DOI:
10.1039/C7MD00527J
(Research Article)
Med. Chem. Commun., 2018,
9, 160-164
Design, synthesis and antibacterial evaluation of a polycationic calix[4]arene derivative alone and in combination with antibiotics†
Received
17th October 2017
, Accepted 1st December 2017
First published on 1st December 2017
Abstract
The growing antibiotic resistance phenomenon continues to stimulate the search for new compounds and strategies to combat bacterial infections. In this study, we designed and synthesized a new polycationic macrocyclic compound (2) bearing four N-methyldiethanol ammonium groups clustered and circularly organized by a calix[4]arene scaffold. The in vitro activity of compound 2, alone and in combination with known antibiotics (ofloxacin, chloramphenicol or tetracycline), was assessed against strains of Staphylococcus aureus (ATCC 6538 and methicillin-resistant isolate 15), S. epidermidis (ATCC 35984 and methicillin-resistant isolate 57), and Pseudomonas aeruginosa (ATCC 9027 and antibiotic-resistant isolate 1). Calix[4]arene derivative 2 showed significant antibacterial activity against ATCC and methicillin-resistant Gram positive Staphylococci, improved the stability of tetracycline in water, and in combination with antibiotics enhanced the antibiotic efficacy against Gram negative P. aeruginosa by an additive effect.
Introduction
The discovery of antibiotics has revolutionized the treatment and prevention of infectious diseases considered incurable in the past. But, unfortunately, overuse or misuse of antibiotics and gene recombination mechanisms have led to the onset of a selection of resistant bacteria, with the result being that common infections could once again become fatal. For this reason, the fight against community-acquired or nosocomial bacterial infections is a subject of topicality in the field of public health and stimulates an urgent need for new antibiotics and strategies.1
Calix[n]arenes are a family of oligomeric phenolic macrocycles of great interest in host–guest chemistry.2 The low cytotoxicity and limited immunogenicity of water-soluble calix[n]arene derivatives3 are features that have opened the way to the investigation of these macrocycles in the pharmaceutical and biomedical fields.4 The functionalization of the calixarene backbone has provided a variety of pharmacologically active derivatives, including compounds with antimicrobial activity.5 The covalent conjugation of nalidixic acid to the calix[4]arene skeleton provided a prodrug with antibacterial activity,6 and the clustering of multiple guanidinium units onto a calix[4]arene platform provided polycationic oligomers with higher antibacterial activity and lower cytotoxicity than the monomeric analogues versus eukaryotic cells.7,8 We ourselves observed that the arrangement of multiple units of fucose onto a calix[4]arene platform gave a Pseudomonas aeruginosa biofilm inhibitor9 and demonstrated that the entrapment of a nitric oxide radical photodonor10 or photosensitizers11 into a polycationic micellar calix[4]arene resulted in light-controlled bactericidal formulations. In the search for new antibacterial compounds, the ability of the hydrophobic cavity of calix[n]arene derivatives to form inclusion complexes with metals such as Ag ions12 or antibiotics such as isoniazid13 and fluoroquinolones14 also appeared very promising. Indeed, the inclusion complexes resulted in an enhancement of the antibiotic physicochemical and biopharmaceutical properties.
