DOI:
10.1039/C8QO00025E
(Research Article)
Org. Chem. Front., 2018,
5, 1143-1147
Copper(II)-catalyzed trifluoromethylation of iodoarenes using Chen's reagent†
Received
10th January 2018
, Accepted 24th January 2018
First published on 25th January 2018
Abstract
The introduction of a trifluoromethyl group to organic molecules is significant for modern drug discovery; thus practical routes towards catalytic trifluoromethylation are highly desired. Herein, we report the efficient copper(II)-catalyzed nucleophilic trifluoromethylation of various aryl and heteroaryl iodides using methyl fluorosulfonyldifluoroacetate (FO2SCF2CO2Me, Chen's reagent). The use of CuCl2 instead of CuI resulted in a significant improvement in the original Chen's methodology; specifically, catalytic amounts (10 to 15%) of CuCl2 were used instead of Cu(I) salts for the generation of CuCF3 species. The improved trifluoromethylation converts aryl and heteroaryl iodides into the corresponding CF3-containing molecules with multiple functional groups in moderate to high yields. Moreover, a mechanism was proposed for this new catalytic system.
Introduction
Fluorine plays a unique role in chemical transformations, pharmaceutical effects, and physical properties; as a result, a variety of trifluoromethylated aromatics have been developed for potent drugs such as Prozac.1–3 Many strategies have been applied for the trifluoromethylation of aryl and heteroaryl compounds, including radical trifluoromethylation, electrophilic trifluoromethylation, and nucleophilic trifluoromethylation.4–15 Among them, CF3Cu complexes are generally prepared or generated in situ to ensure efficient trifluormethylation.16–20 However, copper-mediated trifluoromethylation reactions of haloarenes usually require stoichiometric or excess amounts of copper species to obtain good yields of trifluoromethylated products. As such, catalytic trifluoromethylation reactions are in high demand and represent a significant challenge in organic chemistry.
In 1989, Chen first reported the catalytic trifluoromethylation of aryl, alkenyl, and benzyl halides using methyl fluorosulfonyldifluoroacetate (FO2SCF2CO2Me, Chen's reagent).20 Today, this approach is well-recognized as Chen's methodology.16,18a,21–24 Besides this example, catalytic trifluoromethylation was not reported until 2009 (Scheme 1).8,25–31 In 2009, Amii reported a catalytic trifluoromethylation reaction using Cu(I)-diamine complexes and CF3SiEt3.25a In 2010, Buchwald achieved the Pd-catalyzed trifluoromethylation of aryl chlorides using a phosphine ligand such as BrettPhos or RuPhos.30 Mechanistically, “ligandless” reactions facilitate the reactivity of “CuCF3” and thus promote the oxidative addition of “CuCF3” to aryl halides.32 However, catalysis without nitrogen or phosphine ligands has not been extensively studied.
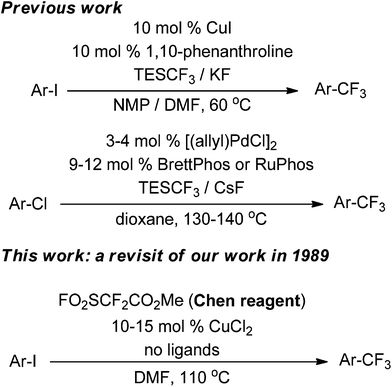 |
| Scheme 1 Nucleophilic trifluoromethylations. | |
Chen's reagent is a commercially available and easy to handle liquid for the trifluoromethylation of haloarenes; it exhibits good functional group compatibility and a versatile trifluoromethylation ability. This cost-effective reagent is amenable for the trifluoromethylation of a wide variety of bioactive compounds. However, even in the pioneering work, copper species were often used in stoichiometric or excess amounts in academic research and industrial applications.21,22 Chen's reagent is also used as a difluorocarbene reagent or a precursor for a difluoromethyl radical (˙CF2CO2Me).23 Moreover, a series of related reagents have been developed for trifluoromethylation.18a,24 In some cases, Chen's reagent was claimed to be better than the Ruppert–Prakash reagent (TMSCF3), CF3B(OMe)3K, and CF3CO2Na.22a Trifluoromethylation using Chen's reagent can also be induced by zero valence copper, as disclosed in Chen's early research.21a Owing to recent green requirements in transition metal catalysis, we revisited the initial publication in 1989, and found an improved catalytic trifluoromethylation, which we believe will benefit the future discovery of new medicines and agrochemicals.
