A fast-response two-photon fluorescent probe for the detection of Cys over GSH/Hcy with a large turn-on signal and its application in living tissues†
Received
7th October 2016
, Accepted 14th November 2016
First published on 15th November 2016
Abstract
Cysteine (Cys), a small-molecule aminothiol, plays important roles in various physiological processes in connection with various diseases, such as skin lesions, edema, hair depigmentation and liver damage. We developed a novel two-photon fluorescent probe for sensing Cys in presence of GSH and Hcy in vivo. The two-photon fluorescent probe exhibited favorable properties, including fast response (about 20 min), good selectivity, and low cytotoxicity. Furthermore, it was successfully applied for imaging Cys in living cells and tissues.
Introduction
Cysteine (Cys), a small-molecule aminothiol, plays important roles in various physiological processes in connection with various diseases, such as skin lesions, edema, hair depigmentation and liver damage.1 Cys is structurally similar to homocysteine (Hcy) and glutathione (GSH); thus, it is very challenging to detect Cys in the presence of Hcy and GSH. Therefore, it is worthwhile to construct efficient and functional methods for selective and sensitive detection of cysteine (Cys) over other amino acids, particularly Hcy and GSH, under physiological conditions.
Some existing analytical methods for the detection of Cys, such as high-performance liquid chromatography (HPLC), electrochemical assay, capillary electrophoresis, UV-Vis spectroscopy, FT-IR spectroscopy, mass spectrometry, gas chromatography, and immunoassay, have been exploited in previous reports.2–9 However, sophisticated instrumentation and highly customized operating techniques are required during these processes, which are complex and time-consuming, i.e., they are not fit for practical application in biosystems. During the last few years, organic fluorescent probes, which are regarded as powerful monitoring tools, have become important in biological studies due to their high selectivity, high sensitivity and real-time detection.10–18
Recently, a number of fluorescent probes for monitoring Cys over Hcy and GSH in living biosystems have been reported.19–45 Most of these probes were excited by one photon at short excitation wavelengths leading to photobleaching of fluorescent dyes and damage to living cells and tissues. Moreover, there are significant merits of two-photon microscopy (TPM) with long excitation wavelengths such as three-dimensional detection of living tissues, suppression of photo-damage to biological samples, increased penetration ability into tissue and reduced fluorescent interference of background signals. Furthermore, there are few examples for the detection of Cys over Hcy and GSH under two-photon excitation. Therefore, it is very important and necessary to construct a two-photon fluorescent probe, which could be suitable for imaging Cys specifically in vivo.
Ideal fluorescent sensors with fast response, good selectivity and two-photon imaging that can specifically monitor Cys over Hcy and GSH are rare. As Cys plays an important role in biosystems, it is necessary to design and synthesize Cys two-photon fluorescent probes with fast response and good selectivity. The following challenges must be overcome to construct such a probe: (1) identification of a suitable two-photon fluorescent platform and (2) selectivity for Cys over other amino acids and ions, particularly Hcy and GSH.
In this report, we have constructed a novel two-photon fluorescent probe NP-Cys for imaging Cys over Hcy and GSH (Scheme 1). This novel turn-on fluorescent probe was designed for the recognition of Cys in vivo with a fast response and good selectivity over other amino acids and analytes, and was suitable for revealing the role of Cys in various physiological processes (Scheme 2).
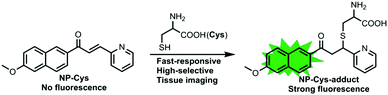 |
| Scheme 1 The structure of NP-Cys and the proposed sensing mechanism for Cys. | |
 |
| Scheme 2 Synthesis of the two-photon fluorescent probe NP-Cys. | |
Results and discussion
Synthesis of probe NP-Cys
Compound 1 and 2 were obtained from commercial sources and used without further purification. The two-photon probe NP-Cys was easily synthesized in one step from compound 1 and 2. Complete characterization of NP-Cys by 1H NMR, 13C NMR and HRMS is detailed in the ESI.†
Sensing properties of NP-Cys probe for Cys
With the two-photon probe NP-Cys in hand, its spectral properties were examined. NP-Cys showed almost no fluorescence under 360 nm excitation (Fig. S1, ESI†). In the presence of Cys, NP-Cys exhibited strong emission at 456 nm excitation in PBS–MeOH (v/v = 19/1, pH = 7.4) within 20 min at ambient temperature. With the time extended to 60 minutes, the fluorescence intensity was almost unchanged (Fig. 1), i.e., PBS–MeOH (v/v = 19/1, pH = 7.4) was regarded as a suitable solvent for fluorescence experiments. In addition, the probe NP-Cys was also stable under irradiation, as depicted in Fig. S2 (ESI†).
