DOI:
10.1039/C8FO01227J
(Paper)
Food Funct., 2018,
9, 6298-6306
Blueberry, combined with probiotics, alleviates non-alcoholic fatty liver disease via IL-22-mediated JAK1/STAT3/BAX signaling†
Received
27th June 2018
, Accepted 17th October 2018
First published on 31st October 2018
Abstract
Non-alcoholic fatty liver disease (NAFLD) is one of the most prevalent diseases worldwide. Blueberry, combined with probiotics (BP), might be a potential candidate for NAFLD treatment, due to its anti-inflammatory and anti-apoptotic properties. Here, we investigated whether the anti-inflammatory cytokine, IL-22, was involved in the therapeutic process of BP, using cell and rat models of NAFLD. Results indicated that BP significantly reduced lipid droplets and triglyceride (TG) accumulation in NAFLD cells. However, when IL-22 was deficient, the lipid droplets and TG content were significantly increased. In vivo, the serum parameters and pathological degree of NAFLD rats were significantly improved by BP, while IL-22 silencing significantly abolished the BP effect. Immunohistochemistry, immunofluorescence, qRT-PCR, and western blotting showed that the NAFLD group expressed significantly lower levels of IL-22, JAK1, and STAT3, and higher levels of BAX, than the normal group. Furthermore, BP significantly elevated the levels of IL-22, JAK1 and STAT3, and reduced the level of BAX in NAFLD, while IL-22 silencing prevented BP from restoring the expression of JAK1, STAT3, and BAX. We conclude that IL-22 is vital for the therapeutic effect of BP, and acts via activation of JAK1/STAT3 signaling and inhibition of the apoptosis factor BAX, which makes IL-22 a promising target for therapy of NAFLD.
Introduction
Non-alcoholic fatty liver disease (NAFLD) is one of the most common liver diseases worldwide, characterized by lipid accumulation in the liver, with no established efficient therapy.1 The clinical burden of NAFLD is largely associated with liver related morbidity, for instance, nonalcoholic fatty hepatitis and liver cirrhosis, and the development of fatal and nonfatal cardiovascular diseases.2 Recently, the effect of immune inflammation on NAFLD has started to be taken seriously. Once the intrahepatic immune cells are stimulated by bacteria, viruses, and drugs or other metabolites, the LPS/TLR4 signaling pathway induces the activation of pro-inflammatory factors and anti-inflammatory factors.3 The pro-inflammatory cytokines can activate fat synthesis, which leads to the accumulation of triglycerides and cholesterol in liver cells, and results in NAFLD. Interleukin-22 (IL-22) is a member of the anti-inflammatory cytokine IL-10 family.4 A previous study found that IL-22 has potential therapeutic effects in alcoholic fatty liver disease due to its anti-apoptotic, anti-microbial, and anti-oxidative functions, mediated via the activation of Janus kinase 1 (JAK1)/signal transducer and activator of transcription 3 (STAT3) signaling pathways.5 However, whether IL-22 is involved in NAFLD or not is largely unknown.
As intestinal microecological regulators, probiotics can effectively reduce endotoxemia and improve the intestinal barrier function, and are thought to play a therapeutic role in the treatment of NAFLD.6,7 Additionally, blueberries are common fruits that are rich in trace elements, flavonoids, vitamins, and especially polyphenols, such as anthocyanins, which have anti-oxidative, anti-inflammatory and immune regulatory properties. It has been shown that blueberries play a crucial role in liver protection and liver fibrosis reversal.8,9 Moreover, we have earlier reported that a combination of blueberries and probiotics (BP) can effectively reduce liver inflammation.10
Here, the therapeutic mechanism of BP in NAFLD was investigated by exploring the roles of IL-22, the JAK1/STAT3 signaling pathway, and the apoptotic regulator, Bcl-2-associated X protein (BAX).
Materials and methods
Ethics
All the protocols in this study were approved by the animal care and ethical committee of Affiliated Hospital of Guizhou Medical University. All animal-handling procedures were performed according to the Guide for the Care and Use of Laboratory Animals of the National Institutes of Health and followed the guidelines of the Animal Welfare Act.
Preparation of BP
Blueberries were obtained from Majiang Blueberry Plant (Guiyang, China). Blueberry juice was obtained in light of a previous study.11 Briefly, 1 kg blueberry blend was thawed at 4 °C for 8 h and crushed using a Braun Global Hand Blender MR300 (De'Longhi Kenwood A.P.A Ltd, Hong Kong, China). The milled blueberries were pressed under the maximum pressure of 0.9 MPa in a bag press mode. The prepared blueberry juice was gavaged to rats immediately. The main composition of the blueberry juice was detected as in a previous study.12Table 1 shows the main chemical characterisation of blueberry juice and the anthocyanidin concentration was 0.98 ± 0.07 (mg mL−1). Dry probiotic tablets mixed with probiotic species of Bifidobacterium infantis, Bifidobacterium animalis and Lactobacillus acidophilus were obtained from China General Microbiological Culture Collection Center (Beijing, China). Dried probiotic tablets (each tablet contained 5 × 106 CFU ml−1 bacteria) were ground into a powder, and to each 10 ml of blueberry juice was added a portion of probiotics, and incubated at 4 °C. The live bacteria in the juice were monitored as follows. After incubation for 6 h, 12 h, and 24 h, 0.01 ml of the blueberry probiotic mixture was taken and the total number of colonies was calculated directly under a microscope. Probiotic survival rate (%) = lg
N1/lg
N0 × 100% (N1: number of live bacteria (CFU ml−1) after treatment and N0: initial quantity of live bacteria (CFU ml−1)). The number of live bacteria at 6 h, 12 h, and 24 h was 5.9 × 107, 6.3 × 107, and 5.2 × 108 (CFU ml−1), respectively (Table S1†). BP with a concentration of 108 CFU ml−1 probiotics was used for further study.
