |
0.05 |
21 480 |
AVO |
DMSO |
364.5 |
0.05 |
37 540 |
3.2 Photoisomerization of CAB and ACB
It is well known that azobenzene compounds can undergo reversible trans–cis isomerization when exposure to UV and visible light irradiation or thermal relaxation.28 On contrary, AVO exhibits irreversible structural change such as chemical photo-oxidation or photodegradation upon UV irradiation.29 The azo compound is always the mixture of trans and cis isomers in a photostationary state (PSS) due to the overlap of the n–π* transition. In order to keep the cis isomer in negligible amounts, the azobenzene compounds in DMSO were stored in the dark overnight before experiment. 1H NMR measurements suggested that the PPS of CAB in DMSO was composed of 99% trans isomer and 1% cis isomer, and ACB was composed of 92.1% trans isomer and 7.9% cis isomer, respectively (Fig. S1†). Due to the limited solubility of the two compounds in DMSO and the cis form of two compounds in the PSS was less than 10%, we used their UV-Vis spectra for evaluating the amount of the trans isomer percentage in solution. The trans–cis spectral change of ACB, CAB and AVO in DMSO or cream under 365/420 nm irradiation were shown in Fig. S2 and S3,† respectively. The trans-to-cis or cis-to-trans isomerization degree was represented as the percentage of trans-sample (% transsample) after 365 nm or 420 nm light irradiation as shown in Fig. S4.†
In the solution state, about 90% trans-ACB/CAB was converted to the cis form after UV irradiation; and the reversible cis-to-trans for ACB and CAB was about 80%, respectively. In addition, the cis-to-trans isomerization degree for ACB/CAB was about 70% in the cream state (Fig. S4†). As for AVO, the trans-to-cis isomerization could happen in DMSO, but the isomerization degree was not as high as both ACB and CAB. Moreover, AVO lost the ability of recovery due to the photodegradation in cream (Fig. S3F†). All chemical sunscreens are designed for one-time use because they will decompose and gradually lose their protective effect due to their instability against UV light.30 As a conclusion, CAB and ACB can absorb UV light repeatedly by reversible conformation change of the trans–cis isomerization, which provides the chemical structural basis for their special application as reusable sunscreen cosmetics.
3.3 Effects of CAB or ACB on DNA structure of NIH 3T3 cells
Except for high molar absorption coefficient and good stability, the safety of the sunscreen, especially the possible damage to DNA requires further investigation. It is well known that physical and chemical mutagen breaks double-strands of DNA which reduce its strand molecular weight. This can be determined by using agarose gel electrophoresis. As can be seen from Fig. 1, DNA extracted from NIH 3T3 cells which was treated with different concentrations of azo compounds showed only one band on agarose gel electrophoresis (Fig. 1A and B). The two azobenzene compounds did not induce changes in DNA ladder pattern as seen in apoptosis or shearing of DNA by H2O2 (positive control).31–33 Compared with the blank control group, there was no significant difference even when the concentration increased to 100 μg mL−1. Long time treatment with 100 μg mL−1 CAB or ACB for 24 h also gave no detectable dispersing (or nicking) band of DNA. The results showed that both CAB and ACB did not induce DNA damage of NIH 3T3 cells (Fig. 1C and D), implying safety of the two azobenzene compounds.
