DOI:
10.1039/C7FO01147D
(Paper)
Food Funct., 2018,
9, 279-293
Structural elucidation and immunostimulatory activity of a new polysaccharide from Cordyceps militaris
Received
28th July 2017
, Accepted 3rd November 2017
First published on 8th November 2017
Abstract
A new polysaccharide (CMPB90-1) was isolated from cultured Cordyceps militaris by alkaline extraction. The chemical structure of CMPB90-1 was determined by analysis of physicochemical and spectral data. The backbone of CMPB90-1 is composed of (1→6)-linked α-D-glucopyranosyl and (1→3)-linked α-D-glucopyranosyl residues, with branching at O-6, which consists of (1→4)-linked β-D-mannopyranosyl and (1→6)-linked α-D-glucopyranosyl residues, respectively. β-D-Galactopyranosyl residues is the terminal unit. In vitro immunomodulatory assay revealed that CMPB90-1 promoted proliferation of splenic lymphocytes, enhanced cytotoxicity of NK cells and promoted lymphocyte secretion of the cytokine interleukin-2. Besides, CMPB90-1 upregulated T-cell subpopulation, strengthened phagocytosis function of macrophages and induced their M1 polarization. The mechanism of the effects might be due to the activation of TLR2, MAPK and NF-κB pathways. The results proposed that CMPB90-1 can be researched and developed as a new functional food.
1. Introduction
Natural mushrooms have been used as functional foods for more than two thousand years.1Cordyceps militaris has been one of the most famous functional mushroom for revitalizing various systems of the body from ancient times in Asia. Besides the popular application of these constituents as functional foods by local communities,2 many beneficial effects of C. militaris are attributed to polysaccharides. The results of many investigations have shown that polysaccharides in this fungus possess various biological effects, including immunomodulatory, antitumor and antioxidative effects.2–5
Immunity is defined as the ability of an organism to protect against diseases by identifying and destroying foreign harmful substances.6 The host immune response is composed of innate immunity and adaptive immunity in a living organism, and it relies on some important immune organs.7 The spleen is the most important organ for antibacterial and antifungal immune reactivity, which combines the innate and adaptive immune system in a uniquely organized way.8 The spleen is composed of T and B lymphocytes and natural killer (NK) cells. T and B lymphocytes are two important classes of immunologically active cells. The former is mainly responsible for cellular immunity, and the latter is the only cell capable of producing antibodies.9 NK cells are an important group of immune cells, serving as effector lymphocytes that control several types of tumors and microbial infections by limiting their spread and subsequent tissue damage.10
The innate immunity is the first line of defense against infections.7 It is renowned that the innate immune system mainly contains macrophages, monocytes and granulocytes.11 Among these components, macrophages play a vital role in innate host defense against external invaders and malignancies due to their phagocytic, cytotoxic and intracellular killing activities.12 However, macrophages may undergo polarized activation to play their respective functions depending on the different activation signals.13 One is classically activated macrophages (M1), which are considered to mediate host defense against microbial infections and tumors. M1 macrophages are characterized by elevated expression of cluster of differentiation (CD) 86 and major histocompatibility complex (MHC) class II and generation of tumor necrosis factor alpha (TNF-α), reactive oxygen species (ROS) and nitric oxide (NO). Another is alternatively activated macrophages (M2), which are involved in immune tolerance and tumor progression. M2 macrophages are associated with transforming growth factor beta (TGF-β), cell surface expression of CD163 or CD206. For some diseases like cancer, the proportion of M2 macrophages is high, which contributes to immune tolerance. Generally speaking, M1 macrophages are considered to mediate host defense to infections and have more a powerful function in removing external invaders versus M2 macrophages.14,15
Some studies have shown that polysaccharides administered orally can activate the immune system in the small intestine. Orally administered polysaccharides can be absorbed either through M cells or through binding to the projected tips of dendritic cells (DCs) in the follicle-associated epithelium (FAE) of Peyer's patches and subsequently bind to dectin-1 and toll-like receptor 2 (TLR2) to trigger immune response.16 Additionally, orally applied nondigestible carbohydrates have been associated with immune-modulating effects and other health benefits.17 Thus, polysaccharides can activate the intestinal immune system by oral administration, which creates favorable conditions for its application as a functional food.
As far as we know, there are no reports on the immunoregulatory activities of alkali-soluble polysaccharides from C. militaris, and few studies on the immunomodulatory activity of polysaccharides have focused on the different macrophage phenotypes. Isolation and characterization of polysaccharides from C. militaris and the evaluation of their immunomodulatory activities on the immune response is urgently required to research and develop new natural functional foods.
In this paper, we reported alkaline extraction, purification and structural characterization of a novel low-molecular-weight polysaccharide (CMPB90-1) from the cultured fruiting bodies of C. militaris. Furthermore, the immunostimulatory effect of CMPB90-1 was evaluated in vitro, and the mechanism of inducing M1 polarization of macrophages through activation of TLR2, mitogen-activated protein kinase (MAPK) and nuclear factor kappa B (NF-κB) pathways was also investigated.
2. Materials and methods
2.1 Plant material
The cultured fruiting bodies of C. militaris were obtained from Jiangmen Honghao Bioscience and Technology Corporation, Jiangmen, China. The materials were identified by Professor Rongmin Yu, College of Pharmacy, Jinan University, China.
2.2 Experimental reagents and materials
DEAE-52 cellulose and Sephadex G-25 were obtained from Whatman Ltd. Sephacryl S-300 HR was purchased from Amersham Biosciences. AB-8 macroporous adsorption resin was obtained from Sigma Chemical Co. (St Louis, MO, USA). Dimethylsulfoxide (DMSO), 3-(4,5-dimethylthiazol-2-y1)-2,5-diphenyltetrazolium bromide (MTT), trypan, concanavalin A (Con A), penicillin G, FITC-dextran, polymyxin B (PMB) and streptomycin were obtained from Sigma Chemical Co. (St Louis, MO, USA). A Griess reagent kit was obtained from Beyotime Corp (China). PE anti-mouse MHC II, FITC anti-mouse CD206, FITC anti-mouse CD86, FITC anti-mouse CD3, PE anti-mouse CD4 and PE anti-mouse CD8a were obtained from Biolegend Corp (Shenzhen, Guangdong, China). RPMI 1640 medium, DMEM and fetal bovine serum (FBS) were purchased from Gibco Invitrogen Corp (San Diego, CA, USA). ELISA test kits for murine TNF-α and interleukin-2 (IL-2) were obtained from R&D Systems (Santa Monica, CA, USA). Propidium Iodide (PI) dye was obtained from BD Biosciences Corp (Franklin Lakes, NJ, USA). An ECL western blotting detection kit was obtained from Tanon Crop (Shanghai, China). Anti-TLR2, anti-TLR4, anti-phospho-NF-κB p65, anti-NF-κB p65, anti-phospho-IKBα, anti-ERK (ERK), anti-phospho-ERK (p-ERK), antil-JNK (JNK), anti-phospho-JNK (p-JNK), anti-p38 (p38), and antiphospho-p38 (p-p38) primary antibodies (rabbit monoclonal antibodies) were provided by Cell Signaling Technology (Beverly, MA, U.S.A.). All other reagents used in the experiments were of analytical grade.
2.3 Animals and cells
Kunming mice (grade II, SCXK 2011-0029, 20–25 g, 6–8 weeks old) were obtained from the Experimental Animal Center, Sun Yat-sen University, China. Animals were acclimatized for at least 7 days prior to use and maintained in a temperature-controlled environment (22 ± 2 °C) with a 12 h light–dark cycle and free access to water and standard rodent chow. The RAW264.7 macrophage cell lines and YAC-1 lymphoma cell lines were obtained from the Cell Bank of Type Culture Collection of the Chinese Academy of Sciences (Shanghai, China). The YAC-1 lymphoma cell lines were maintained in RPMI 1640 medium supplemented with 100 IU mL−1 penicillin, 100 μg mL−1 streptomycin and 10% FBS at 37 °C under humidified air with 5% CO2. The RAW264.7 cell lines were maintained in DMEM supplemented with 100 IU mL−1 penicillin, 100 μg mL−1 streptomycin and 10% FBS at 37 °C under humidified air with 5% CO2. All experiments were performed in compliance with the relevant laws and institutional guidelines. The animal experiments were carried out in compliance with the Animal Management Rules of the Ministry of Health of the People's Republic of China and approved by the Animal Care and Use Committee of Jinan University.