Since drug pairs can interact synergistically or additively to yield effects larger than those expected from the individual drugs, the combination of antibiotics is considered an option to combat antibiotic-resistant bacteria. With this in mind, here we report the synthesis of a new polycationic calix[4]arene derivative (2) and the evaluation of its antibacterial activity alone and in combination with ofloxacin, chloramphenicol or tetracycline. Additivity, synergy and antagonism were evaluated in vitro by the fractional inhibitory concentration index (FIC) against bacterial reference strains (Staphylococcus aureus ATCC 6538, S. epidermidis ATCC 35984, and Pseudomonas aeruginosa ATCC 9027) representative of important pathogens currently encountered in medical practice, and against methicillin-resistant S. aureus (MRSA) 15 and methicillin-resistant S. epidermidis (MRSE) 57, and antibiotic-resistant P. aeruginosa isolate 1, derived from patients undergoing cataract surgery.15
Results and discussion
Design, synthesis and structural characterization of calix[4]arene derivative 2
In the design of a new water-soluble calix[4]arene derivative endowed with antibacterial properties, we selected N-methyldiethanol ammonium moieties as cationic polar head groups because of the known ability of N-methyl-hydroxylamines to inhibit the growth of Gram-positive and Gram-negative bacteria without harmful effects on eukaryotic cells.16 Recently, we demonstrated that a calix[4]arene derivative bearing N-dimethylethanol ammonium moieties as a polycationic nanocarrier of photo-activated drugs exhibited low cytotoxicity on eukaryotic cells and photo-induced antibacterial efficacy.17,18
To introduce four N-methyldiethanol ammonium groups onto the upper rim of a calix[4]arene skeleton blocked in a cone conformation (Scheme 1), we reacted the tetra-propoxy-p-chloromethyl-calix[4]arene derivative (1), prepared by a procedure described in the literature,19 with N-methyldiethanolamine in THF as the solvent. Compound 2 (Scheme 1) was obtained in high yield (86%) and characterized by NMR and high-resolution ESI-MS spectrometry. The pattern of the signals in the NMR and HR ESI-MS spectra were indicative of an exhaustive tetra-functionalization of the calix[4]arene upper rim. Dynamic light scattering measurements showed that in water at the concentration used in this work, the calix[4]arene derivative (2) did not form aggregates despite its amphiphilic structure.
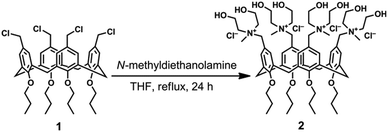 |
| Scheme 1 Synthesis of polycationic calix[4]arene derivative 2 from tetra-propoxy-p-chloromethyl-calix[4]arene derivative 1. | |
Calix[4]arene derivative 2 in combination with antibiotics
Interestingly, we observed that compound 2 speeded up the dissolution of ofloxacin, chloramphenicol, or tetracycline in water, when it was added to each antibiotic in a 1
:
1 molar ratio, and reduced the degradation of tetracycline in water at 37 °C. Fig. 1 shows that the concentration of tetracycline alone and in the presence of calix[4]arene derivative 2 decreases following an exponential decay. After 7 days of incubation, the amount of tetracycline in the presence of calix[4]arene 2 was more than double that of tetracycline alone. A half-life of 3.0 and 8.7 days was calculated for tetracycline alone and in the presence of calix[4]arene derivative 2, respectively, corresponding to an enhancement of the tetracycline half-life of about three-fold. This behavior can be associated with the noted ability of calix[4]arene derivatives to establish noncovalent host-guest interactions with a variety of ions and neutral molecules. Enhancing the stability of tetracycline is very appealing to improve the pharmaceutical formulation of this antibiotic. As an example, tetracycline for ophthalmic application is marked as an extemporaneous preparation undergoing rapid degradation once reconstituted.
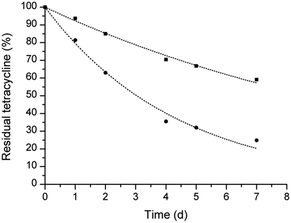 |
| Fig. 1 Stability of tetracycline in water at 37 °C alone (circle) and in the presence of calix[4]arene derivative 2 (square). | |
Antibacterial activity of calix[4]arene derivative 2 alone and in combination with antibiotics
The antibacterial activity of compound 2 was tested by a microdilution method. The MIC and MBC values, summarized in Table 1, showed that calix[4]arene derivative 2 possesses intrinsic antibacterial activity. Compound 2 was more effective against Staphylococcus strains (MIC 3.9 μg mL−1 for both ATCC and antibiotic-resistant strains) than P. aeruginosa ones (MIC 125 μg mL−1 for ATCC and 250 μg mL−1 for antibiotic-resistant isolate 1). The higher biocide effect against Gram positive compared to Gram negative bacteria agrees with the known major susceptibility of Gram positive bacteria versus ammonium quaternary salts.20 Regarding the mechanism of action, it is plausible that analogously to other polycationic calixarene derivatives,21–23 the antibacterial activity of calix[4]arene derivative 2 is related to the perturbation of the bacterial membrane. Polycationic compound 2 can establish electrostatic interactions with the negatively charged bacterial surface, and hydrogen bonds and hydrophobic interactions involving OH groups and aromatic rings and alkyl chains, respectively, can also occur in the interaction of compound 2 with the bacterial membrane. Compared to antibiotics, compound 2 was more effective than ofloxacin and chloramphenicol against all the resistant strains: Staphylococci MRSA 15 and MRSE 57 and P. aeruginosa isolate 1 (see Table 1). Compound 2 showed MIC 131-fold and MBC 262-fold lower than ofloxacin against MRSA 15 and MRSE 57; MIC and MBC 16-fold higher than ofloxacin were instead found against the ATCC strains of S. aureus and S. epidermidis.