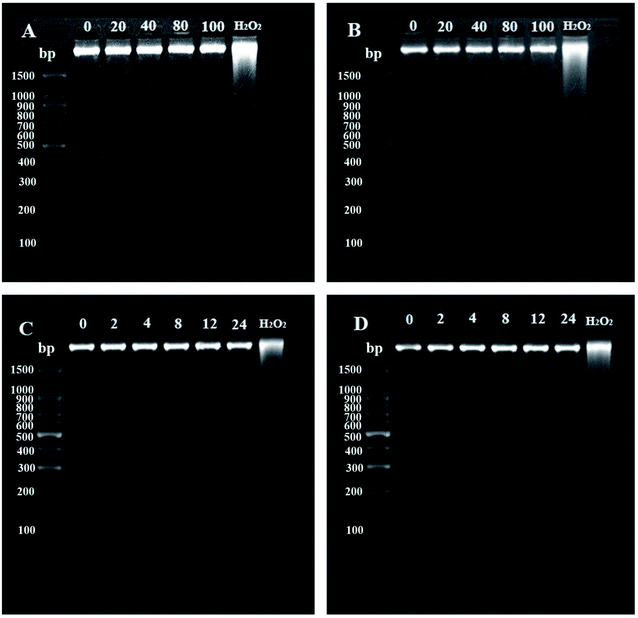 |
| Fig. 1 The agarose gel electrophoresis of DNA extracted from NIH 3T3 cells when treated with different concentrations (A and B) and times (C and D). CAB (A and C) and ACB (B and D), H2O2 served as the positive control. | |
3.4 Apoptosis induced by CAB or ACB
Detection of DNA fragments in situ using the terminal deoxyribonucleotidyl transferase (TdT)-mediated biotin-16-d UTP nick-end labelling (TUNEL) assay is much sensitive method to investigate apoptosis compared with agarose gel electrophoresis.34 As shown in Fig. 2, no obvious increase of apoptosis-related fluorescence was observed for chromatin condensation and apoptotic bodies with incubation time. Most NIH 3T3 cells maintained the same morphology (spindle shaped) as the control group when treated with CAB or ACB, while a very small of apoptotic cells with red nuclei and fragmented morphology were observed (Fig. 2). Thus, combining with the electrophoretic data, it can be proposed that both CAB and ACB have no damaging effect on DNA.
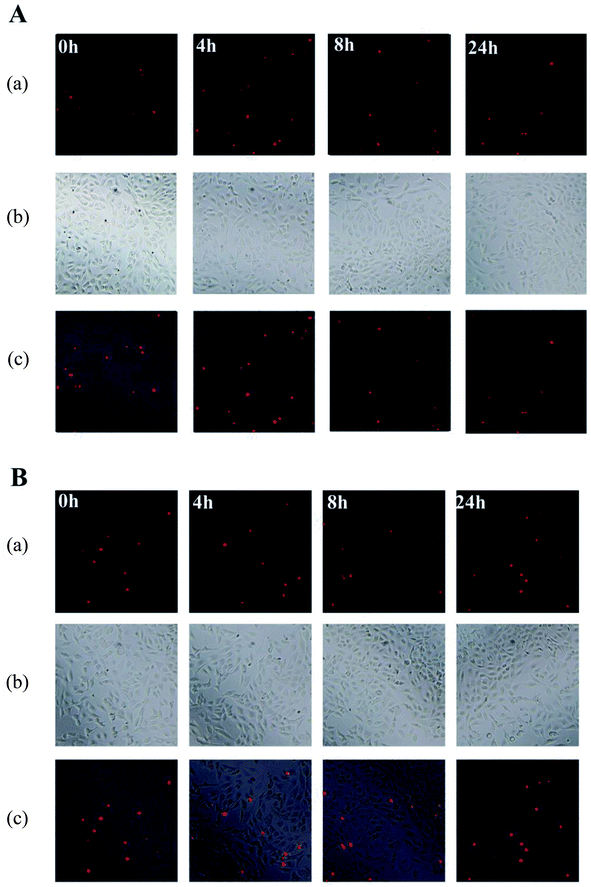 |
| Fig. 2 Apoptosis evaluation of NIH 3T3 cells induced by CAB (A) and ACB (B). (a) Fluorescence microscopy images; (b) phase contrast microscopy images; (c) merge of (a) with (b). | |
3.5 Effect of CAB or ACB on UVA induced ROS
ROS produced by UV irradiation in cutaneous tissues can cause DNA damage and induce apoptosis of cells. In order to study if the azobenzene compounds can affect UV-induced ROS production, DCFH-DA was used to quantify the amount of intracellular ROS by detecting the fluorescence intensity of DCF.35 The ROS level induced by UVA was close to the positive control H2O2, so the model of UV-induced ROS production can be established. After pretreated NIH 3T3 cells with different concentrations of CAB or ACB, UVA induced ROS was in a dose-dependent manner as indicated by change in fluorescence intensity of DCF, and decreased to the level of the blank control group at the concentration of 200 μg mL−1. Fig. 3 clearly showed the intracellular DCF fluorescence after pretreated by the two azobenzene compounds and then upon UVA irradiation, which indicated that UVA might be absorbed by either CAB or ACB effectively to weaken the UVA induced ROS production.