2.4 Isolation of CMPB90-1
The dried fruiting bodies of C. militaris were pretreated with ethanol (7
:
1 w/v) to remove the fat and pigments, as described in our previous report.18 The dried residues were extracted with distilled water (10
:
1 w/v) and then 0.3 mol L−1 NaOH (25 °C) for 12 h. The extracts were combined and the pH adjusted to 7.00 with 1 M HCl and then concentrated to one-fourth of its original volume under reduced pressure. Ethanol was added to reach a final concentration of 80% (v/v) and then the mixture was kept in a beaker overnight at 4 °C and centrifuged at 5000g for 15 min to precipitate proteins and high-molecular-weight polysaccharides. The supernatant (CMPB90) was collected and lyophilized.
CMPB90 was purified using a column (2.6 × 95 cm) packed with AB-8 macroporous adsorption resin.19 Distilled water was employed as the mobile phase. The flow rate was 2 mL min−1. Each fraction (20 mL) was collected and analyzed with phenol–sulfuric acid reagent at 490 nm using a spectrophotometer. Fractions 5–18, which coincided with the major peak, were collected together, concentrated at 60 °C with a rotary evaporator under vacuum, dialyzed (Mw cut off: 500 Da) and lyophilized. The polysaccharide samples obtained by lyophilizing were dissolved in distilled water and fractionated on a DEAE-52 cellulose column (2.6 × 40 cm) equilibrated with distilled water and then a linear gradient from 0 to 1.0 M NaCl at a flow rate of 0.5 mL min−1. All the fractions were assayed for carbohydrate content by the phenol–sulfuric acid method and the fraction representing only one sharp peak was collected, dialyzed, concentrated and further purified using a Sephadex G-25 column (1.6 × 70 cm) by eluting with distilled water at a flow rate of 0.3 mL min−1. Consequently, a fine polysaccharide, named CMPB90-1, was obtained. CMPB90-1 with (c 1.0, H2O) was used in the following structural and bioactivity experiments.
2.5 Analysis of chemical composition
Total sugar content was determined by the phenol–sulfuric acid colorimetric method using glucose as the standard.20 Protein content was determined by using Bradford reagent and bovine serum albumin was set as the standard. The homogeneity and molecular weight of CMPB90-1 were evaluated by gel permeation chromatography (GPC) on a Sephacryl S-300HR column (1.6 × 70 cm) with standard dextrans (T-4, T-7, T-10, T-70, T-200 and blue dextran) and glucose. The elution volume was plotted against the logarithm of their respective molecular weights. The elution volume of CMPB90-1 was plotted in the same graph and the molecular weight was determined.21 The monosaccharide composition was analyzed by high-performance anion exchange chromatography (HPAEC) after hydrolization and UV detection, coupled with pulsed amperometric detection (PAD), equipped with a Carbo PAC™ PA10 (2.0 × 250 mm) column. Gas chromatography-mass spectrometry (GC-MS) was conducted with a Hewlett Packard 5895 instrument using a fused-silica capillary column (30 × 25 mm) coated with a 0.2 mm film of DB-5.
2.6 Spectroscopy analysis
Optical rotation was recorded with a Jasco P-1020 polarimeter. The Fourier transform infrared (FT-IR) spectroscopy spectra were recorded using a Tensor 27 Bruker instrument. The sample was ground with KBr powder and then pressed into pellets for FT-IR measurement in the frequency range of 4000–500 cm−1. One-dimensional (1D) and two-dimensional (2D) NMR spectra were recorded with a Bruker 500 MHz instrument and the sample was dissolved in D2O.
2.7 Analysis of monosaccharide composition
CMPB90-1 (5 mg) was hydrolyzed with 2 M trifluoroacetic acid (TFA, 2 mL) at 100 °C in a sealed tube for 8 h. Excess acid was removed by evaporation in a water bath at a temperature of 40 °C and co-distilled with MeOH after the hydrolysis was completed. The monosaccharide content was measured by HPAEC-PAD. The hydrolysate was dissolved in pure water (1 mL). The solution was used for ionic chromatography analysis by HPAEC-PAD on a Dionex ICS-2500 system, eluted with a mixture of water and 200 mM NaOH at a volume ratio of 92
:
8.
2.8 Partial acid hydrolysis
CMPB90-1 (15 mg) was hydrolyzed with 0.05 M TFA for 6 h at 100 °C and dialyzed (500 Da) with distilled water for 48 h. The fraction out of the sack was collected. After the excess TFA in the fraction was removed by co-distillation with MeOH (2 mL × 3), the fraction was evaporated to dryness (Fraction 1). The fraction in the sack was dried by evaporation and then hydrolyzed with 0.5 M TFA. The hydrolysate was dialyzed and the fraction out of the sack (fraction 2) and fraction in the sack (fraction 3) were collected. Fractions 1, 2 and 3 were hydrolyzed with 2 M TFA and tested by HAPEC-PAD.22
2.9 Methylation analysis
The sample was dried at 50 °C under vacuum overnight and was methylated by the referenced method.23 The methylated polysaccharide was treated with 90% formic acid (3 mL) for 10 h at 100 °C in a sealed tube. After removal of the formic acid, the residues were heated with 2 M TFA (2 mL) under the above conditions and the hydrolysate was concentrated to dryness. The methylated sugars were reduced with NaBH4 and then acetylated with acetic anhydride. Alditol acetates were analyzed by GC-MS.
2.10 Splenic lymphocyte proliferation assay
The MTT method was used to assay splenic lymphocyte proliferation. Briefly, spleens were aseptically removed from sacrificed mice with scissors and forceps in cold phosphate-buffered saline (PBS) and then minced in aseptic PBS. The splenocytes were obtained by pressing the spleen tissue through nylon mesh filters. The removal of blood cells in the splenocyte suspension was performed by washing cells with Tris-NH4Cl for 3 min. The isolated splenocytes were counted by trypan blue exclusion with viability of more than 95%, and the splenocytes were placed into 96-well flat-bottom microplates at 5 × 106 cells per mL in RPMI 1640 medium and treated with CMPB90-1 (31.2 to 500 μg mL−1) or concanavalin A (Con A). The negative and positive controls were treated with RPMI 1640 (10% FBS) medium and Con A (5 μg mL−1), respectively. The cells were incubated at 37 °C under 5% CO2 for 48 h, and then 20 μL of MTT (5 mg mL−1) was added to each well with further incubation for 4 h. After removal of MTT by centrifugation at 3000g for 15 min at 4 °C, the formazan precipitate was solubilized in DMSO (200 μL per well). The splenic lymphocyte proliferation was measured by determining the optical density (OD) at 570 nm using a multifunctional microplate reader.
2.11 Natural killer cell cytotoxicity assay
Lymphocytes were prepared as effector cells, whereas YAC-1 cells were used as target cells. The effector cells (1.2 × 107 cells per mL) were incubated for 48 h with CMPB90-1 ranging from 31.3 to 500 μg mL−1. The same volume of YAC-1 cells (2 × 105 cells per mL, effector cells/target cells = 60
:
1) was then added to the wells of 96-well round-bottom microwell plates in the sample group. In addition, the wells that contained only effector cells or target cells were considered as effector and target cell control groups, respectively. Each step was carried out in triplicate. After 4 h of incubation, the MTT method was used to detect natural killer (NK) cell activity. The OD value at 570 nm for each well was measured using a multifunctional microplate reader (Bio-Rad, USA). The percentage of NK cell cytotoxicity was calculated from the following formula:
NK cell cytotoxicity = [1 − (ODS − ODE)/ODT] × 100% |
where ODS refers to the OD value of the test sample; ODE refers to the OD value of the effector cell control, whereas ODT refers to the OD value of the target cell control.
2.12 Measurement of IL-2 production
The ability of CMPB90-1 to induce production of IL-2 in lymphocyte cells was determined after dissolving the polysaccharide in culture medium for 48 h. Supernatants were harvested and the concentration of IL-2 was determined using an ELISA kit, according to the manufacturer's protocol.
2.13 RAW264.7 cell viability assay
The effect of CMPB90-1 on the viability of RAW264.7 cells was determined by the MTT method. RAW264.7 cells (2 × 104 cells per mL) in DMEM were pre-incubated in a 96-well microplate for 12 h. Different concentrations of CMPB90-1 (31.2 to 500 μg mL−1) were prepared with serial dilutions and added to the cells incubating in the microplate wells. 1 μg ml−1 lipopolysaccharide was used as a positive control. After 48 h of incubation, 20 μL of the combined MTT (5 mg ml−1) was added into each well of the microplate. The plates were incubated for 4 h at 37 °C in a humidified 5% CO2 environment. The absorbance of formazan at 570 nm was measured directly from the 96-well microplate.
2.14 Flow cytometry
T-cell subpopulations and the proportions of CD3+, CD4+ and CD8+ T lymphocytes in the mice spleen lymphocytes were detected by flow cytometry according to Giraldo.24 The ratio of CD4+/CD8+ T lymphocyte was then calculated. When testing the phenotype of macrophages, single-cell suspensions were blocked in the presence of PE anti-mouse MHC II, FITC anti-mouse CD206 and FITC anti-mouse CD86 at 4 °C for 30 min. Ten thousand viable cells per treatment were analyzed using a BD FACScan flow cytometer.