Table 1 Minimum inhibitory concentration (MIC) and minimum bactericidal concentration (MBC) of calix[4]arene derivative 2 and antibiotics (μg mL−1), MIC (μg mL−1) and fractional inhibitory concentration index (FICI) of calix[4]arene 2/antibiotic combinations
Strain |
Calixarene 2 |
OFL |
Calixarene 2 + OFL |
CAM |
Calixarene 2 + CAM |
TET |
Calixarene 2 + TET |
MIC |
MBC |
MIC |
MBC |
MICa |
FICIb |
MIC |
MBC |
MICa |
FICIb |
MIC |
MBC |
MICa |
FICIb |
OFL = ofloxacin; CAM = chloramphenicol; TET = tetracycline.
Concentration of calix[4]arene 2 and antibiotic, respectively, at the MIC of their combination.
FICI <0.5 (Synergy), 0.5–1.0 (Additivity), 1.0–2.0 (Indifference).
|
S. aureus ATCC 6538 |
4 |
8 |
0.25 |
0.5 |
1 + 0.25 |
1.25 |
4 |
8 |
4 + 0.5 |
1.12 |
0.125 |
4 |
4 + 0.06 |
1.48 |
MRSA 15 |
4 |
8 |
512 |
>2048 |
4 + 1 |
1 |
8 |
128 |
4 + 0.5 |
1.06 |
1 |
16 |
4 + 0.03 |
1.03 |
S. epidermidis ATCC 35984 |
4 |
8 |
0.25 |
0.5 |
1 + 0.25 |
1.25 |
16 |
128 |
4 + 0.5 |
1.03 |
0.5 |
8 |
4 + 0.03 |
1.06 |
MRSE 57 |
4 |
8 |
512 |
>2048 |
4 + 1 |
1 |
8 |
256 |
4 + 0.5 |
1.06 |
1 |
16 |
4 + 0.03 |
1.03 |
P. aeruginosa ATCC 9027 |
128 |
256 |
2 |
2 |
8 + 2 |
1.06 |
64 |
128 |
64 + 8 |
0.62
|
16 |
32 |
128 + 1 |
1.06 |
P. aeruginosa isolate 1 |
256 |
256 |
256 |
512 |
128 + 32 |
0.62
|
512 |
1024 |
128 + 16 |
0.53
|
16 |
32 |
128 + 1 |
0.56
|
Analogously, calix[4]arene 2 showed similar MIC and 2-fold lower MBC than ofloxacin against resistant P. aeruginosa isolate 1 and MIC 62-fold and MBC 125-fold higher than ofloxacin against the ATCC strain.
Compared to chloramphenicol, calix[4]arene 2 showed similar antibacterial activity against S. aureus ATCC 6538 but higher activity than chloramphenicol against S. aureus MRSA 15 (MIC 2-fold and MBC 16-fold lower), S. epidermidis ATCC 35984 (MIC 4-fold and MBC 16-fold lower) and S. epidermidis MRSE 57 (MIC 2-fold and MBC 32-fold lower). Regarding the P. aeruginosa strains, calix[4]arene 2 was more effective than chloramphenicol against isolate 1 (MIC 2-fold and MBC 4-fold lower), but it was less effective against P. aeruginosa ATCC 9027 (MIC and MBC 2-fold higher). Notably, the susceptibility to calix[4]arene derivative 2 was independent of the antibiotic susceptibility status of the tested bacteria. Calix[4]arene derivative 2 was less effective than tetracycline against all the analyzed strains (Table 1).