Table 1 Main chemical characterisation of blueberry juice
Items |
Value |
pH |
3.20 ± 0.03 |
Total anthocyanidins (mg mL−1) |
0.98 ± 0.07 |
Total phenolic acid (mg mL−1) |
2.54 ± 0.16 |
Total flavonols (mg mL−1) |
1.18 ± 0.06 |
Vitamin C (μg mL−1) |
171.40 ± 3.0 |
Preparation of blueberry probiotic serum (BPS)
Male Wistar rats (weight: 180 ± 20 g) of clean grade were purchased from the Animal Experiment Center of Guizhou Medical University (SCXK – (Guizhou) 2012–2012), and kept in separate cages and allowed free feeding with the standard diet. Rats were randomly divided into two groups: the physiological saline control group and the blueberry probiotic group (1 mL per 100 g). Gavage was administered 2 times per day, for 5 days. Two hours after the last gavage, rats were gavaged again, and 1 h later, blood was collected from the femoral artery, and centrifuged at 3000 rpm for 20 min. Complement components of the obtained serum were inactivated in a water bath at 50 °C for 30 min. Thereafter, bacterial contaminants were removed by filtration, using a 0.22 μm Millipore filter, and stored at −20 °C.
Establishment of a NAFLD cell model
Human normal liver cell line L-02 was cultured in 90% Dulbecco's modified Eagle's medium (DMEM) containing 10% fetal bovine serum (FBS), 100 U mL−1 penicillin, 100 mg L−1 streptomycin, and 1% non-essential amino acids, at 37 °C under 5% CO2. NAFLD cells were established by treatment with 1 mmol L−1 of a mixed free fatty acid (oleic acid (OA)
:
palmitic acid (PA) = 2
:
1).
Cell treatment
Cells were divided into five groups: normal group, free fatty acid + siRNA negative control group (FFA-NC), free fatty acid + IL-22 siRNA group (FFA-IL-22si), free fatty acid + siRNA negative control + BPS group (FFA-NC-BPS), and free fatty acid + IL-22 siRNA + BPS group (FFA-IL-22si-BPS). Cells were normally cultured for 24 h, after which, the medium was discarded, and fresh medium containing 10% saline–serum was added to the normal group, while the other groups were transfected with siRNA reagents (primers are shown in Table S1†). After transfection for 24 h, saline–serum was added to the FFA-NC group and the FFA-IL-22si group, while BPS was added to the FFA-NC-BPS group and the FFA-IL-22si-BPS group. After 24 h, 1 mmol L−1 of the mixed free fatty acid was added to all groups, except for the normal group, and cultured for 24 h.
Oil red O (ORO)
Cells were seeded into 6-well plates, and cell slides were prepared and fixed with 4% paraformaldehyde (PFA) for 15 min, and stained with ORO for 30 min in a dark room. The cell slides were washed with 60% isopropyl alcohol and stained with hematoxylin for 10 s, and observed under a microscope.
Immunofluorescence
Immunostaining was performed according to a previous study.13 In brief, cells were fixed with 4% PFA for 30 min, and permeabilized with 0.5% Triton X-100 for 20 min at 20 °C. Primary antibodies (1
:
100), including anti-IL-22 (Abcam, Cambridge, UK, ab5984), anti-JAK1 (Abcam, ab138005), anti-STAT3 (Abcam, ab76315) and anti-BAX (Abcam, ab32503) were added and incubated at 4 °C, overnight. Slides were washed and incubated with FITC or Alexa Fluor 594 labeled goat–anti-rabbit IgG secondary antibodies for 2 h. The cells were visualized using an Olympus FV 1000 (Olympus, Tokyo, Japan).
Rat model
Forty male Sprague-Dawley rats (200 to 250 g) were purchased from the Animal Center of Guiyang Medical College (Approval Number SCXK (Guizhou) 2002–0001, Guiyang, China), and were randomly divided into five groups: (1) normal group, which received a standard diet; (2) NC group, in which rats were fed with a high-fat diet (HFD) and treated with lentivirus carrying siRNA NC; (3) NC-BP group, in which HFD rats were treated with siRNA NC and BP; (4) IL-22 siRNA group, in which HFD rats were treated with IL-22 siRNA; and (5) IL-22 siRNA-BP group, in which HFD rats were treated with IL-22 siRNA and BP. Except for the normal group, rats were injected with lentivirus carrying siRNA (virus titer of 1 × 108, at a time) on alternate days, for 12 weeks. Thereafter 3 rats from each group were randomly selected to confirm the NAFLD model and the siRNA effect, and BP groups were gavaged with BP (once a day, 1.5 mL per 100 g weight, containing probiotics at a concentration of 108 CFU mL−1 and an anthocyanin amount of 1.47 mg per 100 g weight, per time) for 8 weeks. Thereafter rats were kept fasting for 12 hours, after which they were weighed and sacrificed, and the blood and liver were sampled.