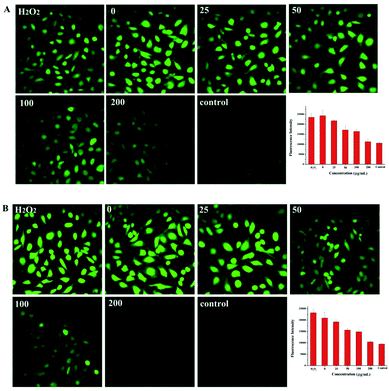 |
| Fig. 3 The fluorescent images and histogram of ROS in NIH 3T3 cells induced by UVA irradiation after treated with different concentrations (μM) of CAB (A) or ACB (B). Cells without UVA irradiation was used as the blank control and H2O2 served as the positive control. | |
3.6 In vitro phototoxicity: 3T3 neutral red uptake (NRU) assay
3T3 NRU test has the advantages of simple operation, low cost, good reproducibility and short test period.36 It has been proved that both cis-CAB and cis-ACB were not cytotoxic (Fig. S6†). To further analyze whether the two azobenzene compounds are phototoxic or not, the concentration response should be obtained in the presence and absence of irradiation. Anthracene was used as the positive control. Cell viability treated with anthracene was high in the absence of UV radiation, and there was no significant cytotoxicity even when the concentration was up to 120 μg mL−1 (Fig. 4A). However, after UV irradiation, the cell viability decreased to about 10%, even though the concentration was only 2.0 μg mL−1 (Fig. 4A). The PIF value of anthracene was calculated to be greater than 240, indicating an obvious phototoxicity. Thus, the model of NIH 3T3 cells neutral red uptake assay could be used for phototoxicity evaluation.
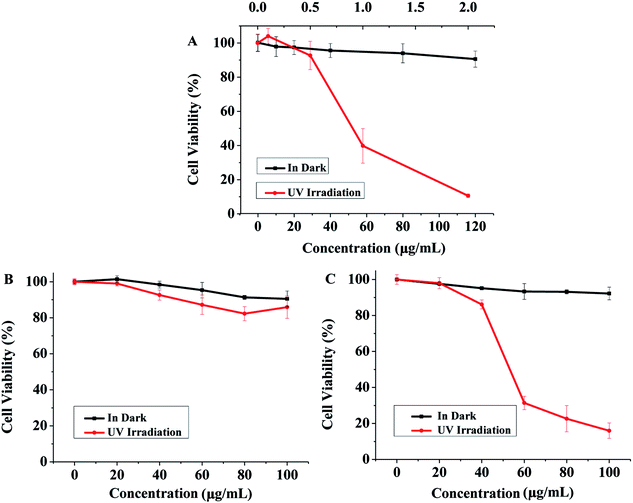 |
| Fig. 4 Phototoxicity evaluation of CAB and ACB on NIH 3T3 cells measured by neutral red uptake assay. Cell viability treated with different concentrations of anthracene (A, as the positive control), CAB (B), and ACB (C) with and without UV irradiation (n = 3). | |
CAB had no significant toxicity to the cells no matter with or without UV irradiation (Fig. 4B). However, in the case of ACB, it didn't show cytotoxicity in the dark condition but induced an obvious cell damage with UV irradiation (Fig. 4C). When the concentration of ACB increased to 100 μg mL−1, cell viability was less than 10%, indicating its possible phototoxicity. Therefore, we can conclude that CAB might be more suitable as the candidate of sunscreens.
3.7 In vivo phototoxicity test of CAB or ACB
In view of the high safety requirements for sunscreens, animal experiment was carried out in accordance with Hygienic Standard for Cosmetics (2007). The judgment criterion is the skin phototoxicity scores of skin reaction. When the skin reaction score was larger than 2 with the test substance and equal to 0 without the tested substance, the compound was considered as a phototoxic substance. Both ACB and CAB showed no skin phototoxicity (Tables 6 and 7) compared with the obvious skin damage in the positive control group (Tables 4 and 5). Different from the result of in vitro phototoxicity experiments, ACB did not show in vivo phototoxicity. Combining the both in vitro and in vivo data, CAB satisfied the requirements of sunscreen products to be safe, stable, efficient and hypoallergenic, better than ACB.