The antibacterial activities of compound 2 in combination with ofloxacin, chloramphenicol or tetracycline were analyzed by a checkerboard test. By this approach, Grare and coworkers demonstrated additive, synergistic or indifferent effects for guanidinium calix[4]arene derivatives combined with a variety of antibiotics.24 The results, shown in Table 1, in accordance with the criteria established for interpreting the effects of combinations of antibiotics, showed an indifferent or additive effect. Although none of the calix[4]arene/antibiotic combinations yielded a synergistic effect, no antagonism was observed.
All the combinations resulted in an indifferent effect against S. aureus and S. epidermidis strains; an additive effect was instead observed against P. aeruginosa isolate 1 (FICI 0.53–0.62 range) as evidenced by 1/2, 1/8, 1/32, and 1/16 reduction of the initial MIC of calix[4]arene derivative 2, ofloxacin, chloramphenicol and tetracycline alone, respectively. On P. aeruginosa ATCC 9027, an additive effect was only observed for the calix[4]arene 2/chloramphenicol combination (FICI 0.62) in which the initial MIC of calix[4]arene derivative 2 and chloramphenicol decreased by 1/2 and 1/8, respectively. The additive effect observed on P. aeruginosa strains could be related to an enhancement of the antibiotic uptake into the bacterial cell mediated by calix[4]arene derivative 2 that, with its charges and three-dimensional organization, can disorganize the bacterial membrane by interactions with the negatively charged ultrastructures of Gram negative bacteria (i.e. phospholipids and lipopolysaccharides). This mechanism of action on antibiotic-resistant P. aeruginosa strains was demonstrated for a polycationic guanidinium calix[4]arene derivative,25 which interestingly showed no side effects on eukaryotic cells.21
Conclusion
In summary, we designed and synthesized a new water-soluble polycationic macrocyclic compound (2) built onto a calix[4]arene scaffold and demonstrated its potential as an antibacterial agent against Gram positive and Gram negative bacteria, including ATCC and resistant strains, alone and in combination with known antibiotics. Compound 2 could be a new antibacterial drug with a structure different from that of the currently used antibiotics. The ability of calix[4]arene derivative 2 to enhance the stability of tetracycline in water solution is also noteworthy. This feature could be exploited to improve the pharmaceutical formulation of this antibiotic which undergoes rapid degradation in aqueous medium.
Experimental section
Materials and methods
Reagents and solvents were purchased from Sigma-Aldrich and used without purification. Ofloxacin, chloramphenicol and tetracycline were purchased from Sigma-Aldrich in the form of sterile powder. Staphylococcus aureus ATCC 6538, S. epidermidis ATCC 35984 and P. aeruginosa ATCC 9027 were purchased from LGC Standards. Methicillin-resistant S. aureus (MRSA) 15, methicillin-resistant S. epidermidis (MRSE) 57 and P. aeruginosa isolate 1 were isolated from clinical ocular swabs.15 TLC analyses were carried out using aluminum sheets precoated with silica gel 60 F254, and UV radiation was used for detection. 1H NMR (400.13 MHz) and 13C NMR (100.61 MHz) spectra were acquired using a Bruker Avance™ 400 spectrometer. Chemical shifts (δ) are expressed in parts per million (ppm) and reported relative to the residual proton solvent peak; coupling constant (J) values are given in Hz. High-resolution (HR) ESI-MS spectra were recorded using a Q Exactive (Orbitrap) mass spectrometer (Thermo Fisher Scientific Instruments). Sample solutions were introduced into the ESI source on a 100 mm internal diameter fused silica via a 500 mL syringe. Full MS scans in the m/z range 300–2000 were acquired with an MS resolution of 150
000 FWHM (at m/z 400) in the Orbitrap. The experimental conditions for spectra acquired in the positive ion mode were: spray voltage = 3.5 kV, capillary temperature = 250 °C, m/z range = 300–2000, S-lens RF level = 60 V, and sheath gas = 5. The molecular species were detected as clusters of peaks because of the isotopic distribution of elements.