Biochemical analysis
The intracellular plasma triglyceride (TG) was tested using a biochemical analyzer (Beijing Jinji Aomeng Co., Ltd, Beijing, China), and the total protein was measured using a Pierce BCA Protein Assay Kit (catalog 23225; Life Technologies). The serum levels of aspartate aminotransferase (AST) and alanine aminotransferase (ALT) were also determined using a Biochemical Analyzer (Siemens Advia 1650; Siemens, Bensheim, Germany). Serum TG, total cholesterol (TC), high-density lipoprotein–cholesterol (HDL), and low-density lipoprotein–cholesterol (LDL) levels were measured using the biochemical analyzer manufactured by Beijing Jinji Aomeng Co., Ltd, Beijing, China.
Histological analysis
Liver tissues were fixed in 10% neutral formaldehyde for paraffin-embedded sections, followed by hematoxylin and eosin (HE) staining and ORO staining, and observed under an A12.1503 microscope (Opto-Edu (Beijing) Co., Ltd, Beijing, China). The pathological diagnosis was evaluated according to the guidelines set out by the National Institutes of Health.15,16 Hepatic steatosis and inflammation were evaluated according to the NAFLD activity score (NAS) system shown in Table S2.† The fatty cells from 10 fields were randomly selected for calculation of the number and area.
Immunohistochemical staining
Immunohistochemical staining was performed on sections from paraffin embedded liver tissues. The IL-22, JAK1 and STAT3 anti-mouse monoclonal antibodies were diluted to 1
:
500. The BAX anti-rabbit monoclonal antibody was diluted to 1
:
2000. The sections were stained with DAB and re-dyed with hematoxylin. Images were captured via an Olympus BX41, the optical density was measured using a MIAS (MIAS 2000, Olympus, Tokyo, Japan), and the mean optical density for each section was calculated from five randomly visualized fields, under 400×.
Western blotting
The total protein concentration was measured using a BCA assay kit (Pierce Biotechnology, Inc., Rockford, IL, USA). Equivalent concentrations of samples were run on 10% SDS-PAGE gel and transferred to polyvinylidene fluoride membranes (Millipore, Bedford, MA, USA). Membranes were blocked and incubated with primary antibodies (1
:
1000; the antibodies were the same as those used for the immunofluorescence assays) for 12 hours at 4 °C, followed by incubation with a secondary antibody for 2 hours. Proteins were photographed via a Chemi Doc MP system (Bio-Rad, Hercules, USA), and analyzed with ImageJ.
Quantitative reverse transcription-PCR (qRT-PCR)
Hepatic tissues were collected for RNA extraction using Trizol, and reverse transcribed using a PrimeScript™ RT reagent Kit with a gDNA Eraser. qRT-PCR was performed using an SYBR Premix Ex Taq™ (TaKaRa, Tokyo, Japan) on an ABI one-step fast thermocycler (Applied Biosystems, Paisley, UK). The qRT-PCR conditions used were: 1 cycle at 95 °C for 10 min, followed by 40 cycles at 95 °C for 5 s, and annealing and extension at 55–58 °C for 30 s. Each group was analyzed in triplicate. The primers are shown in Table S2.† Actin was used as the internal reference. Data were obtained by the 2−ΔΔCT method.
Statistical analysis
Data were analyzed via SPSS 19.0 (SPSS Inc., Chicago, IL, USA) and shown as mean ± standard deviation (SD). The groups were compared by one-way ANOVA, and P < 0.05 was considered a statistically significant difference.
Results
BP reduced lipid deposition via IL-22 in vitro
Normal liver cells showed a normal morphology, and no lipid droplets were deposited in the cytoplasm (Fig. 1A). With FFA treatment, the cells displayed an irregular or elliptic shape, and red lipid droplets were ubiquitously present in the cytoplasm, while the BPS treatment significantly decreased the red lipid droplets (Fig. 1B and C). Moreover, knockdown of IL-22 significantly increased the red lipid droplets in FFA treated cells, and the IL-22 siRNA significantly blocked the restoration effect of BPS, as evidenced by the lipid increase in the FFA-IL-22si-BPS group, compared to the FFA-NC-BPS group (Fig. 1C–E). Additionally, a significant accumulation of TG contents was observed in the NAFLD cells compared to those in the normal cells, while BPS significantly reduced the TG contents, and IL-22 deficiency completely abolished the BPS effect (Fig. 1F, P < 0.05).
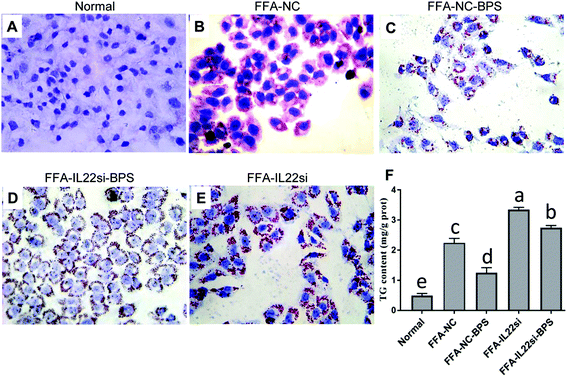 |
| Fig. 1 BP reduced lipid deposition via IL-22 in L-02 cells. (A) Oil red O staining indicated the red lipid droplets in each group, ×400. (B) Triglyceride (TG) concentration in each group. Data are expressed as TG/total protein, per gram of cells (P < 0.05, one way ANOVA, n = 3). | |
BP activated JAK1/STAT3 signaling via IL-22 in vitro
The expression level of IL-22 in the FFA-NC group was significantly decreased compared to that in the normal group (Fig. 2A–D). After treatment with BPS, the expression level of IL-22 was significantly increased in the FFA-NC-BPS group compared to that in the FFA-NC group, indicating that IL-22 might be involved in the treatment process of BPS. In addition, the IL-22 signal in the FFA-IL-22si and FFA-IL-22si-BPS groups was remarkably lower than that in the FFA-NC and FFA-NC-BPS groups respectively, suggesting that the IL-22 siRNA successfully silenced IL-22 in the NAFLD model cells. Interestingly, the expression levels of STAT3 and JAK1 were significantly decreased in the FFA-NC group, compared to those in the normal group (P < 0.05). With BPS treatment, the expression of STAT3 and JAK1 in the FFA-NC-BPS group was restored to approximately normal levels compared to the normal group (P < 0.05). However, IL-22 silencing significantly inhibited BPS from restoring the expression of STAT3 and JAK1 (P < 0.05). Similarly, the FFA-IL-22si group expressed lower levels of STAT3 and JAK1, compared to the FFA-NC group (P < 0.05).