2.15 Measurement of the production of nitric oxide and TNF-α
RAW264.7 cells were cultured in a 96-well plate at a density of 1 × 106 cells per mL for 12 h, and then incubated with different concentrations of CMPB90-1 (31.3 to 500 μg mL−1). Lipopolysaccharide (LPS, 1 μg ml−1) was used as a positive control. The nitrite accumulation was measured using the Griess reagent; this quantity is an indicator of NO production in the medium. NaNO2 was used as a standard to calculate the nitrite concentrations. Supernatants were harvested and the concentration of TNF-α was determined using an ELISA kit, according to the manufacturer's protocol.
To eliminate the interference of endotoxin, RAW264.7 cells (1 × 106 cells per well) were pre-incubated in 12-well plates for 12 h and then incubated with CMPB90-1 (500 μg ml−1), LPS (1 μg ml−1) and PMB (50 μg ml−1) for 24 h. After treatments, the amount of NO in the cultured supernatants was measured using the Griess reagent, as described above.
2.16 Phagocytosis assay
The phagocytic ability of the macrophages was measured using a neutral red uptake assay.25 Confluent cultures of RAW264.7 cells in 96-well plates were exposed to medium alone or with different concentrations of CMPB90-1 (62.5 to 500 μg mL−1) or LPS solution and incubated for 48 h at 37 °C. After removal of the supernatant, the non-adherent cells were removed by washing twice with PBS and 100 μL of neutral red solution was added to each well. The plates were incubated for 1 h and the supernatant was removed. To remove excess neutral red solution, the cells were washed with PBS twice. Subsequently, 100 μL of cell lysate (1.0 M acetic acid/ethanol = 1
:
1, v/v) was then added to each well. After incubation overnight at room temperature, the absorbance at 540 nm of each well was measured by a microplate reader.
To confirm the phagocytotic ability of macrophages, FITC-dextran internalization was detected by flow cytometry. Cells were collected at the end of the culture with CMPB90-1 and incubated with FITC-dextran (1 mg ml−1) at 37 °C for 1 h. The reaction was terminated by cold PBS containing 2% fetal serum. Cells were washed three times and resuspended in PBS containing 2% paraformaldehyde. The FITC-dextran internalization of cells was analyzed by flow cytometry.
2.17 Western blot assay
Cells were harvested, washed twice with PBS, lysed on ice for 30 min in 100 μL of lysis buffer (120 mM NaCl, 40 mM Tris, pH 8, 0.1% NP 40) and centrifuged at 13
000g for 15 min. The resultant supernatants were collected and protein concentrations were determined using the BCA assay. Equal amounts of total protein were separated by 10% sodium dodecyl sulfate polyacrylamide gel electrophoresis (SDS-PAGE) and transferred to a nitrocellulose membrane. The membranes were blocked with 5% skimmed milk in TBST for 2 h, which were then incubated with primary antibodies. The membranes were subsequently incubated with the secondary immunoglobulin G-horseradish peroxidase, which was conjugated and then developed by ECL. The western blot detection kits used were chosen to facilitate exposure of the signals to X-ray films.
2.18 Statistical analysis
All measurements were performed in triplicate, and all values are expressed as mean ± standard deviation. Statistical analysis was performed by an independent T test using statistical analysis software SPSS 11.5. P < 0.05 and P < 0.01 were considered to represent statistically significant differences.
3. Results and discussion
3.1 Isolation and chemical composition
Crude low-molecular-weight polysaccharides (9.23 g) were obtained from the cultured fruiting bodies of C. militaris by alkaline extraction followed by ethanol precipitation. After purification with AB-8 resin, DEAE-52 cellulose and a Sephadex G-25 column, the profile of CMPB90-1 appeared as a single, sharp, symmetric peak, which was detected by the phenol–sulfuric acid assay. The yield of CMPB90-1 from the crude low-molecular-weight polysaccharides was 46.68%. The uronic acid content of CMPB90-1 was below the detection limit. The average molecular weight of CMPB90-1 was determined as 5.8 kDa by GPC on a Sephacryl S-300 HR column. The total carbohydrate content of CMPB90-1 was 94.8% (w/w).
The protein content was determined using Bradford reagent and no protein was found in CMPB90-1.
3.2 Structural characterization of CMPB90-1
Three monosaccharides, galactose (Gal), glucose (Glc) and mannose (Man), were identified in the hydrolysate of CMPB90-1, and their ratios were determined by HAPEC-PAD analysis to be 3.04
:
1.00
:
1.45 (Fig. 1A and B). Fractions 1, 2 and 3 were obtained through partial acid hydrolysis. Each fraction was subjected to HAPEC-PAD analysis. The component present in fraction 3 (Fig. 1E) indicated that glucose might form the backbone structure of CMPB90-1. The analysis results of fractions 2 (Fig. 1D) and 1 (Fig. 1C) showed that the branched structure of CMPB90-1 was composed of glucose and mannose (the ratio was 2.89
:
1.00), and the chain terminated with galactose.
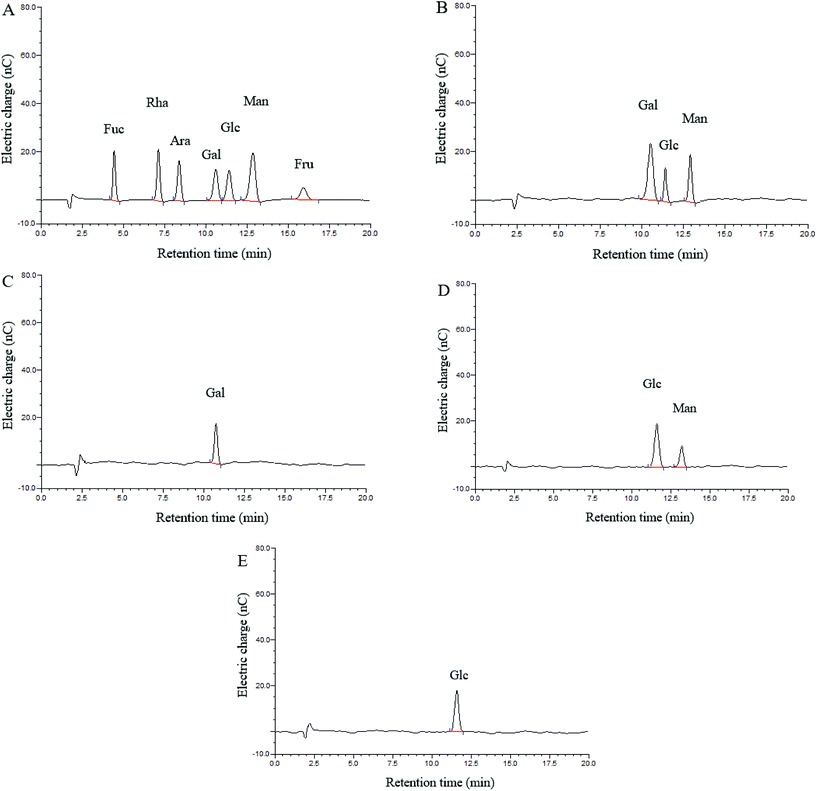 |
| Fig. 1 HPAEC-PAD chromatograms of CMPB90-1. (A) HPAEC-PAD chromatograms of standard monosaccharide. (B) HPAEC-PAD chromatograms of CMPB90-1. (C) HPAEC-PAD chromatograms of fraction 1. (D) HPAEC-PAD chromatograms of fraction 2. (E) HPAEC-PAD chromatograms of fraction 3. | |
The fully methylated CMPB90-1 was hydrolyzed with acid and analyzed by GC-MS. The results showed the presence of four components: 2,3,4,6-Me4-Gal, 2,3,6-Me3-Man, 2,3,4-Me3-Glc, and 2,4-Me2-Glc in a molar ratio of 2.98
:
1.30
:
1.00
:
0.78 (Table 1). Based on the standard data in the CCRC Spectral Database for PMAAs, the linkage of galactose was deduced as (1→), the linkage of mannose was deduced as (1→4) and the linkages of glucose as (1→6) and (1→3, 6). This result showed a good correlation between terminal and branched residues.