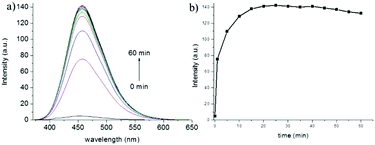 |
| Fig. 1 Reaction-time profiles of NP-Cys (10 μM) in the absence and presence of Cys. | |
To our delight, a large turn-on fluorescence enhancement (about 30-fold) was observed upon addition of up to 30 equiv. of Cys under 456 nm excitation (Fig. 2), which is better than some of the previously reported fluorescent probes. The two-photon fluorescent sensing spectrum of the probe was also obtained (Fig. S3, ESI†). This two-photon probe can be applied to image Cys due to its fast response, good selectivity and large turn-on signal. To get insight into the proposed sensing process, we studied the reaction of NP-Cys with Cys by mass spectrometry. When the probe NP-Cys (20 μM) was treated with Cys (400 μM) in pH 7.4 PBS/MeOH (v/v = 19/1) at room temperature, a significant peak at m/z 411.1378 corresponding to the product NP-Cys-adduct appeared in the ESI-MS spectrum (Fig. S4, ESI†). This result was in good agreement with the proposed sensing mechanism (Scheme 1). Furthermore, the result was also in accordance with previous reports.47
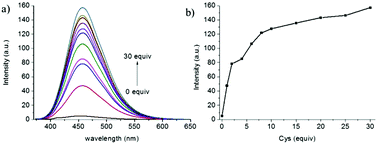 |
| Fig. 2 The fluorescence spectra of NP-Cys (10 μM) in pH 7.4 PBS buffer (containing 5% MeOH) in the absence and presence of Cys (0–30 equiv.). | |
Effect of pH
The pH value of PBS buffer is a factor that may have great effect on fluorescence response. The probe NP-Cys was treated with PBS buffer solutions of distinct pH, as depicted in the Fig. 3. The fluorescence intensity was almost unchanged in the absence of Cys at both acidic and basic pH. The data showed that NP-Cys was immobile over a wide pH range of 1.0 to 10.0. With the addition of Cys, the fluorescence intensity increased gradually until it reached a plateau at a pH range of 6.0 to 10.0, i.e., the probe NP-Cys can image Cys in PBS–MeOH (v/v = 19/1, pH = 7.4) after 20 min at physiological pH (7.4) with 30-fold fluorescence enhancement, showing that the two-photon probe may be applied to biological systems.
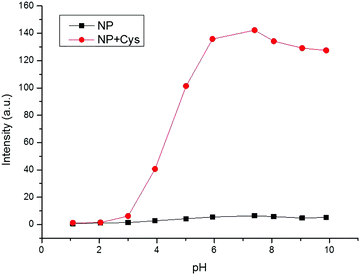 |
| Fig. 3 pH effects on the fluorescence spectrum of NP-Cys (10 μM) in pH 7.4 PBS buffer (containing 5% MeOH) in the absence (■) and presence ( ) of Cys. | |
Selectivity of the NP-Cys probe
Selectivity testing was performed for the free probe NP-Cys using various amino acids, including Cys, Hcy, GSH, Ala, Asp, Glu, Gly, His, Ile, Phe, Ser, Thr, Trp, Tyr and Val. NP-Cys showed a strong fluorescent response to Cys over the other amino acids, particularly Hcy and GSH (Fig. 4a). When other analytes, such as F−, Br−, HPO42−, I−, N2H4, NO3−, OAc−, PO43−, and SO42−, were treated with NP-Cys the fluorescence intensity was almost unchanged (Fig. 4b). In particular, reactive sulfur species such as S2− and SO32− had no influence on the fluorescence response of NP-Cys. In addition, when we increased the concentration of GSH (up to 600 μM), the fluorescence intensity was still much weaker than that when the probe was treated with Cys (100 μM, Fig. S5, ESI†). Therefore, GSH had little effect on the detection of Cys. These results indicated that the probe was suitable to detect Cys with high selectivity over other species.