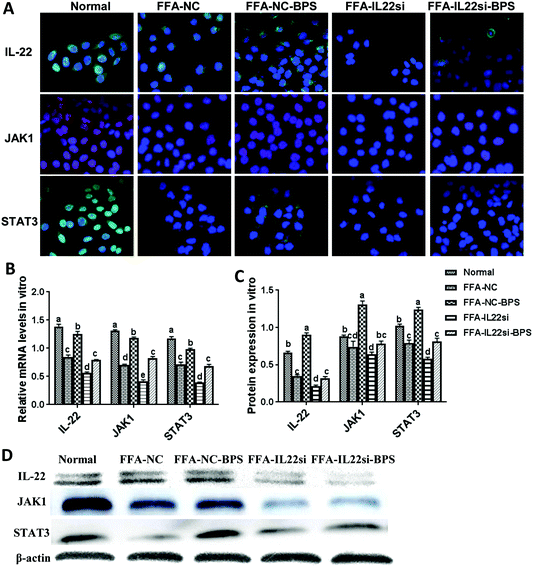 |
| Fig. 2 BP increased the expression of JAK1 and STAT3 via IL-22 in L-02 cells. (A) Immunofluorescence analysis of IL-22, JAK1, and STAT3 in each group, ×400. (B) mRNA expression levels of IL-22, JAK1, and STAT3 in each group, by qPCR. Samples were normalized to actin mRNA levels. (B, C) Western blot of IL-22, JAK1, and STAT3. Optical density measurements analyzed by ImageJ. Different lowercase letters (a, b, c, d) represent significant differences (P < 0.05, one way ANOVA, n = 3). | |
BP decreased BAX via IL-22 in vitro
The mRNA and protein levels of BAX were significantly increased in the FFA-NC group, compared to those of the normal group (Fig. 3, P < 0.05). However, with BPS treatment, the expression of BAX in the FFA-NC-BPS group was decreased compared to that in the FFA-NC group (P < 0.05). Moreover, IL-22 silencing significantly prevented BPS from decreasing the expression of BAX (P < 0.05). Similarly, the expression level of BAX in the FFA-IL-22si group was significantly increased compared to that in the FFA-NC group (P < 0.05).
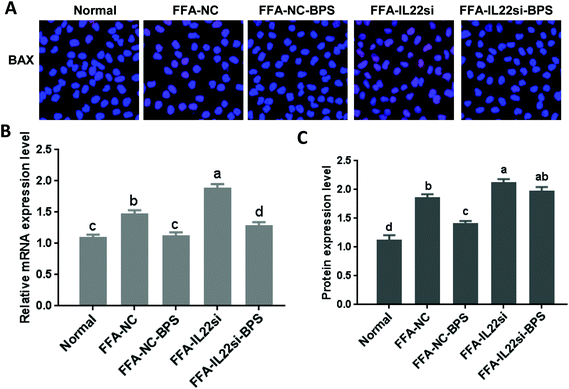 |
| Fig. 3 BP decreased the expression of BAX via IL-22 in L-02 cells. (A) Immunofluorescence analysis of BAX, ×400. (B) qPCR analysis of BAX. (C) Western blot of BAX. Different lowercase letters (a, b, c, and d) represent significant differences (P < 0.05, one way ANOVA, n = 3). | |
BP improved enzymology indicators of NAFLD rats via IL-22
The serum levels of ALT and AST in NC rats were significantly higher than those in the normal rats (Fig. 4A, P < 0.05). After silencing of IL-22, the levels of ALT and AST were significantly increased in the NAFLD rats (P < 0.05). Moreover, the ALT and AST levels in the NC-BP group were significantly lower than those in the NC group (P < 0.05). However, the IL-22 siRNA diminished the effect of BP, since the levels of ALT and AST in the IL-22si-BP group were higher than those in the NC-BP group. In addition, compared to the normal rats, the NC rats had higher TG, TC, and LDL, but lower HDL (P < 0.05, Fig. 4B). Furthermore, the serum levels of TG, TC, and LDL were significantly increased, and that of HDL was significantly reduced in the IL-22si rats compared to the respective levels in the NC rats (P < 0.05). The serum TG, TC, and LDL levels of NC-BP rats were lower, and that of HDL was higher than those of NC rats (P < 0.05). Inversely, the serum TG, TC, and LDL levels were increased, and HDL was decreased in IL-22si-BP rats compared to those in the NC-BP rats. In particular, there was no significant difference in the TC and LDL levels between the NC and IL-22si-BP groups.