Results and discussion
Among most examples described in the previous work published in 1989, excess aryl iodides were typically required, which hindered the separation of the starting materials from final products when the modifying molecules were structurally complex.20 Therefore, it is desired to use organic halides as limiting reagents.
To begin, we screened the reaction conditions using 1-iodonaphthalene (1a) as a model substrate and limiting reagent, and reacted it with Chen's reagent (2) in the presence of 10 mol% CuCl2 in DMF at 110 °C (Table 1). The 88% yield of the trifluoromethylated aromatic (3a) was inspiring (Table 1, entry 1). Next, we compared the effects of several copper(II) salts, namely CuBr2, Cu(OAc)2, and Cu(OH)2, which give the desired products in 84%, 86%, and 86% yields, respectively (Table 1, entries 2–4). However, CuO and CuF2 did not give the desired product (Table 1, entries 5 and 6). When the amount of CuCl2 was reduced to 5 mol%, the product yield decreased to 67% (Table 1, entry 7). The reduction of 2 to 2.0 equivalents or 1.5 equivalents gave the product in 80% and 73% yields (Table 1, entries 8 and 9). Finally, reaction temperatures of 100, 90, and 80 °C resulted in moderate to good yields (55 to 83%) of the products (Table 1, entries 10–12). The yield was 83% at 120 °C (Table 1, entry 13).
Table 1 Optimization of trifluoromethylation catalyzed by Cu(II) saltsa

|
Entry |
[Cu] |
[Cu] mol% |
2 (equiv.) |
Temp. (°C) |
Yieldb (%) |
Reaction conditions: 1-Iodonaphthalene (1a, 0.5 mmol), copper salt (as indicated), 2 (as indicated), at the indicated reaction temperatures in DMF (1.5 mL) under a nitrogen atmosphere for 2 h.
The yields were determined by the 19F NMR analysis of crude reaction mixtures using benzotrifluoride as the internal standard.
1a was completely consumed.
Isolated yields.
|
1 |
CuCl2 |
10 |
2.5 |
110 |
88c(82)d |
2 |
CuBr2 |
10 |
2.5 |
110 |
84c |
3 |
Cu(OAc)2 |
10 |
2.5 |
110 |
86c |
4 |
Cu(OH)2 |
10 |
2.5 |
110 |
86c |
5 |
CuO |
10 |
2.5 |
110 |
0 |
6 |
CuF2 |
10 |
2.5 |
110 |
0 |
7 |
CuCl2 |
5 |
2.5 |
110 |
67 |
8 |
CuCl2 |
10 |
2.0 |
110 |
80 |
9 |
CuCl2 |
10 |
1.5 |
110 |
73 |
10 |
CuCl2 |
10 |
2.5 |
100 |
83 |
11 |
CuCl2 |
10 |
2.5 |
90 |
72 |
12 |
CuCl2 |
10 |
2.5 |
80 |
55 |
13 |
CuCl2 |
10 |
2.5 |
120 |
83 |
Next, we explored the scope of the copper(II)-catalyzed nucleophilic trifluoromethylation of aromatic and heteroaromatic iodides (Table 2). Electron-deficient aryl iodides exhibited good reactivity and the products (3e–3m, 3p, and 3q) were obtained in 78–92% yields. While some electron-neutral (3a–3c) and electron-rich substrates (3s) gave the corresponding products in 78–85% yields, aryl and heteroaryl substrates required slightly greater amounts of the catalyst (15 mol% of CuCl2) and 3 equivalents of 2 for a good conversion. Thus, 3d, 3n, 3o, 3r, 3t, and 3v were obtained in 51 to 82% yields. An ortho effect was observed upon comparing o-methoxy and p-methoxy aryl iodides (3rvs. 3s). A range of functional groups, including nitro, halogen, aldehyde, ether, ester, ketone, cyano, and hydroxyl moieties, were tolerated in this transformation. Notably, halogen, aldehyde, ketone, ester, and hydroxyl groups can provide a complementary platform for further transformations. Additionally, heteroaryl iodides (5-chloro-2-iodopyrimidine, 3-iodo-2-methoxypyridine, 2-iodo-4-methoxypyrimidine, 3-iodothiophene, and 2-iodopyridine) were all suitable substrates, giving the desired trifluoromethylated products (3w–3aa) in moderate to good yields (55–83%).