Tetra-propoxy-p-chloromethyl calix[4]arene 1 was synthesized by following the procedure reported in ref. 19.
Synthesis and characterization of calix[4]arene derivative 2
A solution of N-methyldiethanolamine (37 μL, 323 μmol) in THF (0.4 mL) was added to a stirring solution of tetra-propoxy-p-chloromethyl calix[4]arene 119 (60 mg, 76 μmol) in THF (1.5 mL). The reaction mixture was refluxed for 24 h. After cooling, the suspension was diluted with THF (5 mL) and diethyl ether (15 mL) and centrifuged (4000 rpm, 5 min). The precipitate was washed with a mixture of THF (2 mL) and diethyl ether (8 mL) and then with diethyl ether (3 × 5 mL) by repeated centrifugation (4000 rpm, 5 min) and removal of the supernatant. The solid was dried under vacuum for 24 h to give a white powder (83 mg, 86% yield). 1H-NMR (MeOD): δ 1.05 (t, 12H, J = 7.4 Hz, 4 × CH3), 2.00 (m, 8H, J = 7.4 Hz, 4 × CH2), 2.96 (s, 12H, 4 × NCH3), 3.35–3.42 (m overlapped, 12H, 2 × ArCH2Ar and 4 × CH2N), 3.49 (m, 8H, 4 × CH2N), 3.96 (t, J = 7.4 Hz, 8H, 4 × OCH2), 4.02 (br s, 16H, 8 × CH2OH), 4.53 (s, 8H, 4 × ArCH2N), 4.54 (d, 4H, J = 10.7 Hz, 2 × ArCH2Ar), 7.05 (s, 8H, 8 × ArH). 13C-NMR (MeOD): δ 10.7 (q), 24.5, 31.4, 56.8, 64.2, 69.0, 78.5 (t), 122.9 (d), 135.1, 136.9, 159.7 (s). HR ESI-MS: (m/z) calcd for C64H104Cl3N4O12+ [M-Cl−]+ = 1225.671, found [M-Cl−]+ = 1225.670; calcd for C64H104Cl2N4O122+ [M-2Cl−]2+ = 595.351, found [M-2Cl−]2+ = 595.351.
Stabilization of tetracycline by calix[4]arene derivative 2
Two water solutions of tetracycline (0.35 mg mL−1) alone and in the presence of calix[4]arene derivative 2 (1 mg mL−1, tetracycline
:
calix[4]arene molar ratio 1
:
1) were prepared to compare the tetracycline stability over time at 37 °C. The amount of tetracycline was monitored at different time intervals by using a Dionex HPLC system (P680 pump, ASI-100 autosampler, UVD170U detector, TCC-100 temperature-controlled column compartment) and a Phenomenex Luna 5 μm C18 reverse-phase column (250 × 4.6 mm). Eluents A: 0.1% formic acid in CH3CN, B: 0.1% formic acid in water, gradient: A from 5% to 30% in 25 min, then isocratic A 30%, 5 min, flow 1 mL min−1, T = 40 °C, λ = 270 nm. 25 μL of sample were diluted with 25 μL of MeOH and 425 μL of water, and then 50–100 μL of diluted sample were injected.