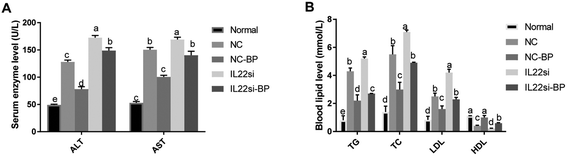 |
| Fig. 4 Serum biochemical indices in rats. (A) Enzyme indicators in each group. (B) Serum lipids in each group. The different lowercase letters (a, b, c, d, and e) represent significant differences (P < 0.05, one way ANOVA, n = 5). | |
BP improved pathological feature of NAFLD rats via IL-22
HE staining suggested that the hepatic lobules of normal rats were complete and clear (Fig. 5A). The central vein was large and thin, and was surrounded by liver cells, which were radially and orderly distributed. The hepatic sinus was clearly visible, the size was consistent, the nuclear structure was clearly visible, and there was no fatty degeneration. The NC group showed swollen hepatocytes with microbubble fatty degeneration, accompanied by balloon-like degeneration, and neutrophil and lymphocyte infiltrates were observed. Importantly, the hepatocyte fatty degeneration in the NC-BP group was significantly decreased compared to that in the NC group, while the fatty degeneration was significantly increased in the IL-22si-BP group compared to that in the NC-BP group. Similarly, ORO staining showed that the lipid droplets in the NC rats were significantly increased compared to those in the normal rats, while the BP treatment significantly reduced the lipid droplets (Fig. 5B). The lipid droplets in the IL-22si-BP rats were significantly increased compared to those in the NC-BP rats. Additionally, NAS integration was performed for liver tissues (Fig. 5C). The NAS of the NC group was significantly higher than that of the normal group (P < 0.01). With BP treatment, the NAS of the NC-BP group was significantly lower than that of the NC group (P < 0.01). Furthermore, the integration of the IL-22si group was significantly higher than that of the NC group (P < 0.01), and the score of the IL-22si-BP group was significantly higher than that of the NC-BP group (P < 0.05). In addition, the NC group displayed a larger area of adipose cells than the normal group, while BP treatment significantly decreased the area of adipose cells (Table S3†). However, the IL-22 siRNA dramatically blocked the effect of the BP adipose tissue area.
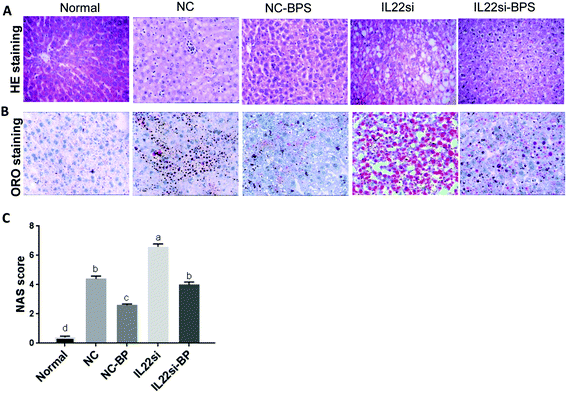 |
| Fig. 5 NAFLD related pathological analysis in liver tissues. (A) Hematoxylin and eosin (HE) staining analysis of each group. (B) Oil red O staining of liver tissues. (C) NAFLD activity scoring (NAS) of each group. Different lowercase letters (a, b, c, d) represent significant differences (P < 0.05, one way ANOVA, n = 5). | |
BP alleviates NAFLD with IL-22 via JAK1/STAT3/BAX
Immunohistochemistry results showed that the signals of IL-22, JAK1, and STAT3 in the NC rats were significantly weaker than those in the normal rats, while the BAX signal was stronger in the NC rats, compared to that in the normal rats (Fig. 6A and B, P < 0.05). With BP treatment in the NAFLD rats, the IL-22, JAK1, and STAT3 signals were significantly increased, while the BAX signal was found to be decreased in the liver tissue (P < 0.05). Moreover, the signals of JAK1 and STAT3 were decreased and BAX was increased in the IL-22si-BP rats compared to those in the NC-BP rats (P < 0.05). Additionally, qRT-PCR and western blotting showed that the mRNA and protein levels of IL-22, JAK1, and STAT3 were significantly lower, and BAX was significantly higher in the liver tissue of NAFLD rats than in that of the normal rats (Fig. 6C and D, S1,†P < 0.05). However, the BP treatment partly restored the expression of IL-22, JAK1, STAT3, and BAX in the NAFLD rats, close to the levels of the normal rats. Moreover, the IL-22 siRNA decreased the levels of IL-22, JAK1, and STAT3, and increased the level of BAX in the NAFLD rats (P < 0.05). As expected, the expression of IL-22, JAK1, and STAT3 was lower, and that of BAX was higher in the IL22si-BP rats than in the NC-BP rats (P < 0.05).