Table 2 Substrate scope of copper(II)-catalyzed trifluoromethylationa,b
Reaction conditions: Iodides (0.5 mmol), 2 (2.5 equiv.) and CuCl2 (10 mol%) at 110 °C in DMF (1.5 mL) under a nitrogen atmosphere for 2 h.
Isolated yields (19F NMR yields).
Reaction conditions: Iodides (0.5 mmol), 2 (3 equiv.), and CuCl2 (15 mol%), at 110 °C in DMF (1.5 mL) under a nitrogen atmosphere for 2 h.
|
|
In order to demonstrate the potential synthetic utility of this reaction, a large-scale experiment was performed, which produced 3e in 83% yield (Scheme 2a). This methodology could be used to produce biologically active molecules such as Prozac (Scheme 2b).
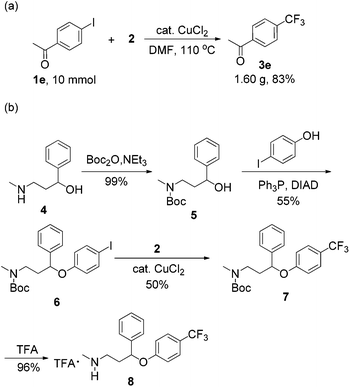 |
| Scheme 2 Application of Cu(II)-catalyzed trifluoromethylation. | |
To gain further insights into the Cu(II)-catalyzed trifluoromethylation reaction, the decomposition of 2 and its reaction with CuCl2 were studied using 19F NMR spectroscopy (see the ESI†). When CuCl2 was mixed with 2 in a 0.2
:
1 ratio in DMF, two signals at −28.9 ppm and −34.1 ppm were detected within 5 min. According to the literature,33,34 the signals at −28.9 ppm and −34.1 ppm were assigned “CuCF3” and “[Cu(CF3)4]−,” respectively. Based on these findings, we proposed a mechanism for the Cu(II) catalyzed trifluoromethylation (Scheme 3). First, CuCl2 reacts with 2 in DMF giving Cu(I) (“CuCF3”) and Cu(III) ([Cu(CF3)4]−) species. The “ligandless” CuCF3 generated in situ reacts with haloarenes to provide the desired trifluoromethylated products and generates CuI, which acts as the catalyst for the reaction. By using a catalytic amount of CuI, the yield of trifluoromethylation was 86% (for 3a). When KCl and Bu4NCl were added as an additive to the reaction mixture under the CuI catalyzed conditions, the yields decreased to 48% and 72%, respectively. These experiments imply that the CuI instead of chlorides is the true catalyst for the reaction.
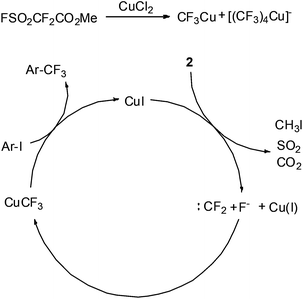 |
| Scheme 3 Proposed mechanism of Cu(II)-catalyzed trifluoromethylation with Chen's reagent. | |
Conclusion
The efficient copper(II)-catalyzed nucleophilic trifluoromethylation of aryl and heteroaryl iodides was achieved in the presence of Chen's reagent. 10–15 mol% Cu(II) chloride allowed the conversion of various aryl and heteroaryl iodides into the corresponding trifluoromethyl-containing products in moderate to high yields. The reaction was applicable to both electron-deficient and electron-rich arenes, as well as heteroarenes, and a broad range of functional groups was tolerated. The power of Chen's methodology was highlighted, and this work will reinforce the synthesis of functional molecules bearing CF3 moieties.
Conflicts of interest
There are no conflicts to declare.
Acknowledgements
The support for our work by the National Natural Science Foundation of China (No. 21737004, 21672239, 21421002, and 21302207), and Key Laboratory of Photochemical Conversion and Optoelectronic Materials, TIPC, CAS is gratefully acknowledged.