The half-life of tetracycline alone and in the presence of calixarene 2 was derived from eqn (1) and (2), respectively, which as a function of time t fits the experimental data:
| Residual tetracycline (%) = 100e−t/4.38 R2 = 0.990 | (1) |
| Residual tetracycline (%) = 100e−t/12.6 R2 = 0.992 | (2) |
Determination of minimum inhibitory concentration (MIC) and minimum bactericidal concentration (MBC)
The MIC of the calix[4]arene derivative (2) and antibiotics were determined by a microbroth dilution technique in 96-well polystyrene microtiter plates (Costar, Corning) according to the guidelines of the National Committee for Clinical Laboratory Standards.26 The overnight bacteria in Mueller Hinton broth (MHB) were inoculated in a 96-well plate to yield a final inoculum of 5 × 105 CFU mL−1. Each of the bacterial strains was tested against calix[4]arene derivative 2 (1–1024 μg mL−1), ofloxacin (0.06–2048 μg mL−1), chloramphenicol (1–2048 μg mL−1) and tetracycline (0.03–128 μg mL−1). The microtiter plates were incubated at 37 °C for 24 h and the MIC was defined as the lowest concentration of antibacterial agent that inhibited visible growth. All determinations were performed in triplicate and growth control in MHB was included. The MBC was determined by seeding 15 μL from all clear MIC wells in a ground plate of Mueller Hinton agar. The MBC was defined as the lowest concentration that allowed no microbial growth after incubation at 37 °C for 24 h. The data from at least three replicates were evaluated and the modal results were calculated.
Checkerboard assay
Combinations of calix[4]arene derivative 2 and antibiotics were tested by the checkerboard method. The final concentration of the antibacterial agents ranged from 2 to 0.015 times the MIC. Thus, 64 different combinations were obtained in 96-well-microplates. The inoculum of each bacterial strain was prepared in MHB and was added to the wells at a final concentration of 5 × 105 CFU mL−1.
After incubation at 37 °C overnight, the fractional inhibitory concentration index (FICI) was determined according to formula (3):
where FIC
A = MIC
A in combination/MIC
A alone and FIC
B = MIC
B in combination/MIC
B alone. The combination value was derived from the highest dilution of antibacterial combination permitting no visible growth. The results were interpreted as synergistic when the FICI was ≤0.5, as additive when between 0.5 and 1.0, as indifferent when between 1.0 and 2.0 and as antagonistic when the index was ≥2.0.
27
Conflicts of interest
The authors declare no competing interests.
Acknowledgements
The authors would like to thank PON R&C 02_00355_2964193 (MIUR, Rome).
References
- U. Theuretzbacher, F. Van Bambeke, R. Cantón, C. Giske, J. R. Mouton, L. Nation, M. Paul, J. D. Turnidge and G. Kahlmeter, J. Antimicrob. Chemother., 2015, 70, 2177–2181 CrossRef CAS PubMed.
-
P. Neri, J. L. Sessler and M.-X. Wang, Calixarenes and Beyond, Springer, Berlin-Heidelberg, 2016 Search PubMed.
- A. W. Coleman, S. Jebors, S. Cecillon, P. Perret, D. Garin, D. Marti-Battle and M. Moulin, New J. Chem., 2008, 32, 780–782 RSC.
-
(a) B. Mokhtari and K. Pourabdollah, J. Inclusion Phenom. Macrocyclic Chem., 2012, 73, 1–15 CrossRef CAS;
(b) M. D. Shah and Y. K. Agrawal, J. Sci. Ind. Res., 2012, 71, 21–26 CAS.
- R. Lamartine, M. Tsukadab, D. Wilsonc and A. Shirata, C. R. Chim., 2002, 5, 163–169 CrossRef CAS.
- H. M. Dibama, I. Clarot, S. Fontanay, A. B. Salem, M. Mourer, C. Finance, R. E. Duval and J. B. Regnouf-de-Vains, Bioorg. Med. Chem. Lett., 2009, 15, 2679–2681 CrossRef PubMed.
- M. Mourer, H. M. Dibama, S. Fontanay, M. Grare, R. E. Duval, C. Finance and J.-B. Regnouf-de-Vains, Bioorg. Med. Chem., 2009, 17, 5496–5509 CrossRef CAS PubMed.
- M. Grare, H. Massimba Dibama, S. Lafosse, A. Ribon, M. Mourer, J.-B. Regnouf-de-Vains, C. Finance and R. E. Duval, Clin. Microbiol. Infect., 2010, 16, 432–438 CrossRef CAS PubMed.
- G. M. L. Consoli, G. Granata, V. Cafiso, S. Stefani and C. Geraci, Tetrahedron Lett., 2011, 52, 5831–5834 CrossRef CAS.