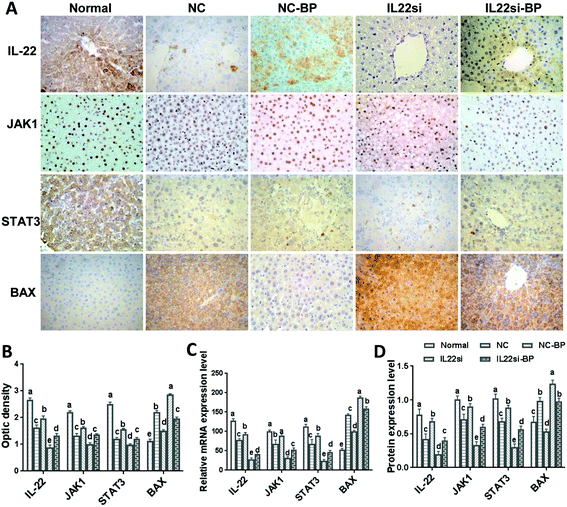 |
| Fig. 6 Expression levels of IL-22, JAK1, STAT3, and BAX in liver tissues of each group. (A, B) Immunohistochemical analysis of IL-22, JAK1, STAT3, and BAX in each group, ×400. (C) qPCR analysis of IL-22, JAK1, STAT3, and BAX mRNA expression in each group. Samples were normalized to actin mRNA. (D) Western blot of IL-22, JAK1, STAT3, and BAX. Different lowercase letters (a, b, c, d, and e) represent significant differences (P < 0.05, one way ANOVA, n = 5). | |
Discussion
At present, there is no efficient evidence-based drug for NAFLD, and dietary therapy is critical in any attempt to control the progress of NAFLD at the early stage. Similar to our results, a previous study has shown that blueberries have a potential inhibitory effect on NAFLD at the early stage.14 Blueberry treatment alleviates CCl4-induced liver disease via regulation of inflammation, oxidative stress, and stellate cell activation in mice.15,16 Blueberry extracts attenuate γ-radiation-induced hepatocyte damage via effecting oxidative stress and suppressing NF-κB in rats.17 Except for blueberries, other berries also display an alleviation effect on NAFLD. Consumption of bayberry juice can protect against NAFLD by improving the plasma antioxidant status and inhibiting the inflammatory and apoptotic responses in young adults.18 Jaboticaba berry peel induces higher production of gut SCFA, compounds known to counteract obesity markers, to prevent hepatic steatosis in mice fed a high-fat diet.19 Furthermore, several data exist to indicate that probiotics may be beneficial in the therapy of NAFLD, via regulating the intestinal microbiota, improving the function of the epithelial barrier, improving intestinal inflammation, and reducing oxidative and inflammatory liver damage.20 For instance, probiotics containing Bifidobacterium infantis, Lactobacillus acidophilus and Bacillus cereus suppress the progression of NAFLD via restoring the gut microbiota structure and reducing intestinal endotoxemia.21 Multi-probiotics (concentrated biomass of Bifidobacterium, Lactobacillus, Lactococcus, and Propionibacterium) reduce the fatty liver index, and cytokine and aminotransferase levels in NAFLD patients.22 However, little is known about the effect of BP. In our previous study, we have reported that BP can reduce the damage to hepatocytes caused by the action of inflammatory cytokines, regulate lipid metabolism and reduce hepatocyte adipose degeneration in vivo.23 In this study, we show that blueberries mixed with probiotics reduce the formation of lipid droplets, improve lipid indicators, and improve the pathology of fatty liver, both in vitro and in vivo, which further supports the alleviatory effect of BP on NAFLD.
It has recently been shown that IL-22 mediates beneficial metabolic and anti-inflammatory effects in NAFLD patients.24 As a member of anti-inflammatory cytokines, IL-22 can act as a receptor in the JAK/STAT signaling pathway and reduce the TG synthesis related genes by activating the STAT3 pathway to improve fatty liver, caused by obesity.25 Kong et al. found that IL-22 can activate the JAK1/STAT3 signaling pathway through its receptor, IL-22RA1, and down-regulate the expression of FATP to improve lipid degeneration of hepatocytes.26 The STAT3 signaling pathway induces numerous genes involved in various biological processes, such as apoptosis, fat synthesis, and cell-cycle progression. Increased IL-22 induced the activation of STAT3 in liver cells Moreover, it increased the expression of anti-apoptotic genes (b cell lymphoma-2 families, BCL-2), anti-oxidation genes, metallothionein 1 and 2, and mitochondrial DNA repair genes, Nei-like homolog 1 (Neil 1), and reduced the expression of genes related to fat formation (such as sterols regulating element binding protein, SREBP-1-c).27 Therefore, IL-22 is a key survival factor for liver cells as it can prevent and repair liver injury by activating STAT3 and subsequently cause up-regulation of a number of genes involved in anti-apoptosis and proliferation.28 Based on these pieces of evidence, we speculated that IL-22 may be involved in the therapeutic effect of BP on NAFLD through JAK1/STAT3 mediated anti-apoptosis, in liver cells. Using the IL-22 siRNA, we found that IL-22 deficiency partly abolished the mitigation capacities of BP for NAFLD. This indicated that IL-22 was involved in the therapeutic process of BP. We next investigated whether IL-22 mediated the therapeutic effect through activation of the JAK1/STAT3 signaling pathway. The results of immunohistochemistry, immunofluorescence, qRT-PCR, and western blot showed that the IL-22 siRNA decreased the expression of JAK1 and STAT3, both in NAFLD cells and in the rat model under BP treatment, suggesting that BP induces IL-22 to activate the JAK1/STAT3 signaling pathway in NAFLD.
To further explore whether the activation of STAT3 improves NAFLD by inducing anti-apoptosis in hepatocytes, we studied the role of the apoptosis regulator, BAX, in NAFLD. BAX was the first identified pro-apoptotic member of the Bcl-2 protein family.29 A previous study showed that blocking JAK1/STAT3 can induce the apoptosis of hepatic stellate cells via increasing the activation of BAX.30 Moreover, Liu et al. found that the increased BAX level accelerates apoptotic death in severe fatty degeneration.31 Similarly, our results showed that BAX was down-regulated in the NAFLD group. Furthermore, the IL-22 siRNA increased the expression of BAX, both in NAFLD cells and in a rat model under BP treatment. Overall, these pieces of evidence suggest that BP mitigates NAFLD, partly by inhibiting the apoptosis of hepatocytes via the IL-22 mediated JAK1/STAT3/BAX signaling pathway.