Table 1 GC-MS of methylated products of CMPB90-1
Methylation product |
Retention time (min) |
Molar ratio |
Mass fragments (m/z) |
Linkage type |
2,3,4,6-Me4-Gal |
11.50 |
2.98 |
45, 87, 101, 117, 145, 161, 205 |
T→ |
2,3,6-Me3-Man |
13.49 |
1.30 |
45, 87, 101, 113, 117, 161, 233 |
1→4 |
2,3,4-Me3-Glc |
15.02 |
1.00 |
43, 71, 101, 129, 145, 189, 205 |
1→6 |
2,4-Me2-Glc |
15.84 |
0.78 |
43, 71, 87, 117, 129, 159 |
1→3,6 |
The infrared spectra of CMPB90-1 revealed the major functional groups and chemical bonds of the purified product (Fig. 2). The broad band centered at 3335 cm−1 arose from OH stretching vibrations of hydroxyl groups. The weak band at 2940 cm−1 was due to C–H stretching vibrations of CH2. The strong absorption band at 1604 cm−1 was attributed to C–O asymmetric stretching vibrations. The signal at 1413 cm−1 was attributed to deforming vibrations of C–H. The absorption band at about 1095 cm−1 was assignable mainly to C–O–C stretching vibrations and C–O–H bending vibrations. The characteristic absorptions at 824 and 894 cm−1 indicated that α- and β-configurations were present simultaneously.26
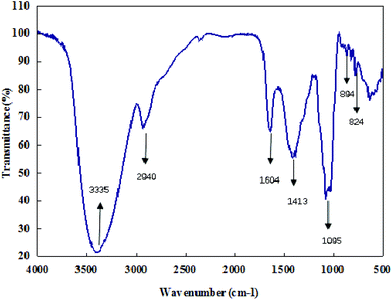 |
| Fig. 2 Infrared spectra of CMPB90-1. | |
3.3 NMR spectroscopy analysis
Signals of CMPB90-1 in 1D NMR (1H-NMR, 13C-NMR) and 2D NMR (HSQC and HMBC) spectra were assigned based on monosaccharide analysis, linkage analysis and chemical shifts.27–31 The 1H-NMR spectrum contained four signals at δ 4.85, 5.08, 4.53 and 5.00 ppm for the anomeric protons, indicating that four residues, designated as A, B, C and D, respectively, were present in CMPB90-1. In the 13C-NMR spectrum, there were four anomeric carbon signals at δ 105.01, 101.83, 102.84 and 98.31 ppm. Furthermore, the cross-peaks of δ 4.85/105.01, 5.08/101.83, 4.53/102.84 and 5.00/98.31 ppm were observed in the 2D HSQC spectrum, indicating that the anomeric carbon signals at δ 105.01, 101.83, 102.84 and 98.31 ppm corresponded to the anomeric proton signals at δ 4.85, 5.08, 4.53 and 5.00 ppm, respectively. The cross peaks of protons and carbons of each of the sugar moieties were also examined in the HSQC spectrum (Fig. 3a).
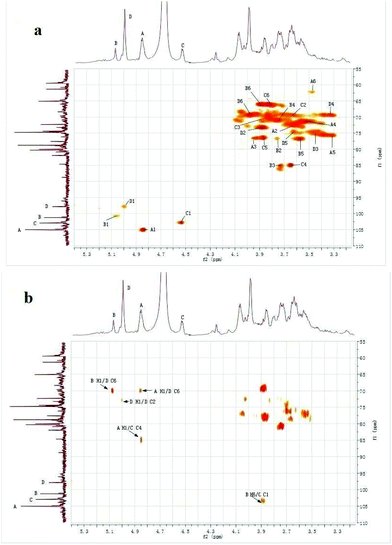 |
| Fig. 3
1H–13C spectra of CMPB90-1. (a) 2D 1H–13C HSQC spectrum of CMPB90-1. (b) 2D 1H–13C HMBC spectrum of CMPB90-1. | |
The observed chemical shifts larger than 5.0 ppm and small J-coupling constants of 3JH-1,H-2 for the anomeric protons of residues B and D suggest that these residues were α-linked, whereas the chemical shifts smaller than 5.0 ppm and relatively large J-coupling constant of 3JH-1,H-2 for the anomeric protons of residues A and C indicate that residues A and B were β-linked. The results showing the presence of α- and β-linked residues corroborated the results from FT-IR analysis.
The 2D HMBC spectrum (Fig. 3b) of CMPB90-1 showed strong cross-peaks between 1H and 13C peaks in different residues and could be assigned as follows: B H-1 (δ 5.08) and D C-6 (δ 69.91); A H-1 (δ 4.85) and D C-6 (δ 69.91); D H-1 (δ 5.00) and D C-2 (δ 73.05); A H-1 (δ 4.85) and C C-4 (δ 85.09); and B H-6 (δ 3.88) and C C-1 (δ 102.84). The cross peaks at δ 5.08/69.91 were assigned to the inter-residue B H-1, D C-6, indicating that residues B and D were connected to each other as B-(1→6)-D. The cross-peaks at δ 4.85/69.91 were assigned to the inter-residue A H-1, D C-6, indicating that residues A and D were connected to each other as A-(1→6)-D. Similarly, the cross-peaks at δ 4.85/85.09 and δ 3.88/102.84 were assigned to the inter-residues A H-1, C C-4 and B H-6, C C-1, respectively. Thus, the following sequences were established: A-(1→4)-C and B-(6→1)-C, respectively. Using 1D and 2D NMR spectra, the entire assignment of the 1H and 13C chemical shifts of the chemical moieties of CMPB90-1 was achieved and is presented in Table 2.
Table 2 Assignment of 13C-NMR and 1H-NMR chemical shifts of CMPB90-1
Sugar residue |
Chemical shifts (ppm) |
C1/H1 |
C2/H2 |
C3/H3 |
C4/H4 |
C5/H5 |
C6/H6 |
A β-D-Gal (1→ |
105.01/4.85 |
72.62/3.64 |
76.02/3.95 |
71.34/3.54 |
75.82/3.31 |
62.43/3.48 |
B →3,6)-α-D-Glc (1→ |
101.83/5.08 |
76.18/3.75 |
85.24/3.73 |
70.53/3.75 |
76.26/3.57 |
66.35/3.88 |
C →4)-β-D-Man (1→ |
102.84/4.53 |
69.56/3.63 |
70.65/3.82 |
85.09/3.67 |
76.27/3.83 |
66.25/3.81 |
D →6)-α-D-Glc (1→ |
98.31/5.00 |
73.05/3.87 |
75.17/3.45 |
69.52/3.34 |
74.87/3.63 |
69.91/3.98 |
Based on the results of HAPEC-PAD, FT-IR, GC-MS, and 1D and 2D NMR, the backbone of CMPB90-1 was determined to be composed of (1→6)-linked α-D-glucopyranosyl residues and (1→3)-linked β-D-glucopyranosyl residues, which branched at O-6. The branch consisted of (1→6)-linked α-D-glucopyranosyl residues and (1→4)-linked α-L-mannopyranosyl residues and was terminated with (1→)-linked α-D-galacopyranosyl residues. From the aforementioned results, the repeating structural unit of CMPB90-1 is shown in Fig. 4.
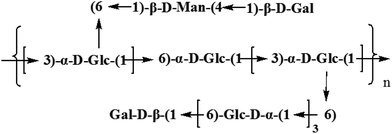 |
| Fig. 4 Predicted structure of CMPB90-1. | |
C. militaris is commonly used as a health food to treat various diseases. Studies have shown that polysaccharides isolated from C. militaris have potential immunostimulating activity and anti-cancer and anti-inflammation effects both in vivo and in vitro.4 In the present study, the action of CMPB90-1 on particular immune activation parameters was evaluated. The mechanism of CMPB90-1 effect on immune regulation was also investigated.
3.4 Effect of CMPB90-1 on splenic lymphocyte proliferation
Immunoregulation is a basic function associated with polysaccharides. The spleen is the most important organ for antibacterial and antifungal immune reactivity, which combines the innate and adaptive immune system in a uniquely organized way. Therefore, the spleen represents an ideal tissue to study immune research.8 To investigate the general activity of CMPB90-1 on immune cells, the immunoregulation activities of CMPB90-1 were evaluated by determining the effect on a mouse spleen lymphocyte proliferation assay. The results showed that CMPB90-1 (31.2–500 μg mL−1) significantly promoted the proliferation of lymphocytes (Fig. 5A). As the spleen contains a large number of T lymphocytes and B lymphocytes, which mediate the body's humoral immunity and cellular immunity,8 CMPB90-1 could significantly increase the activation potential of T- and B-cells and enhance the humoral immunity and cell-mediated immunity. Based on this observation, CMPB90-1 might have immunoregulatory activity to lymphocytes.