Table 4 The scores of skin reaction of the positive control group of CAB on skin phototoxicity test
Animal |
Skin reaction integral |
Number |
Gender |
Weight (g) |
1 h |
24 h |
48 h |
72 h |
1 |
2 |
3 |
4 |
1 |
2 |
3 |
4 |
1 |
2 |
3 |
4 |
1 |
2 |
3 |
4 |
1 |
Male |
268 |
0 |
0 |
0 |
0 |
0 |
4 |
0 |
0 |
0 |
4 |
0 |
0 |
0 |
3 |
0 |
0 |
2 |
Male |
249 |
0 |
1 |
0 |
0 |
0 |
3 |
0 |
0 |
0 |
4 |
0 |
0 |
0 |
3 |
0 |
0 |
3 |
Male |
253 |
0 |
1 |
0 |
0 |
0 |
3 |
0 |
0 |
0 |
4 |
0 |
0 |
0 |
3 |
0 |
0 |
4 |
Female |
257 |
0 |
0 |
0 |
0 |
0 |
4 |
0 |
0 |
0 |
4 |
0 |
0 |
0 |
3 |
0 |
0 |
5 |
Female |
265 |
0 |
0 |
0 |
0 |
0 |
4 |
0 |
0 |
0 |
3 |
0 |
0 |
0 |
3 |
0 |
0 |
6 |
Female |
242 |
0 |
0 |
0 |
0 |
0 |
4 |
0 |
0 |
0 |
4 |
0 |
0 |
0 |
4 |
0 |
0 |
Table 5 The scores of skin reaction of the positive control group (ACB) on skin phototoxicity test
Animal |
Skin reaction integral |
Number |
Gender |
Weight (g) |
1 h |
24 h |
48 h |
72 h |
1 |
2 |
3 |
4 |
1 |
2 |
3 |
4 |
1 |
2 |
3 |
4 |
1 |
2 |
3 |
4 |
1 |
Male |
268 |
0 |
0 |
0 |
0 |
0 |
4 |
0 |
0 |
0 |
4 |
0 |
0 |
0 |
3 |
0 |
0 |
2 |
Male |
249 |
0 |
1 |
0 |
0 |
0 |
3 |
0 |
0 |
0 |
4 |
0 |
0 |
0 |
3 |
0 |
0 |
3 |
Male |
253 |
0 |
1 |
0 |
0 |
0 |
3 |
0 |
0 |
0 |
4 |
0 |
0 |
0 |
3 |
0 |
0 |
4 |
Female |
257 |
0 |
0 |
0 |
0 |
0 |
4 |
0 |
0 |
0 |
4 |
0 |
0 |
0 |
3 |
0 |
0 |
5 |
Female |
265 |
0 |
0 |
0 |
0 |
0 |
4 |
0 |
0 |
0 |
3 |
0 |
0 |
0 |
3 |
0 |
0 |
6 |
Female |
242 |
0 |
0 |
0 |
0 |
0 |
4 |
0 |
0 |
0 |
4 |
0 |
0 |
0 |
4 |
0 |
0 |
Table 6 The scores of skin reaction of CAB on skin phototoxicity test
Animal |
Skin reaction integral |
Number |
Gender |
Weight (g) |
1 h |
24 h |
48 h |
72 h |
1 |
2 |
3 |
4 |
1 |
2 |
3 |
4 |
1 |
2 |
3 |
4 |
1 |
2 |
3 |
4 |
1 |
Male |
248 |
0 |
0 |
0 |
0 |
0 |
0 |
0 |
0 |
0 |
0 |
0 |
0 |
0 |
0 |
0 |
0 |
2 |
Male |
239 |
0 |
0 |
0 |
0 |
0 |
0 |
0 |
0 |
0 |
0 |
0 |
0 |
0 |
0 |
0 |
0 |
3 |
Male |
255 |
0 |
0 |
0 |
0 |
0 |
0 |
0 |
0 |
0 |
0 |
0 |
0 |
0 |
0 |
0 |
0 |
4 |
Female |
244 |
0 |
0 |
0 |
0 |
0 |
0 |
0 |
0 |
0 |
0 |
0 |
0 |
0 |
0 |
0 |
0 |
5 |
Female |
260 |
0 |
0 |
0 |
0 |
0 |
0 |
0 |
0 |
0 |
0 |
0 |
0 |
0 |
0 |
0 |
0 |
6 |
Female |
284 |
0 |
0 |
0 |
0 |
0 |
0 |
0 |
0 |
0 |
0 |
0 |
0 |
0 |
0 |
0 |
0 |
Table 7 The scores of skin reaction of ACB on skin phototoxicity test
Animal |
Skin reaction integral |
Number |
Gender |
Weight (g) |
1 h |
24 h |
48 h |
72 h |
1 |
2 |
3 |
4 |
1 |
2 |
3 |
4 |
1 |
2 |
3 |
4 |
1 |
2 |
3 |
4 |
1 |
Male |
258 |
0 |
0 |
0 |
0 |
0 |
0 |
0 |
0 |
0 |
0 |
0 |
0 |
0 |
0 |
0 |
0 |
2 |
Male |
239 |
0 |
0 |
0 |
0 |
0 |
0 |
0 |
0 |
0 |
0 |
0 |