In conclusion, we have utilized detailed molecular approaches, and cell and rat models to demonstrate that the precise regulation of IL-22 in the liver is essential for the BP-mediated improvement of NAFLD. Moreover, BP might improve NAFLD partly through the inhibition of apoptosis via IL-22, mediating the JAK1/STAT3/BAX signaling pathway. This study further advances our understanding of the mechanisms underlying NAFLD, and can aid in the development of new therapeutic strategies.
Data accessibility
The datasets used and analyzed during the current study are available from the corresponding author on reasonable request.
Author contributions
Conceptualization, Mingliang Cheng; Data curation, Juanjuan Zhu; Investigation, Juanjuan Zhu, Mingyu Zhou, Xueke Zhao and Mao Mu; Project administration, Mingliang Cheng; and Writing – original draft, Juanjuan Zhu.
Conflicts of interest
The authors declare that they have no competing interests.
Funding
This work was supported by the Project of Guizhou Science and Technology Department (Guizhou support (2017)2874), and the Science and Technology Project of Guizhou Health and Family Planning Commission (gzwjkj2016-1-02).
Acknowledgements
We would like to thank all participants involved in this study.
References
- R. Loomba and A. J. Sanyal, The global NAFLD epidemic, Nat. Rev. Gastroenterol. Hepatol., 2013, 10(11), 686–690 CrossRef CAS PubMed.
-
Q. Anstee, A. Mantovani, H. Tilg and G. Targher, Risk of cardiomyopathy and cardiac arrhythmias in patients with nonalcoholic fatty liver disease, Nat. Rev. Gastroenterol. Hepatol., 2018157425–439 Search PubMed.
- S. T. Kolanowski, M. C. Dieker, S. N. Lissenbergthunnissen, G. M. van Schijndel, S. M. van Ham and B. A. Ten, TLR4-mediated pro-inflammatory dendritic cell differentiation in humans requires the combined action of MyD88 and TRIF, Innate Immun., 2014, 20(4), 423 CrossRef PubMed.
- S. S. Iyer and G. Cheng, Role of interleukin 10 transcriptional regulation in inflammation and autoimmune disease, Aerobiologia, 2012, 33(3), 1–11 Search PubMed.
- S. H. Ki, O. Park, M. Zheng, O. Morales-Ibanez, J. K. Kolls, R. Bataller and B. Gao, Interleukin-22 treatment ameliorates alcoholic liver injury in a murine model of chronic-binge ethanol feeding: Role of STAT3, Hepatology, 2010, 52(4), 1291 CrossRef CAS PubMed.
- V. W. Wong, G. L. Won, A. M. Chim, W. C. Chu, D. K. Yeung, K. C. Li and H. L. Chan, Treatment of nonalcoholic steatohepatitis with probiotics. A proof-of-concept study, Ann. Hepatol., 2013, 12(2), 256–262 CAS.
- T. Eslamparast, H. Poustchi, F. Zamani, M. Sharafkhah, R. Malekzadeh and A. Hekmatdoost, Synbiotic supplementation in nonalcoholic fatty liver disease: a randomized, double-blind, placebo-controlled pilot study, Hepatitis Mon., 2016, 16(1), e34897 Search PubMed.
- Y. Wang, M. Cheng, B. Zhang, F. Nie and H. Jiang, Dietary supplementation of blueberry juice enhances hepatic expression of metallothionein and attenuates liver fibrosis in rats, PLoS One, 2013, 8(3), e58659 CrossRef CAS PubMed.
- Y. Wang, M. Cheng, B. Zhang, M. Mu and J. Wu, Effects of blueberry on hepatic fibrosis and transcription factor Nrf2 in rats, World J. Gastroenterol., 2010, 16(21), 2657–2663 CrossRef CAS PubMed.
- J. Zhu, T. Ren, M. Zhou and M. Cheng, The combination of blueberry juice and probiotics reduces apoptosis of alcoholic fatty liver of mice by affecting SIRT1 pathway, Drug Des., Dev. Ther., 2016, 10, 1649–1661 CrossRef CAS PubMed.
- M. Rossi, E. Giussani, R. Morelli, S. Rlo, R. C. Nani and D. Torreggiani, Effect of fruit blanching on phenolics and radical scavenging activity of highbush blueberry juice, Food Res. Int., 2003, 36(9), 999–1005 CrossRef CAS.
- G. Q. Wen, F. Nie and Y. J. Liao, Comparative analysis of physicochemical component content in blueberry fruit and their function evaluation, Acta Agric. Jiangxi, 2012, 24(1), 117–119 CAS.
- P. Xia, S. Wang, G. Huang, Y. Du, P. Zhu, M. Li and Z. Fan, RNF2 is recruited by WASH to ubiquitinate AMBRA1 leading to downregulation of autophagy, Cell Res., 2014, 24(8), 943 CrossRef CAS PubMed.
- S. Vendrame, A. Daugherty, A. S. Kristo, P. Riso and D. Klimis-Zacas, Wild blueberry (Vaccinium angustifolium) consumption improves inflammatory status in the obese Zucker rat model of the metabolic syndrome, J. Nutr. Biochem., 2013, 24(8), 1508–1512 CrossRef CAS PubMed.
- W. Zhan, X. Liao, T. Tian, L. Yu, X. Liu, B. Li, J. Liu, B. Han, R. J. Xie, Q. H. Ji and Q. Yang, Study on the effects of blueberry treatment on histone acetylation modification of CCl4-induced liver disease in rats, GMR, Genet. Mol. Res., 2017, 16(1) DOI:10.4238/gmr16019188.