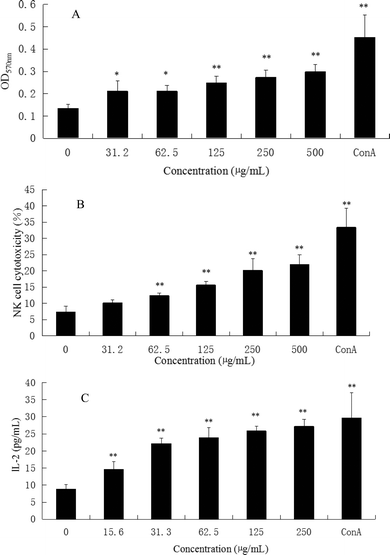 |
| Fig. 5 Effects of CMPB90-1 on splenic lymphocyte proliferation, NK cell cytotoxicity and secretion of IL-2 in the supernatants of lymphocyte cells. (A) Effect of CMPB90-1 on splenic lymphocyte proliferation. The OD570 nm reflected the cell activity of spleen lymphocytes. (B) Effect of CMPB90-1 on NK cell cytotoxicity. (C) Effect of CMPB90-1 on the secretion of IL-2 in the supernatants of lymphocyte cells. Values are expressed as mean ± SD obtained from triplicate experiments (n = 3). Statistical analysis was performed by an independent t-test using the statistical analysis software SPSS 11.5. *P < 0.05, **P < 0.01 versus the negative control. | |
3.5 Effect of CMPB90-1 on NK cell cytotoxicity
NK cells play a major role in the elimination of viruses and tumor cells that have lost self-MHC expression during a transformation process. In addition to the direct effect on viruses and tumors, NK cells can promote the differentiation, activation and recruitment of other immune cells.10 Therefore, we measured the cytotoxic activity of NK cell-sensitive YAC-1 cells. As shown in Fig. 5B, CMPB90-1 enhanced the killing activity of NK cells from splenocytes in vitro (*P < 0.05, **P < 0.01) in a concentration-dependent manner, suggesting that CMPB90-1 enhances nonspecific and specific cytolytic activities against pathogens and tumors.
3.6 Effect of CMPB90-1 on the secretion of IL-2 in the supernatants of lymphocyte cells
IL-2 is essential for the growth, proliferation and differentiation of T cells and is usually produced by T cells during an immune response.32 Thus, the effects of CMPB90-1 on the secretion of IL-2 in supernatants of lymphocyte cells were examined by ELISA assay. As shown in Fig. 5C, CMPB90-1 treatment increased IL-2 supernatant levels in a dose-dependent manner. It demonstrated that CMPB90-1 could enhance the immune function of lymphocyte cells by promoting cytokine levels.
3.7 Effect of CMPB90-1 on T-cell subpopulations
CD3+ is a marker for T lymphocytes, and CD4+ is a marker for T helper lymphocytes. CD4+ T helper lymphocytes activate T lymphocytes and induce cellular immune responses. CD8+ is a marker of T cytotoxic lymphocytes. CD8+ T lymphocytes have cytotoxic effects and suppress the activity of T lymphocytes. The ratio of CD4+/CD8+ thus reflects the activation/inhibitory functions of the immune system.33 T-cell subpopulations after administration of CMPB90-1 are shown in Table 3. The percentage of CD4+ increased in the presence of CMPB90-1 in a dose-dependent manner and the ratio of CD4+/CD8+ T lymphocytes also increased (Table 3). The above results indicated that CMPB90-1 improved the T-lymphocyte immune function partially by upregulating a particular T-cell subpopulation.
Table 3 Effect of CMPB90-1 on T-cell subpopulations (mean ± SD, n = 3)
Group |
Dose (μg mL−1) |
CD3+CD4+ (%) |
CD3+CD8+ (%) |
CD4+/CD8+ |
*P < 0.05 and **P < 0.01 versus the negative control (without CMPB90-1). |
Control |
|
29.27 ± 4.06 |
33.47 ± 4.12 |
0.89 ± 0.25 |
CMPB90-1 |
500 |
44.51 ± 6.44* |
33.66 ± 4.73 |
1.31 ± 0.12* |
|
1000 |
46.57 ± 5.42** |
32.03 ± 6.06 |
1.47 ± 0.33* |
3.8 Effect of CMPB90-1 on the vitality of RAW264.7 cells
To investigate the effect of CMPB90-1 on the viability of RAW.264.7 macrophages, cells were treated with different concentrations of CMPB90-1 (31.3–500 μg mL−1) for 48 h, and then cell viability was determined using the MTT assay. CMPB90-1 at concentrations of 31.3–500 μg mL−1 did not affect cell viability significantly (Fig. 6A) (P > 0.05). This observation demonstrated that CMPB90-1 induces no obvious toxicity in RAW264.7 cells.
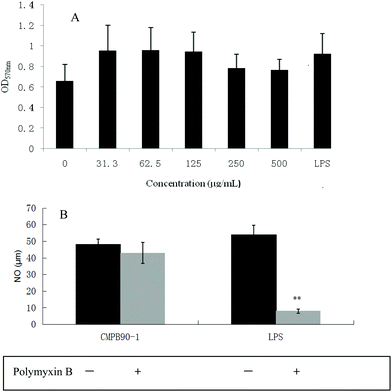 |
| Fig. 6 Effects of CMPB90-1 on vitality of RAW264.7 cells and determination of endotoxin contamination in CMPB90-1. (A) Effect of CMPB90-1 on vitality of RAW264.7 cells. The OD570 nm reflected the cell activity of RAW264.7 cells. (B) Determination of endotoxin contamination in CMPB90-1. Values were expressed as mean ± SD obtained from triplicate experiments (n = 3). Statistical analysis was performed by an independent t-test using the statistical analysis software SPSS 11.5. P > 0.05 versus the negative control. | |
3.9 Effect of CMPB90-1 on the phagocytic activity of macrophages
The phagocytosis of macrophages represents the first and imperative step in an immune response, and a remarkable characteristic of activated macrophages is the observed increase in phagocytosis activity.34 Thus, the phagocytic ability of RAW264.7 cells was measured via the neutral red uptake method (LPS as a positive group). The results showed that CMPB90-1 significantly increased the phagocytosis of RAW 264.7 cells in a dose-dependent manner (Fig. 8A) (*P < 0.05, **P < 0.01) compared with that of the control cells.
To further confirm the effect of CMPB90-1 on the phagocytosis of RAW264.7 cells, the internalization of FITC-dextran by cells was analyzed by flow cytometry, and the phagocytosis was expressed as the mean fluorescence intensity of FITC-dextran in RAW264.7 cells. As shown in Fig. 8B and C, the mean fluorescence intensity increased in a dose-dependent manner when incubated with CMPB90-1 for 24 h. Treatment with the highest dose of CMPB90-1 stimulated significant phagocytosis activity, which was similar to that stimulated by 1.00 μg mL−1 LPS (the positive control). Our results showed that CMPB90-1 observably promoted macrophages to uptake neutral red and fluorescent microspheres in a dose-dependent manner, which illustrated that CMPB90-1 significantly increased the phagocytic activity of macrophages.
3.10 Effect of CMPB90-1 on the expression of surface molecules on RAW264.7 cells
The expression of CD86, CD206 and MHC II on RAW264.7 cells were measured by flow cytometry after treatment with CMPB90-1 for 24 h. We found that treatment of RAW264.7 cells with CMPB90-1 increases M1 phenotype, characterized as increased expression of M1 characteristic surface molecules CD86 and MHC II. At the same time, the expression of CD206 as characteristic surface molecules of M2 macrophages was reduced (Fig. 7). M1 macrophages are considered to mediate host defense to infections and have a more powerful function in removing external invaders versus M2 macrophages.15 Thus, our data indicated that CMPB90-1 improved the function of macrophages by inducing M1 polarization of macrophages, and then evaluated its immunomodulating activities.