0 |
0 |
0 |
0 |
0 |
3 |
Male |
249 |
0 |
0 |
0 |
0 |
0 |
0 |
0 |
0 |
0 |
0 |
0 |
0 |
0 |
0 |
0 |
0 |
4 |
Female |
281 |
0 |
0 |
0 |
0 |
0 |
0 |
0 |
0 |
0 |
0 |
0 |
0 |
0 |
0 |
0 |
0 |
5 |
Female |
250 |
0 |
0 |
0 |
0 |
0 |
0 |
0 |
0 |
0 |
0 |
0 |
0 |
0 |
0 |
0 |
0 |
6 |
Female |
272 |
0 |
0 |
0 |
0 |
0 |
0 |
0 |
0 |
0 |
0 |
0 |
0 |
0 |
0 |
0 |
0 |
3.8 In vivo UV protective efficacy of CAB or ACB
In order to check the practical application of the two azobenzene compounds, we estimated in vivo UV protection ability using Kunming mouse. The histological feature of photoaging is dermal elastosis, which largely consists of thickened, tangled, and ultimately granular amorphous elastic structures.37 Compared with the control group (Fig. 5-1 and 1′), the positive control (Fig. 5-2 and 2′) and the cream-treated group (Fig. 5-3 and 3′) showed the following features: the stratum corneum was thickened and shed away exposing the epidermal layer, associated with congested and increased disorganization of hair follicle. Edema and inflammation between fat cells and hair follicles can be seen in the dermis layer and sebaceous glands hyperplasia irregular establishing a photo-damage mice model.
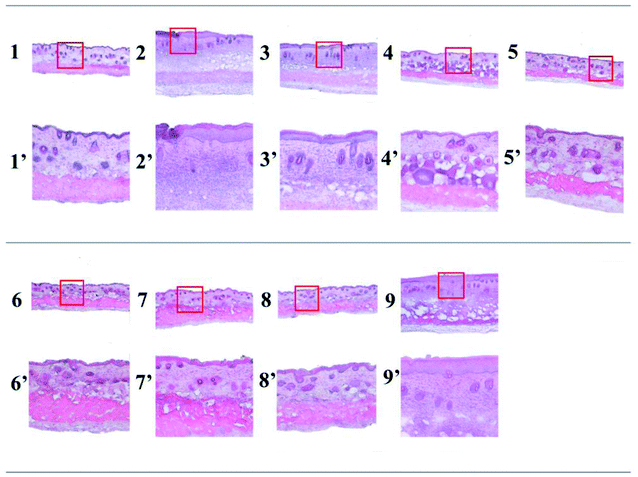 |
| Fig. 5 The skin hematoxylin-eosin (HE) staining of mouse skin. 1, Normal control group; 2, positive control group; 3, cream-treated group; 4, CAB-treated group irradiated single time; 5, ACB-treated group irradiated single time; 6, AVO-treated group irradiated single time; 7, CAB-treated group irradiated twice; 8, ACB-treated group irradiated twice; 9, AVO-treated group irradiated twice; 1′–9′ are magnification (100×) of highlighted areas in 1–9 (40×). | |
Compared with the positive control group, the mouse skin in the normal control group 1 and in the groups treated with CAB (Fig. 5-4 and 4′), ACB (Fig. 5-5 and 5′) and AVO (Fig. 5-6 and 6′) with exposure of 172.8 J cm−2 UV light showed similar features. The results proved that CAB, ACB and AVO displayed sunlight protection ability and prevented the process of mouse skin photoaging. Repeated exposure to UVA was further carried out to evaluate the reusable function of the three compounds. Obviously, the stratum corneum of the group treated with AVO (Fig. 5-9 and 9′) was fallen off; the epidermis layer and the dermis layer were significantly thickened than group 7 (Fig. 5-7 and 7′) and group 8 (Fig. 5-8 and 8′). Therefore, AVO lost its protective ability due to photodegradation, while both CAB and ACB recovered their abilities as sunscreens after visible light irradiation.