References
- Y. Zhou, J. Wang, Z. Gu, S. Wang, W. Zhu, J. L. Aceña, V. A. Soloshonok, K. Izawa and H. Liu, Chem. Rev., 2016, 116, 422–518 CrossRef CAS PubMed.
- D. O'Hagan, Chem. Soc. Rev., 2008, 37, 308–319 RSC.
-
(a) C. Alonso, E. M. de Marigorta, G. Rubiales and F. Palacios, Chem. Rev., 2015, 115, 1847–1935 CrossRef CAS PubMed;
(b) M. A. McClinton and D. A. McClinton, Tetrahedron, 1992, 48, 6555–6666 CrossRef CAS.
-
(a) C.-P. Zhang, Q.-Y. Chen, Y. Guo, J.-C. Xiao and Y.-C. Gu, Coord. Chem. Rev., 2014, 261, 28–72 CrossRef CAS;
(b) S. Roy, B. T. Gregg, G. W. Gribble, V.-D. Le and S. Roy, Tetrahedron, 2011, 67, 2161–2195 CrossRef CAS;
(c) S. L. Clarke and G. P. McGlacken, Chem. – Eur. J., 2017, 23, 1219–1230 CrossRef CAS PubMed.
- X. Liu, C. Xu, M. Wang and Q. Liu, Chem. Rev., 2015, 115, 683–730 CrossRef CAS PubMed.
- G. K. Prakash and A. K. Yudin, Chem. Rev., 1997, 97, 757–786 CrossRef CAS PubMed.
-
(a) J. Charpentier, N. Fruh and A. Togni, Chem. Rev., 2015, 115, 650–682 CrossRef CAS PubMed;
(b) T. Umemoto, Chem. Rev., 1996, 96, 1757–1778 CrossRef CAS PubMed.
-
(a) J. Xu, D.-F. Luo, B. Xiao, Z.-J. Liu, T.-J. Gong, Y. Fu and L. Liu, Chem. Commun., 2011, 47, 4300–4302 RSC;
(b) T. Liu and Q. Shen, Org. Lett., 2011, 13, 2342–2345 CrossRef CAS PubMed.
- H. Egami and M. Sodeoka, Angew. Chem., Int. Ed., 2014, 53, 8294–8308 CrossRef CAS PubMed.
- E. Merino and C. Nevado, Chem. Soc. Rev., 2014, 43, 6598–6608 RSC.
- A. Studer, Angew. Chem., Int. Ed., 2012, 51, 8950–8958 CrossRef CAS PubMed.
- D. A. Nagib and D. W. MacMillan, Nature, 2011, 480, 224–228 CrossRef CAS PubMed.
- Y. Ye and M. S. Sanford, J. Am. Chem. Soc., 2012, 134, 9034–9037 CrossRef CAS PubMed.
- O. A. Tomashenko and V. V. Grushin, Chem. Rev., 2011, 111, 4475–4521 CrossRef CAS PubMed.
- M. D. Levin, T. Q. Chen, M. E. Neubig, C. M. Hong, C. A. Theulier, I. J. Kobylianskii, M. Janabi, J. P. O'Neil and F. D. Toste, Science, 2017, 356, 1272–1276 CrossRef CAS PubMed.
- Q.-Y. Chen, J. Fluorine Chem., 1995, 72, 241–246 CrossRef CAS.
-
(a) Y. Kobayashi and I. Kumadaki, J. Chem. Soc., Perkin Trans. 1, 1980, 661–664 RSC;
(b) D. J. Burton and D. M. Wiemers, J. Am. Chem. Soc., 1985, 107, 5014–5015 CrossRef CAS;
(c) G. E. Carr, R. D. Chambers, T. F. Holmes and D. G. Parker, J. Chem. Soc., Perkin Trans. 1, 1988, 921–926 RSC;
(d) J. G. Macneil and D. J. Burton, J. Fluorine Chem., 1991, 55, 225–227 CrossRef CAS;
(e) H. Urata and T. Fuchikami, Tetrahedron Lett., 1991, 32, 91–94 CrossRef CAS.