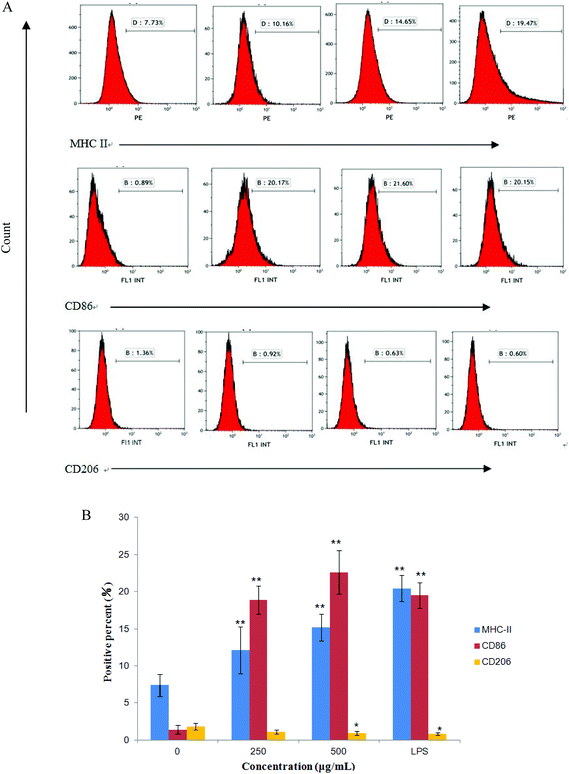 |
| Fig. 7 Effects of CMPB90-1 on expression levels of surface molecules in RAW264.7 cells. (A) Expression of MHC class I, CD86 and CD206 was analyzed by flow cytometry. The percentage in the pane represents the percentage of positive cells. The figure shown is representative of three independent experiments. (B) The histogram demonstrates the positive percentage of cells for various molecules in RAW264.7 cells. The values are presented as mean ± SD (n = 3). *P < 0.05, **P < 0.01 versus negative control. | |
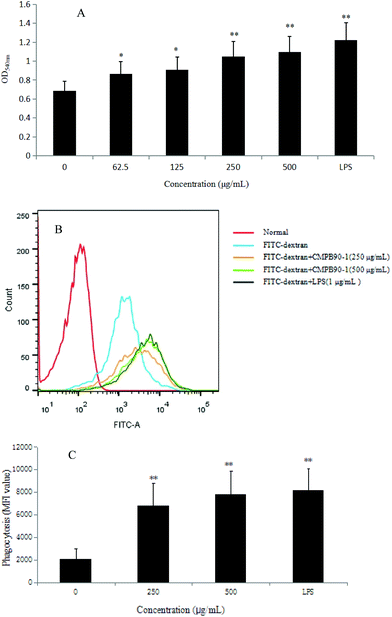 |
| Fig. 8 Effects of CMPB90-1 on the phagocytosis function of macrophages. (A) Effect of CMPB90-1 on macrophage phagocytosis, as determined by the neutral red uptake assay. (B) The extent of phagocytic uptake was determined by flow cytometry. (C) Statistical data of the mean fluorescence intensity (MFI) value determined by flow cytometry. Values are expressed as mean ± SD obtained from triplicate experiments (n = 3). Statistical analysis was performed by an independent t-test using the statistical analysis software SPSS 11.5. *P < 0.05, **P < 0.01 versus the negative control. | |
3.11 Effect of CMPB90-1 on the production of nitric oxide and TNF-α
M1 macrophages (classically activated) exert a pro-inflammatory activity. They have been known to release pro-inflammatory cytokines, such as TNF-α and NO. NO is one of the signaling molecules related to macrophage cytolytic function.14 Our results showed that CMPB90-1 could stimulate NO production. Treatment with a high dose of CMPB90-1 stimulated significant NO production and the level of production was similar to that production stimulated by 1.00 μg mL−1 LPS (Fig. 9A). The results suggested that CMPB90-1 increased the concentration of NO in supernatants, which is crucial for killing microbes, parasites and tumor cells. Besides, the effects of CMPB90-1 on the production of TNF-α in RAW264.7 cells were also determined (Fig. 9B). The secretion level of TNF-α in RAW264.7 cells was close to 1500 pg ml−1 at the dose of 500 μg mL−1. TNF-α is a major pro-inflammatory cytokine produced during inflammation primarily by macrophages. This cytokine plays an important role in regulating immune responsiveness.35 Our results suggested that CMPB90-1 treatment promoted the production of NO and TNF-α in a dose-dependent manner. Thus, CMPB90-1 remarkably induced the secretion of M1 chemokines, which demonstrates that CMPB90-1 could induce M1 polarization of macrophages. These results were consistent with the expression of surface molecules on RAW264.7 cells after CMPB90-1 treatment. M1 macrophages are considered to enhance immune response and mediate host defense against microbial infections and tumors, whereas M2 macrophages are considered to lead to immune tolerance and tumor progression.14 Thus, our research indicated that CMPB90-1 induced M1 polarization of macrophages and then evaluated its defense function and other immunomodulating activities. For some diseases involving macrophage immunodeficiency, such as tumors, CMPB90-1 could potentially be used as a functional supplement food ingredient.
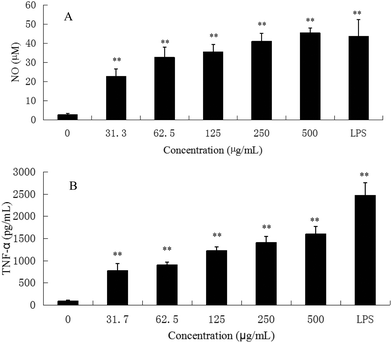 |
| Fig. 9 Effects of CMPB90-1 on nitric oxide and TNF-α production in RAW264.7 macrophages. (A) NO; (B) TNF-α. Values are expressed as mean ± SD obtained from triplicate experiments (n = 3). Statistical analysis was performed by an independent t-test using the statistical analysis software SPSS 11.5. *P < 0.05, **P < 0.01 versus the negative control. | |
To ensure that the effects of CMPB90-1 were not due to endotoxin contamination, NO production was evaluated in RAW264.7 macrophages treated with CMPB90-1 with or without PMB. As shown in Fig. 6B, generation of NO in CMPB90-1-treated macrophages was not affected by the presence of PMB, but LPS-induced NO production was inhibited in the presence of PMB, which indicated that macrophage activation by CMPB90-1 was not due to endotoxin contamination.
3.12 Effect of CMPB90-1 on the expression of NF-κB(65), MAPKs and TLRs
The defensive mechanisms of macrophages can be activated when various stimulus molecules bind to pattern recognition receptors (PRRs), such as Toll-like receptors (TLRs) on the surface of macrophages and trigger several different signaling pathways including MAPKs and NF-κB.36 NF-κB is formed by hetero- or homodimerization of proteins in the Rel family, including p65 and p50. These NF-κB molecules act through distinct signaling pathways that converge on the activation of an IκB-α kinase (IKK); IKK activation initiates IκB-α phosphorylation at specific amino-terminal serine residues, thereby initiating NF-κB phosphorylation, which is then free to translocate to the nucleus and bind to DNA.37 The phosphorylation of NF-κB was detected by western blotting. After incubating RAW264.7 with CMPB90-1 (0, 62.5, 125 and 250 μg ml−1) and LPS (1 μg ml−1) for 24 h, the total protein content was extracted. The level of total NF-κB (p65) remained nearly unchanged, whereas the phosphorylation of NF-κB (p-p65) was significantly enhanced (Fig. 10A) compared to those of the untreated cells (p < 0.01). To confirm the effects of CMPB90-1 on the activation of the NF-κB signaling pathway, the phosphorylation of IκB-α was further examined and protein expression of this protein increased just as we expected. Thus, the results showed that the positive regulation of macrophage activities by CMPB90-1 was probably associated with the IκB-NF-κB signaling pathway. When cells were stimulated by CMPB90-1, NF-κB was translocated into the nucleus, which in turn modulated its downstream gene expression.
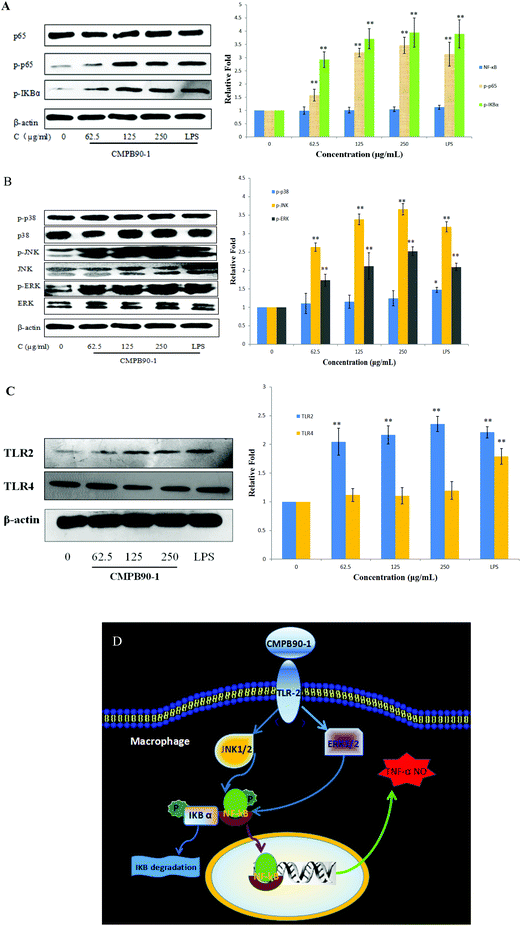 |
| Fig. 10 Effects of CMPB90-1 on expression of NF-κB, MAPKs and TLR2. (A) Effect of CMPB90-1 on the expression of the IκB-NF-κB signaling pathway; (B) effect of CMPB90-1 on the expression of MAPKs; (C) effect of CMPB90-1 on the expression of TLR2 and TLR4; (D) schematic illustrating the potential signaling pathways involved in macrophage activation by CMPB90-1. The figure is representative of three independent experiments. Histogram represents quantification of protein expression levels using Quantity One software (levels of NC group defined as 1). The electrophoresis bands were observed by X-ray film and photos were taken as above, where β-actin was the standard. The values are presented as mean ± SD (n = 3). Significant differences with control cells are designated as *P < 0.05, **P < 0.01. | |
MAPKs play critical roles in the regulation of cell growth and differentiation as well as in cellular responses to cytokines and stresses. MAPKs are also known to be important for the transcriptional activation of NF-κB.38 To investigate whether MAPK signaling pathways were involved in immunostimulatory activity by CMPB90-1, we examined the effect of CMPB90-1 on the phosphorylation of extracellular-signal-regulated kinase (ERK), c-Jun N-terminal kinase (JNK) and p38 in macrophages by western blotting analysis. As shown in Fig. 10B, the protein expression levels of phosphorylated-ERK and phosphorylated-JNK dramatically increased (p < 0.01) in RAW264.7 cells treated by CMPB90-1 over the tested concentration range (levels of NC group defined as 1). During the past years, the mechanisms of immunostimulatory activity have been investigated for polysaccharides. Research has shown that MAPKs are also known to be important for the transcriptional activation of NF-κB. For example, the sulfated polysaccharide SP1 from Caulerpa lentillifera showed the ability to activate macrophages and enhance the production of NO via action on the NF-κB and MAPK signaling pathways.39 In our research, the response of NF-κB nuclear translocation by CMPB90-1 was highly consistent with those of the phosphorylation of ERK and JNK, revealing that the stimulation of the NF-κB signaling pathway by CMPB90-1 is mediated by the activation of MAPK signaling pathways.