4. Conclusions
Both CAB and ACB have efficient absorption ability in UVA range, which laid the foundation for their application as sunscreens. CAB and ACB either in DMSO or cream can transfer from trans-state to cis-state under UV irradiation, and the reversible process can occur under visible light irradiation, implying that azobenzene compounds can be used repeatedly. Furthermore, neither DNA damage nor apoptosis was detected when co-incubation with cells. Although both azobenzene compounds have the ability of UV protection, ACB showed cell phototoxicity while CAB was found safe in evaluation of both in vitro and in vivo. Thus CAB is a better candidate as sunscreens.
Conflicts of interest
There are no conflicts to declare.
Acknowledgements
The authors gratefully acknowledge the financial support provided by the International S&T Cooperation Program of China (2014DFG02330, 2015DFG32730), the Shanghai Municipal Science and Technology Commission (13JC1403400, 15540723900), and Shanghai Municipal Education Commission (Gaofeng Biomedical Engineering Grant, ZXGF082101). We thank to the Instrumental Analysis Center and Center for Advanced Electronic Materials and Devices of Shanghai Jiaotong University.
References
- M. Wharton, M. Geary, N. O'Connor and B. Murphy, Int. J. Cosmet. Sci., 2011, 33, 164–170 CrossRef CAS PubMed.
- P. Kullavanijaya and H. W. Lim, J. Am. Acad. Dermatol., 2005, 52, 937–958 CrossRef PubMed.
- L. H. Kligman, F. J. Akin and A. M. Kligman, J. Invest. Dermatol., 1985, 84, 272–276 CrossRef CAS PubMed.
- N. Agar and A. R. Young, Mutat. Res., 2005, 571, 121 CrossRef CAS PubMed.
- D. H. Sliney, J. Photochem. Photobiol., B, 2001, 64, 166–175 CrossRef CAS.
- R. Wolf, B. Tüzün and Y. Tüzün, Dermatol. Ther., 2001, 14, 208–214 CrossRef.
- E. Chatelain and B. Gabard, Photochem. Photobiol., 2001, 74, 401–406 CrossRef CAS PubMed.
- S. Y. Geng, B. Yang, G. Wang, G. Qin, S. Wada and J. Y. Wang, Nanotechnology, 2014, 25, 1016–1030 CrossRef PubMed.
- Y. Q. Dai, G. Qin, S. Y. Geng, B. Yang, Q. Xu and J. Y. Wang, RSC Adv., 2012, 2, 3340–3346 RSC.
- X. M. Liu, B. Yang, Y. L. Wang and J. Y. Wang, BBA, Biochim. Biophys. Acta, Biomembr., 2005, 1720, 28–34 CrossRef CAS PubMed.
- X. M. Liu, B. Yang, Y. L. Wang and J. Y. Wang, Chem. Mater., 2005, 17, 2792–2795 CrossRef CAS.
- S. Y. Geng, L. P. Wang, T. Kouyama, T. Gotoh, S. Wada and J. Y. Wang, Sci. Rep., 2016, 7, 39202 CrossRef PubMed.
- A. A. Beharry, L. Wong, V. Tropepe and G. A. Woolley, Angew. Chem., Int. Ed., 2011, 50, 1325–1327 CrossRef CAS PubMed.
- A. A. Beharry and G. A. Woolley, Chem. Soc. Rev., 2011, 40, 4422–4437 RSC.
- S. Samanta, A. A. Beharry, O. Sadovski, T. M. Mccormick, A. Babalhavaeji, V. Tropepe and G. A. Woolley, J. Am. Chem. Soc., 2013, 135, 9777–9784 CrossRef CAS PubMed.