-
(a) G. Zhao, H. Wu, Z. Xiao, Q.-Y. Chen and C. Liu, RSC Adv., 2016, 6, 50250–50254 RSC;
(b) C.-P. Zhang, J. Cai, C.-B. Zhou, X.-P. Wang, X. Zheng, Y.-C. Gu and J.-C. Xiao, Chem. Commun., 2011, 47, 9516–9518 RSC;
(c) A. Zanardi, M. A. Novikov, E. Martin, J. Benet-Buchholz and V. V. Grushin, J. Am. Chem. Soc., 2011, 133, 20901–20913 CrossRef CAS PubMed;
(d) P. Ivashkin, G. Lemonnier, J. Cousin, V. Gregoire, D. Labar, P. Jubault and X. Pannecoucke, Chem. – Eur. J., 2014, 20, 9514–9518 CrossRef CAS PubMed;
(e) X. Zhang, J. Wang and Z. Wan, Org. Lett., 2015, 17, 2086–2089 CrossRef CAS PubMed;
(f) X. Li, J. Zhao, L. Zhang, M. Hu, L. Wang and J. Hu, Org. Lett., 2015, 17, 298–301 CrossRef CAS PubMed.
-
(a) G. G. Dubinina, H. Furutachi and D. A. Vicic, J. Am. Chem. Soc., 2008, 130, 8600–8601 CrossRef CAS PubMed;
(b) H. Morimoto, T. Tsubogo, N. D. Litvinas and J. F. Hartwig, Angew. Chem., Int. Ed., 2011, 50, 3793–3798 CrossRef CAS PubMed;
(c) X. Lin, C. Hou, H. Li and Z. Weng, Chem. – Eur. J., 2016, 22, 2075–2084 CrossRef CAS PubMed;
(d) O. A. Tomashenko, E. C. Escudero-Adan, M. M. Belmonte and V. V. Grushin, Angew. Chem., Int. Ed., 2011, 50, 7655–7659 CrossRef CAS PubMed;
(e) X. Lin, Z. Li, X. Han and Z. Weng, RSC Adv., 2016, 6, 75465–75469 RSC.
- Q.-Y. Chen and S.-W. Wu, J. Chem. Soc., Chem. Commun., 1989, 705–706 RSC.
-
(a) Q.-Y. Chen, G.-Y. Yang and S.-W. Wu, J. Fluorine Chem., 1991, 55, 291–298 CrossRef CAS;
(b) X.-S. Fei, W.-S. Tian and Q.-Y. Chen, Bioorg. Med. Chem. Lett., 1997, 7, 3113–3118 CrossRef CAS;
(c) X.-S. Fei, W.-S. Tian and Q.-Y. Chen, J. Chem. Soc., Perkin Trans. 1, 1998, 1139–1142 RSC;
(d) Submitted by X.-S. Fei, W.-S. Tian, K. Ding, Y. Wang and Q.-Y. Chen, Checked by T. Yamakawa and T. Fukuyama, Org. Synth., 2010, 87, 126–136 Search PubMed;
(e) J.-X. Duan, D.-B. Su, J.-P. Wu and Q.-Y. Chen, J. Fluorine Chem., 1994, 66, 167–169 CrossRef CAS;
(f) S. Zhao, C. Liu, Y. Guo, J.-C. Xiao and Q.-Y. Chen, Synthesis, 2014, 1674–1688 Search PubMed;
(g) J. Chen, K.-L. Li, Y. Guo, C. Liu, C.-C. Guo and Q.-Y. Chen, RSC Adv., 2013, 3, 8227–8231 RSC;
(h) L.-M. Jin, L. Chen, J.-J. Yin, J.-M. Zhou, C.-C. Guo and Q.-Y. Chen, J. Org. Chem., 2006, 71, 527–536 CrossRef CAS PubMed;
(i) L.-M. Jin, L. Chen, J.-J. Yin, C.-C. Guo and Q.-Y. Chen, Eur. J. Org. Chem., 2005, 3994–4001 CrossRef CAS;
(j) C. Liu and Q.-Y. Chen, Eur. J. Org. Chem., 2005, 3680–3686 CrossRef CAS.