In most cases, polysaccharides could not enter cells directly because of their large molecular mass. It has been reported that the first step for polysaccharides to perform their functions is the recognition by pattern recognition receptors (PRRs) of cells.40 TLRs are one kind of important PRR in macrophages. TLR2 and TLR4 are involved in the recognition of a wide range of Pathogen Associated Molecular Patterns derived from bacteria, fungi, parasites and viruses.41 We conducted western blot experiments to examine the expression of TLR2 and TLR4 proteins. As shown in Fig. 10C, CMPB90-1 caused a marked increase in the expression of TLR2, but not in that of TLR4. The results showed that macrophage activities of CMPB90-1 are probably associated with TLR2. Thus, we may conclude that converting macrophages to an M1-like phenotype by CMPB90-1 treatment may be partly related to the activation of TLR2, MAPK and NF-κB pathways. A schematic illustrating the potential signaling pathways involved in macrophage activation by CMPB90-1 is presented in Fig. 10D.
The immunomodulatory activity of polysaccharides is closely associated with their structure, such as monosaccharide composition and glycosidic bonds.42 Previous studies have proven that polysaccharides containing galactose and glucose are most likely recognized by TLR2, and galactose and glucose are always associated with immunomodulatory activity.43 Also, CMPB90-1 possessed galactose and glucose. Therefore, the monosaccharide composition of CMPB90-1 may have contributed to immunomodulatory activity. Besides, the glycosidic bonds in polysaccharides are always associated with immunomodulatory activity. It is reported that polysaccharides that include α-D-glucosidic and β-D-glucosidic linkages exhibit a more notable positive effect on macrophage NO production.44 It can be inferred that the glucosidic linkages of CMPB90-1 may contribute to immunomodulatory activity. As reported by Zhang45et al. reported, polysaccharides from Lepidium meyenii with α-D-Glc and β-D-Gal could induce M1 polarization of original macrophages, which is structurally similar to CMPB90-1, and TLR2 was confirmed as the membrane receptor of MC-2 on macrophages. Thus, the bioactivity of CMPB90-1 to macrophages’ phenotype may depend on the composition of α-D-Glc and β-D-Gal. In summary, the bioactivity of CMPB90-1 is closely associated with its structure.
4. Conclusions
In conclusion, the present study clearly demonstrated that a novel low-molecular-weight polysaccharide (CMPB90-1) obtained by alkaline extraction from the cultured fruiting bodies of C. militaris is a homogeneous polysaccharide that predominantly contains D-glucose, D-mannose and D-galactose. CMPB90-1 showed the immunostimulatory activities in splenic lymphocytes and NK cells. Additionally, CMPB90-1 strengthened the phagocytosis function of macrophages and induced M1 polarization of macrophages, which arose from the activation of TLR2 as well as MAPK and NF-κB pathways. These results indicated that CMPB90-1 could potentially be used as a functional supplement food for hypoimmunity.
Funding
This research work was financially supported by Major National Science and Technology Projects/Significant New Drugs Creation (no. 2011ZX09102-001-33).
Conflicts of interest
The authors declare no competing financial interest
Acknowledgements
The authors thank Dr Dongbo Yu from The University of Chicago Medical Center, USA, for proofreading our manuscript.
References
- L. He, P. Ji, J. Cheng, Y. Wang, H. Qian, W. Li, X. Gong and Z. Wang, Structural characterization and immunostimulatory activity of a novel protein-bound polysaccharide produced by Hirsutella sinensis, Food Chem., 2013, 141, 946–953 CrossRef CAS PubMed.
- S. K. Das, M. Masuda, A. Sakurai and M. Sakakibara, Medicinal uses of the Mushroom Cordyceps militaris: Current state and prospects, Fitoterapia, 2010, 81, 961–968 CrossRef PubMed.
- R. M. Yu, W. Yang, L. Y. Song, C. Y. Yan, Z. Zhang and Y. Zhao, Structural characterization and antioxidant activity of a polysaccharide from the fruiting bodies of cultured Cordyceps militaris, Carbohydr. Polym., 2007, 70, 430–436 CrossRef CAS.
- J. S. Lee and E. K. Hong, Immunostimulating activity of the polysaccharides isolated from Cordyceps militaris, Int. Immunopharmacol., 2011, 11, 1226–1233 CrossRef CAS PubMed.
- S. E. Park, J. G. Kim, Y. W. Lee, H. S. Yoo and C. K. Cho, Antitumor activity of water extracts from Cordyceps militaris in NCI-H460 cell xenografted nude mice, J. Acupunct. Merdian Stud., 2009, 2, 294–300 CrossRef.
- X. Xu, X. Wu, Q. Wang, N. Cai, H. Zhang, Z. Jiang, M. Wan and T. Oda, Immunomodulatory effects of alginate oligosaccharides on murine macrophage RAW264.7 cells and their structure-activity relationships, J. Agric. Food Chem., 2014, 62, 3168–3176 CrossRef CAS PubMed.
- M. Zhang, G. Wang, F. Lai and H. Wu, Structural characterization and immunomodulatory activity of a novel polysaccharide from Lepidium meyenii, J. Agric. Food Chem., 2016, 64, 1921–1931 CrossRef CAS PubMed.
- R. E. Mebius and G. Kraal, Structure and function of the spleen, Nature, 2005, 5, 606–616 CAS.
- T. Mosmann and R. Coffman, TH1 and TH2 cells: Different patterns of lymphokine secretion lead to different functional properties, Annu. Rev. Immunol., 1989, 7, 145–173 CrossRef CAS PubMed.
- E. Vivier, E. Tomasello, M. Baratin, T. Walzer and S. Ugolini, Functions of natural killer cells, Nat. Immunol., 2008, 9, 503–510 CrossRef CAS PubMed.
- Q. Fang, J. F. Wang, X. Q. Zha, S. H. Cui, L. Cao and J. P. Luo, Immunomodulatory activity on macrophage of a purified polysaccharide extracted from Laminaria japonica, Carbohydr. Polym., 2015, 134, 66–73 CrossRef CAS PubMed.
- Q. Yu, S. P. Nie, J. Q. Wang, D. F. Huang, W. J. Li and M. Y. Xie, Toll-like receptor 4 mediates the antitumor host response induced by Ganoderma atrum, polysaccharide, J. Agric. Food Chem., 2015, 63, 517–525 CrossRef CAS PubMed.
- B. Ruffell, N. I. Affara and L. M. Coussens, Differential macrophage programming in the tumor microenvironment, Trends Immunol., 2012, 33, 119–126 CrossRef CAS PubMed.
- H. L. Zhang, M. Y. Hassan, X. Y. Zheng, S. Azimullah, H. C. Quezada, N. Amir, M. Elwasila, E. Mix, A. Adem and J. Zhu, Attenuated EAN in TNF-α deficient mice is associated with an altered balance of M1/M2 macrophages, PLoS One, 2012, 7, e38157 CAS.
- C. D. Mills, Anatomy of a discovery: m1 and m2 macrophages, Front. Immunol., 2015, 6, 212 Search PubMed.
- S. Batbayar, D. H. Lee and H. W. Kim, Immunomodulation of fungal β-glucan in host defense signaling by dectin-1, Biomol. Ther., 2012, 20, 433–445 CrossRef CAS PubMed.