- I. Di Bari, R. Picciotto, G. Granata, A. R. Blanco, G. M. L. Consoli and S. Sortino, Org. Biomol. Chem., 2016, 14, 8047–8052 CAS.
- I. Di Bari, A. Fraix, R. Picciotto, A. R. Blanco, S. Petralia, S. Conoci, G. Granata, G. M. L. Consoli and S. Sortino, RSC Adv., 2016, 6, 105573–105577 RSC.
- E. K. Stephens, Y. Tauran, A. W. Coleman and M. Fitzgerald, Chem. Commun., 2015, 51, 851 RSC.
- J. V. deAssis, M. G. Teixeira, C. G. P. Soares, J. F. Lopes, G. S. L. Carvalho, M. C. S. Lourenço, M. V. de Almeida, W. B. de Almeida and S. A. Fernandes, Eur. J. Pharm. Sci., 2012, 47, 539–548 CrossRef CAS PubMed.
- X. Wang, C. Luo, Z. Lv and F. Lu, J. Lumin., 2011, 131, 1986–1990 CrossRef CAS.
- V. Papa, A. R. Blanco and M. Santocono, J. Cataract Refractive Surg., 2016, 42, 1312–1317 CrossRef PubMed.
- E. Julián, A. Baelo, J. Gavaldà and E. Torrents, PLoS One, 2015, 10, e0122049 Search PubMed.
- G. Granata, I. Paterniti, C. Geraci, F. Cunsolo, E. Esposito, M. Cordaro, A. R. Blanco, S. Cuzzocrea and G. M. L. Consoli, Mol. Pharmaceutics, 2017, 14, 1610–1622 CrossRef CAS PubMed.
- R. V. Rodik, A.-S. Anthony, V. I. Kalchenko, Y. Mélya and A. S. Klymchenko, New J. Chem., 2015, 39, 1654–1664 RSC.
- M. Almi, A. Arduini, A. Casnati, A. Pochini and R. Ungaro, Tetrahedron, 1989, 45, 2177–2182 CrossRef CAS.
- R. Bragg, A. Jansen, M. Coetzee, W. van der Westhuizen and C. Boucher, Adv. Exp. Med. Biol., 2014, 808, 1–13 CrossRef CAS PubMed.
- M. Grare, M. Mourer, S. Fontanay, J.-B. Regnouf-de-Vains, C. Finance and R. E. Duval, J. Antimicrob. Chemother., 2007, 60, 575–581 CrossRef CAS PubMed.
- B. Korchowiec, M. Gorczyca, E. Rogalska, J.-B. Regnouf-de-Vains, M. Mourer and J. Korchowiec, Soft Matter, 2016, 12, 181–190 RSC.
- M. Mourer, R. E. Duval, C. Finance and J.-B. Regnouf-de-Vains, Bioorg. Med. Chem. Lett., 2006, 16, 2960–2963 CrossRef CAS PubMed.
-
M. Grare, et al., WO2012140365, 2012.
- C. Formosa, M. Grare, E. Jauvert, A. Coutable, J.-B. Regnouf-de-Vains, M. Mourer, R. E. Duval and E. Dague, Sci. Rep., 2012, 2, 1–9 Search PubMed.
-
Clinical and Laboratory Standards Institute, Methods for dilution antimicrobial susceptibility tests for bacteria that grow aerobically; approved standard, Wayne, PA, 7th edn, Document M7-A7, CLSI, 2009 Search PubMed.
-
G. M. Eliopoulos and R. C. Moellering, Antimicrobial combinations in Antibiotics in laboratory medicine, ed. V. Lorian, Williams & Wilkins, Baltimore, MD, 4th edn, 1996, p. 330 Search PubMed.
Footnote |
† Electronic supplementary information (ESI) available: NMR and HR ESI-MS spectra of calix[4]arene derivative 2. See DOI: 10.1039/c7md00527j |
|
This journal is © The Royal Society of Chemistry 2018 |
Click here to see how this site uses Cookies. View our privacy policy here.