-
(a) R. S. Foster, H. Jakobi and J. P. Harrity, Org. Lett., 2012, 14, 4858–4861 CrossRef CAS PubMed;
(b) Y. Deng, C. Sun, D. K. Hunt, C. Fyfe, C. L. Chen, T. H. Grossman, J. A. Sutcliffe and X. Y. Xiao, J. Med. Chem., 2017, 60, 2498–2512 CrossRef CAS PubMed;
(c) N. Sharma, N. Kumari, T. S. Chundawat, S. Kumar and S. Bhagat, RSC Adv., 2017, 7, 10150–10153 RSC;
(d) Z. Xu, S. Pan and Y. Huang, Chin. J. Org. Chem., 2014, 34, 1391–1398 CrossRef CAS;
(e) M. L. Maddess, J. P. Scott, A. Alorati, C. Baxter, N. Bremeyer, S. Brewer, K. Campos, E. Cleator, A. Dieguez-Vazquez, A. Gibb, A. Gibson, M. Howard, S. Keen, A. Klapars, J. Lee, J. Li, J. Lynch, P. Mullens, D. Wallace and R. Wilson, Org. Process Res. Dev., 2014, 18, 528–538 CrossRef CAS;
(f) H. Nishiyama, M. Ono, T. Sugimoto, T. Sasai, N. Asakawa, S. Ueno, Y. Tominaga, T. Yaegashi, M. Nagaoka, T. Matsuzaki, N. Kogure, M. Kitajima and H. Takayama, MedChemComm, 2014, 5, 452 RSC;
(g) J. F. Miller, P. Y. Chong, J. B. Shotwell, J. G. Catalano, V. W. Tai, J. Fang, A. L. Banka, C. D. Roberts, M. Youngman, H. Zhang, Z. Xiong, A. Mathis, J. J. Pouliot, R. K. Hamatake, D. J. Price, J. W. Seal 3rd, L. L. Stroup, K. L. Creech, L. H. Carballo, D. Todd, A. Spaltenstein, S. Furst, Z. Hong and A. J. Peat, J. Med. Chem., 2014, 57, 2107–2120 CrossRef CAS PubMed;
(h) J. A. Mulder, R. P. Frutos, N. D. Patel, B. Qu, X. Sun, T. G. Tampone, J. Gao, M. Sarvestani, M. C. Eriksson, N. Haddad, S. Shen, J. J. Song and C. H. Senanayake, Org. Process Res. Dev., 2013, 17, 940–945 CrossRef CAS;
(i) Y. Wang, M. Han, L. Zhang, S. Zhou and Z. Duan, Chin. J. Org. Chem., 2013, 33, 1057–1061 CrossRef CAS;
(j) A. Aguilar, H. Zhou, J. Chen, L. Liu, L. Bai, D. McEachern, C. Y. Yang, J. Meagher, J. Stuckey and S. Wang, J. Med. Chem., 2013, 56, 3048–3067 CrossRef CAS PubMed;
(k) T. Sifferlen, R. Koberstein, E. Cottreel, A. Boller, T. Weller, J. Gatfield, C. Brisbare-Roch, F. Jenck and C. Boss, Bioorg. Med. Chem. Lett., 2013, 23, 2212–2216 CrossRef CAS PubMed;
(l) E. M. Skoda, G. C. Davis and P. Wipf, Org. Process Res. Dev., 2012, 16, 26–34 CrossRef CAS PubMed;
(m) F.-L. Qing and F. Zheng, Synlett, 2011, 1052–1072 CrossRef CAS;
(n) P. Wipf, J. Xiao and C. R. Stephenson, Chimia, 2009, 63, 764–775 CrossRef CAS PubMed;
(o) C.-L. Wang, H.-Q. Li, W.-D. Meng and F.-L. Qing, Bioorg. Med. Chem. Lett., 2005, 15, 4456–4458 CrossRef CAS PubMed;
(p) X. Zheng, W.-D. Meng, Y.-Y. Xu, J.-G. Cao and F.-L. Qing, Bioorg. Med. Chem. Lett., 2003, 13, 881–884 CrossRef CAS PubMed;
(q) F.-L. Qing, J. F. Fan, H.-B. Sun and X.-J. Yue, J. Chem. Soc., Perkin Trans. 1, 1997, 3053–3057 RSC;
(r) F.-L. Qing and J. Fan, Bioorg. Med. Chem. Lett., 1997, 7, 2117–2120 CrossRef CAS;
(s) F.-L. Qing, X. Zhang and Y. Peng, J. Fluorine Chem., 2001, 111, 185–187 CrossRef CAS.