- A. P. Vos, L. M'Rabet, B. Stahl, G. Boehm and J. Garssen, Immune-modulatory effects and potential working mechanisms of orally applied nondigestible carbohydrates, Crit. Rev. Immunol., 2007, 27, 97–140 CrossRef CAS PubMed.
- Y. S. Jing, X. L. Cui, Z. Y. Chen, L. J. Huang, L. Y. Song, T. Liu, W. J. Lv and R. M. Yu, Elucidation and biological activities of a new polysaccharide from cultured Cordyceps militaris, Carbohydr. Polym., 2014, 102, 288–296 CrossRef CAS PubMed.
- B. Yang, K. N. Prasad, H. H. Xie, S. Lin and Y. M. Jiang, Structural characteristics of oligosaccharides from soy sauce lees and their potential prebiotic effect on lactic acid bacteria, Food Chem., 2011, 126, 590–594 CrossRef CAS.
- M. Dubois, K. A. Gilles, J. K. Hamilton, P. A. Rebers and F. Smith, Colorimetric method for determination of sugars and related substances, Anal. Chem., 1956, 28, 350–356 CrossRef CAS.
- D. H. Luo, Identification of structure and antioxidant activity of a fraction of polysaccharide purified from Dioscorea nipponica Makino, Carbohydr. Polym., 2008, 71, 544–549 CrossRef CAS.
- X. Q. Hu, J. L. Wang, Y. S. Jing, L. Y. Song, J. H. Zhu, X. L. Cui and R. M. Yu, Structural elucidation and in vitro antioxidant activities of a new heteropolysaccharide from Litchi chinensis, Drug Discoveries Ther., 2015, 9, 116–122 CrossRef PubMed.
- S. Hakomori, A rapid premethylation of glycolipid, and polysaccharide catalyzed by methylsulfinyl carbanion in dimethyl sulfoxide, J. Biochem., 1964, 55, 205–208 CAS.
- P. C. Giraldo, J. B. Carvalho, R. L. Amaral, S. Goncalves, J. J. Eleuterio and F. Guimaraes, Identification of immune cells by flow cytometry in vaginal lavages from women with vulvovaginitis and normal microflora, Amer, J. Reprod. Immunol., 2012, 67, 198–205 CrossRef CAS PubMed.
- Z. Dai, D. Su, Y. Zhang, Y. Sun, B. Hu, H. Ye, S. Jabbar and X. Zeng, Immunomodulatory activity in vitro and in vivo of verbascose from mung beans (Phaseolus aureus), J. Agric. Food Chem., 2014, 62, 10727–10735 CrossRef CAS PubMed.
- J. H. Xie, X. Liu, M. Y. Shen, S. P. Nie, H. Zhang, C. Li, D. M. Gong and M. Y. Xie, Purification, physicochemical characterisation and anticancer activity of a polysaccharide from Cyclocarya paliurus leaves, Food Chem., 2013, 136, 1453–1460 CrossRef CAS PubMed.
- E. K. Mandal, K. Maity, S. K. Gantait, B. Behera and T. K. Maiti, Chemical analysis of an immunostimulating (1-4)-, (1-6)-branched glucan from an edible mushroom Calocybe indica, Carbohydr. Res., 2012, 347, 172–177 CrossRef CAS PubMed.
- Y. G. Niu, H. Y. Wang, Z. H. Xie, M. Whent, X. D. Gao and X. Zhang, Structural analysis and bioactivity of a polysaccharide from the roots of Astragalus membranaceus (Fisch) Bge. var. mongolicus (Bge.) Hsiao, Food Chem., 2011, 128, 620–626 CrossRef CAS.
- J. S. Lee, J. S. Kwon, J. W. Pahk, W. C. Shin and S. Y. Lee, Structural characterization of immunostimulating polysaccharide from cultured mycelia of Cordyceps militaris, Carbohydr. Polym., 2010, 80, 1011–1017 CrossRef CAS.
- G. L. Song and Q. Z. Du, Structure characterization and antitumor activity of an α, β-glucan polysaccharide from Auricularia polytricha, Food Res. Int., 2012, 45, 381–387 CrossRef CAS.
- H. T. Bi, T. T. Gao, Z. H. Li, L. Ji, W. Yang, B. J. Iteku, E. X. Liu and Y. F. Zhou, Structural elucidation and antioxidant activity of a water-soluble polysaccharide from the fruit bodies of Bulgaria inquinans, (Fries), Food Chem., 2013, 138, 1470–1475 CrossRef CAS PubMed.
- X. Xie, G. Zou and C. Li, Antitumor and immunomodulatory activities of a water-soluble polysaccharide from Chaenomeles speciosa, Carbohydr. Polym., 2015, 132, 323–329 CrossRef CAS PubMed.
- Y. Z. Zhu, C. W. Hu, X. W. Li, B. Shao, H. Sun, H. S. Zhao and Y. F. Li, Suppressive effects of aluminum trichloride on the T lymphocyte immune function of rats, Food Chem. Toxicol., 2012, 50, 532–535 CrossRef CAS PubMed.
- A. Cheng, F. Wan, J. Wang, Z. Jin and X. Xu, Macrophage immunomodulatory activity of polysaccharides isolated from Glycyrrhiza uralensis Fish, Int. Immunopharmacol., 2008, 8, 43–50 CrossRef CAS PubMed.
- Y. Chen, H. Zhang, Y. Wang, S. Nie, C. Li and M. Xie, Sulfated modification of the polysaccharides from Ganoderma atrum and their antioxidant and immunomodulating activities, Food Chem., 2015, 186, 231–238 CrossRef CAS PubMed.
- F. Sheu, P. J. Chien, K. Y. Hsieh, K. L. Chin, W. T. Huang, C. Y. Tsao, Y. F. Chen, H. C. Cheng and H. H. Chang, Purification, cloning, and functional characterization of a novel immunomodulatory protein from Antrodia camphorata, (bitter mushroom) that exhibits TLR2-dependent NF-Kb activation and M1 polarization within murine macrophages, J. Agric. Food Chem., 2009, 57, 4130–4141 CrossRef CAS PubMed.
- M. H. Pan, S. Y. Lin-Shiau, C. T. Ho, J. H. Lin and J. K. Lin, Suppression of lipopolysaccharide-induced nuclear factor-κB activity by theaflavin-3,3′-digallate from black tea and other polyphenols through down-regulation of IκB kinase activity in macrophages, Biochem. Pharmacol., 2000, 59, 357–367 CrossRef CAS PubMed.
- L. Vermeulen, G. D. Wilde, P. V. Damme, W. V. Berghe and G. Haegeman, Transcriptional activation of the NF-κB p65 subunit by mitogen- and stress-activated protein kinase-1 (MSK1), EMBO J., 2003, 22, 1313–1324 CrossRef CAS PubMed.
- R. Maeda, T. Ida, H. Ihara and T. Sakamoto, Immunostimulatory activity of polysaccharides isolated from Caulerpa lentillifera on macrophage cells, Biosci., Biotechnol., Biochem., 2012, 76, 501–505 CrossRef CAS PubMed.
- S. Z. Xie, R. Hao, X. Q. Zha, L. H. Pan, J. Liu and J. P. Luo, Polysaccharide of Dendrobium huoshanense, activates macrophage via toll-like receptor 4-mediated signaling pathways, Carbohydr. Polym., 2016, 146, 292–300 CrossRef CAS PubMed.
- S. Akira, S. Uematsu and O. Takeuchi, Pathogen recognition and innate immunity, Cell, 2006, 124, 783–801 CrossRef CAS PubMed.
- S. S. Ferreira, C. P. Passos, P. Madureira, M. Vilanova and M. A. Coimbra, Structure-function relationships of immunostimulatory polysaccharides: A review, Carbohydr. Polym., 2015, 132, 378–396 CrossRef CAS PubMed.
- R. T. Figueiredo, V. C. B. Bittencourt, L. C. L. Lopes, G. Sassaki and E. Barreto-Bergter, Toll-like receptors (TLR2 and TLR4) recognize polysaccharides of Pseudallescheria boydii cell wall, Carbohydr. Res., 2012, 356, 260–264 CrossRef CAS PubMed.
- D. T. Wu, J. Xie, L. Y. Wang, Y. J. Ju, G. P. Lv, F. Leong, J. Zhao and S. P. Li, Characterization of bioactive polysaccharides from Cordyceps militaris produced in China using saccharide mapping, J. Funct. Foods, 2014, 9, 315–323 CrossRef CAS.
- M. Zhang, W. Wu, Y. Ren, X. F. Li, Y. Tang and T. Min,
et al., Structural characterization of a novel polysaccharide from lepidium meyenii (maca) and analysis of its regulatory function in macrophage polarization in vitro, J. Agric. Food Chem., 2017, 65, 1146–1157 CrossRef CAS PubMed.
Footnote |
† These authors contributed equally to this work. |
|
This journal is © The Royal Society of Chemistry 2018 |