- J. Sun, Y. Wu, C. Long, P. He, J. Gu, L. Yang, Y. Liang and Y. Wang, Anthocyanins isolated from blueberry ameliorates CCl4 induced liver fibrosis by modulation of oxidative stress, inflammation and stellate cell activation in mice, Food Chem. Toxicol., 2018, 120, 491–499 CrossRef CAS PubMed.
- M. I. Alkhaklaf and F. K. Khalifa, Blueberry extract attenuates γ-radiation-induced hepatocyte damage by modulating oxidative stress and suppressing NF-κB in male rats, Saudi J. Biol. Sci., 2018, 25(7), 1272–1277 CrossRef CAS.
- H. Guo, R. Zhong, Y. Liu, X. Jiang, X. Tang, Z. Li, M. Xia and W. Ling, Effects of bayberry juice on inflammatory and apoptotic markers in young adults with features of non-alcoholic fatty liver disease, Nutrition, 2014, 30(2), 198–203 CrossRef CAS PubMed.
- Â. G. Batista, J. K. da Silva-Maia, M. C. P. Mendonça, E. S. Soares, G. C. Lima, S. Bogusz Junior, M. A. da Cruz-Höfling and M. R. Maróstica Júnior, Jaboticaba berry peel intake increases short chain fatty acids production and prevent hepatic steatosis in mice fed high-fat diet, J. Funct. Foods, 2018, 48, 266–274 CrossRef.
- S. M. Ferolla, C. A. Couto and T. C. A. Ferrari, Probiotics as a complementary therapeutic approach in nonalcoholic fatty liver disease, World J. Hepatol., 2015, 7(3), 559–565 CrossRef PubMed.
- L. Xue, J. He, N. Gao, X. Lu, M. Li, X. Wu, Z. Liu, Y. Jin, J. Liu, J. Xu and Y. Geng, Probiotics may delay the progression of nonalcoholic fatty liver disease by restoring the gut microbiota structure and improving intestinal endotoxemia, Sci. Rep., 2017, 7, 45176 CrossRef CAS PubMed.
- N. Kobyliak, L. Abenavoli, G. Mykhalchyshyn, L. Kononenko, L. Boccuto, D. Kyriienko and O. Dynnyk, A Multi-strain Probiotic Reduces the Fatty Liver Index, Cytokines and Aminotransferase levels in NAFLD Patients: Evidence from a Randomized Clinical Trial, J. Gastrointestin. Liver Dis., 2018, 27(1), 41–49 Search PubMed.
- T. Ren, J. Zhu, L. Zhu and M. Cheng, The Combination of Blueberry Juice and Probiotics Ameliorate Non-Alcoholic Steatohepatitis (NASH) by Affecting SREBP-1c/PNPLA-3 Pathway via PPAR-α, Nutrients, 2017, 9(3), 198 CrossRef PubMed.
- A. Abdulla, C. Reynolds and H. A-Kader, Non-alcoholic fatty liver disease (NAFLD): The search for a cure, Eur. Med. J., 2016, 1(2), 93–100 CAS.
- L. Yang, Y. Zhang, L. Wang, F. Fan, L. Zhu, Z. Li, X. Ruan, H. Huang, Z. Wang and Z. Huang, Amelioration of high fat diet induced liver lipogenesis and hepatic steatosis by interleukin-22, J. Hepatol., 2010, 53(2), 339–347 CrossRef CAS PubMed.
- X. Kong, D. Feng, S. Mathews and B. Gao, Hepatoprotective and anti-fibrotic functions of interleukin-22: therapeutic potential for the treatment of alcoholic liver disease, J. Gastroenterol. Hepatol., 2013, 28(Supplement S1), 56–60 CrossRef CAS PubMed.
- S. Radaeva, R. Sun, H. N. Pan, F. Hong and B. Gao, Interleukin 22 (IL-22) plays a protective role in T cell-mediated murine hepatitis: IL-22 is a survival factor for hepatocytes via STAT3 activation, Hepatology, 2004, 39(5), 1332–1342 CrossRef CAS PubMed.
- C. Pan, J. Tang, X. Wang, F. Wu, J. Ge and F. Chen, Role of Interleukin-22 in liver diseases, Inflammation Res., 2014, 63(7), 519–525 CrossRef CAS PubMed.
- Z. N. Oltval, C. L. Milliman and S. J. Korsmeyer, Bcl-2 heterodimerizes in vivo with a conserved homolog, Bax, that accelerates programmed cell death, Cell, 1993, 74(4), 609–619 CrossRef.
- Y. J. Gu, W. Y. Sun, S. Zhang, X. R. Li and W. Wei, Targeted blockade of JAK/STAT3 signaling inhibits proliferation, migration and collagen production as well as inducing the apoptosis of hepatic stellate cells, Int. J. Mol. Med., 2016, 38(3), 903–911 CrossRef CAS PubMed.
- K. Liu, J. Lou, T. Wen, J. Yin, B. Xu, W. Ding, A. Wang, D. Liu, C. Zhang and D. Chen, Depending on the stage of hepatosteatosis, p53 causes apoptosis primarily through either DRAM-induced autophagy or BAX, Liver Int., 2013, 33(10), 1566–1574 CAS.
Footnote |
† Electronic supplementary information (ESI) available. See DOI: 10.1039/c8fo01227j |
|
This journal is © The Royal Society of Chemistry 2018 |
Click here to see how this site uses Cookies. View our privacy policy here.