- J. Chauveau, M. Meunier and A. Benoit, Int. J. Cancer, 1974, 13, 1–8 CrossRef CAS PubMed.
- K. T. Chung, J. Environ. Sci. Health, Part C: Environ. Carcinog. Ecotoxicol. Rev., 2000, 18, 51–74 CrossRef.
- S. Goetze, C. Hiernickel and P. Elsner, Skin Pharmacol. Physiol., 2017, 30, 76–80 CrossRef CAS PubMed.
- M. Horie, S. Sugino, H. Kato, Y. Tabei, A. Nakamura and Y. Yoshida, Toxicol. Mech. Methods, 2016, 26, 284–294 CrossRef CAS PubMed.
- C. M. Kawakami, L. N. C. Máximo, B. B. Fontanezi, R. S. da Silva and L. R. Gaspar, Eur. J. Pharm. Sci., 2017, 99, 299–309 CrossRef CAS PubMed.
- A. C. Green and G. M. Williams, Cancer Epidemiol., Biomarkers Prev., 2007, 16, 1921–1922 CAS.
- B. Yang, S. Y. Geng, X. M. Liu, J. T. Wang, Y. K. Chen, Y. L. Wang and J. Y. Wang, Soft Matter, 2011, 8, 518–525 RSC.
- Q. Xu, S. Geng, Y. Dai, G. Qin and J. Y. Wang, J. Photochem. Photobiol., B, 2013, 129, 78–86 CrossRef CAS PubMed.
- B. B. Zhang, J. L. He, Y. Bei, Y. Y. Zhang, Y. D. Huang and Q. Xiang, J. Pharm. Biomed. Sci., 2016, 6(5), 295–300 CrossRef CAS PubMed.
- D. Moyal, Expert Rev. Dermatol., 2008, 3, 307–313 CrossRef CAS.
- A. S. Barnard, Nat. Nanotechnol., 2010, 5, 271–274 CrossRef PubMed.
- L. E. Agrapidis-Paloympis and R. A. Nash, J. Soc. Cosmet. Chem., 1987, 38, 209–221 CAS.
- H. Zhao, S. Sen, T. Udayabhaskararao, M. Sawczyk, K. Kučanda, D. Manna, P. K. Kundu, J. W. Lee, P. Král and R. Klajn, Nat. Nanotechnol., 2016, 11, 82–88 CrossRef CAS PubMed.
- C. Paris, V. Lhiaubet-Vallet, O. Jiménez, C. Trullas and M. Á. Miranda, Photochem. Photobiol., 2009, 85, 178–184 CrossRef CAS PubMed.
- W. G. Wamer, P. Vath and D. E. Falvey, Free Radical Biol. Med., 2003, 34, 233–242 CrossRef CAS PubMed.
- M. Singh, P. Kaur, R. Sandhir and R. Kiran, Mutat. Res., 2008, 654, 145–149 CAS.
- K. Wassermann, K. W. Kohn and V. A. Bohr, J. Biol. Chem., 1990, 265, 13906–13913 CAS.
- S. E. Freeman, A. D. Blackett, D. C. Monteleone, R. B. Setlow, B. M. Sutherland and J. C. Sutherland, Anal. Biochem., 1986, 158, 119–129 CrossRef CAS PubMed.
- C. Charriaut-Marlangue and Y. Ben-Ari, NeuroReport, 1995, 7, 61–64 CrossRef CAS PubMed.
- R. P. Rastogi, S. P. Singh, D.-P. Häder and R. P. Sinha, Biochem. Biophys. Res. Commun., 2010, 397, 603–607 CrossRef CAS PubMed.
- H. Spielmann, M. Balls, J. Dupuis, W. J. Pape, O. De Silva, H.-G. Holzhütter, F. Gerberick, M. Liebsch, W. W. Lovell and U. Pfannenbecker, Altern. Lab. Anim., 1998, 26, 679–708 CAS.
- Y. Wu, Q. Tian, L. Li, M. N. Khan, X. Yang, Z. Zhang, X. Hu and S. Chen, Funct. Foods, 2013, 5, 527–538 CrossRef CAS.
Footnote |
† Electronic supplementary information (ESI) available. See DOI: 10.1039/c7ra10290a |
|
This journal is © The Royal Society of Chemistry 2018 |
Click here to see how this site uses Cookies. View our privacy policy here.