-
(a) S. Eusterwiemann, H. Martinez and W. R. Dolbier Jr., J. Org. Chem., 2012, 77, 5461–5464 CrossRef CAS PubMed;
(b) W. Yu, X.-H. Xu and F.-L. Qing, Org. Lett., 2016, 18, 5130–5133 CrossRef CAS PubMed;
(c) C. S. Thomoson, H. Martinez and W. R. Dolbier, J. Fluorine Chem., 2013, 150, 53–59 CrossRef CAS.
-
(a) Y. Liu, H. Wu, Y. Guo, J.-C. Xiao, Q.-Y. Chen and C. Liu, Angew. Chem., Int. Ed., 2017, 56, 15432–15435 CrossRef CAS PubMed;
(b) Z.-Y. Long, J.-X. Duan, Y.-B. Lin, C.-Y. Guo and Q.-Y. Chen, J. Fluorine Chem., 1996, 78, 177–181 CrossRef CAS;
(c) D.-B. Su, J.-X. Duan and Q.-Y. Chen, Tetrahedron Lett., 1991, 32, 7689–7690 CrossRef CAS;
(d) J.-X. Duan and Q.-Y. Chen, J. Chem. Soc., Perkin Trans. 1, 1994, 725–730 RSC;
(e) Q.-Y. Chen and J.-X. Duan, J. Chem. Soc., Chem. Commun., 1993, 1389–1391 RSC;
(f) J.-X. Duan, D.-B. Su and Q.-Y. Chen, J. Fluorine Chem., 1993, 61, 279–284 CrossRef CAS;
(g) Q.-Y. Chen and S.-W. Wu, J. Chem. Soc., Perkin Trans. 1, 1989, 2385–2387 RSC.
-
(a) M. Oishi, H. Kondo and H. Amii, Chem. Commun., 2009, 1909–1911 RSC;
(b) H. Kondo, M. Oishi, K. Fujikawa and H. Amii, Adv. Synth. Catal., 2011, 353, 1247–1252 CrossRef CAS;
(c) N. Shimizu, H. Kondo, M. Oishi, K. Fujikawa, K. Komoda and H. Amii, Org. Synth., 2016, 93, 147–162 CrossRef CAS.
- T. Knauber, F. Arikan, G. V. Röschenthaler and L. J. Gooßen, Chem. – Eur. J., 2011, 17, 2689–2697 CrossRef CAS PubMed.
- Z. Weng, R. Lee, W. Jia, Y. Yuan, W. Wang, X. Feng and K.-W. Huang, Organometallics, 2011, 30, 3229–3232 CrossRef CAS.
- K. Aikawa, Y. Nakamura, Y. Yokota, W. Toya and K. Mikami, Chem. – Eur. J., 2015, 21, 96–100 CrossRef CAS PubMed.
- J. Zheng, J.-H. Lin, X.-Y. Deng and J.-C. Xiao, Org. Lett., 2015, 17, 532–535 CrossRef CAS PubMed.
- E. J. Cho, T. D. Senecal, T. Kinzel, Y. Zhang, D. A. Watson and S. L. Buchwald, Science, 2010, 328, 1679–1681 CrossRef CAS PubMed.
- D. M. Ferguson, J. R. Bour, A. J. Canty, J. W. Kampf and M. S. Sanford, J. Am. Chem. Soc., 2017, 139, 11662–11665 CrossRef CAS PubMed.
- A. I. Konovalov, A. Lishchynskyi and V. V. Grushin, J. Am. Chem. Soc., 2014, 136, 13410–13425 CrossRef CAS PubMed.
- D. M. Wiemers and D. J. Burton, J. Am. Chem. Soc., 1986, 108, 832–834 CrossRef CAS.
-
(a) S.-L. Zhang and W.-F. Bie, Dalton Trans., 2016, 45, 17588–17592 RSC;
(b) N. Nebra and V. V. Grushin, J. Am. Chem. Soc., 2014, 136, 16998–17001 CrossRef CAS PubMed.
Footnote |
† Electronic supplementary information (ESI) available. See DOI: 10.1039/c8qo00025e |
|
This journal is © the Partner Organisations 2018 |
Click here to see how this site uses Cookies. View our privacy policy here.