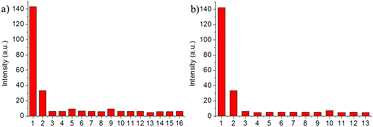 |
| Fig. 4 Fluorescence intensity response of NP-Cys (10 μM) in pH 7.4 PBS buffer (containing 5% MeOH) to various relevant species (100 μM): (a) 1: Cys; 2: GSH; 3: Hcy; 4: Ala; 5: Asp; 6: Glu; 7: Gly; 8: His; 9: Ile; 10: Phe; 11: Ser; 12: Thr; 13: Trp; 14: Tyr; 15: Val; 16: none; (b) 1: Cys; 2: GSH; 3: Hcy; 4: Al3+; 5: Ca2+; 6: Co2+; 7: Cu+; 8: Cu2+; 9: Mg2+; 10: S2−; 11: SO32−; 12: Ni2+; 13: Zn2+. | |
Cytotoxicity and fluorescence imaging with NP-Cys
The above analyses led us to test the detection of Cys in living cells. We believed that the probe NP-Cys would provide high sensitivity, selectivity and resolution. In the beginning, MTT assays were carried out for the probe NP-Cys in different concentrations. The living cells grew very well after treatment with NP-Cys (10.0 μM), i.e., the two-photon probe exhibited low cytotoxicity to the living cells after 24 h (Fig. S6, ESI†). Next, fluorescence imaging experiments were conducted for the detection of Cys in living cells. When HeLa cells were incubated with NP-Cys (10.0 μM) for 0.5 h at 37 °C, evident fluorescence was observed in green channels (Fig. 5b). On the contrary, when the HeLa cells were pre-treated with N-ethylmaleimide (NEM) for 1 h and then incubated with NP-Cys (10.0 μM) for 0.5 h, there was almost no fluorescence in the green channels (Fig. 5e). When the HeLa cells were pre-treated with Cys (600 μM) for 0.5 h prior to treatment with NP-Cys for another 0.5 h, stronger fluorescence intensity was obtained (Fig. 5h). The two-photon excitation fluorescence spectrum was recorded for the HeLa cells after incubation with the probe and excitation at 800 nm (Fig. S7, ESI†). The spectrum was consistent with the results at 360 nm described above, with a strong emission at around 460 nm. These results demonstrated that the probe NP-Cys can be applied to monitor Cys in vivo.
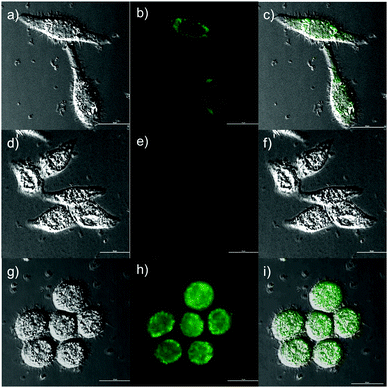 |
| Fig. 5 Fluorescence response of the NP-Cys probe (10.0 μM) to Cys in living HeLa cells. (a) Bright-field image of live HeLa cells incubated with probe NP-Cys (10.0 μM) for 30 min; (b) fluorescence image of (a); (c) overlay of brightfield and green channel. (d) Bright-field image of live HeLa cells pre-treated with NEM (1 mm) for 60 min, and then incubated with NS-S probe (10.0 μM) for 30 min; (e) fluorescence image of (d); (f) overlay of brightfield and green channel. (g) Bright-field image of live HeLa cells pre-treated with Cys (600 μM) for 30 min, and then incubated with NS-S probe (10.0 μM) for 30 min; (h) fluorescence image of (g); (i) overlay of bright-field and green channel. Excitation at 488 nm. Scale bar = 20 μm. | |
Two-photon fluorescence imaging in living tissues with TPM
The previous examination showed that endogenous Cys in living cells could be detected by NP-Cys. With these results and the merits of TPM in hand, we conducted experiments for imaging Cys in living mouse tissues. The thickness of the living tissue slices of mouse liver was about 400 μm. The tissue slices thus prepared were pre-treated with the probe NP-Cys (10.0 μM) for 0.5 h at 37 °C in PBS buffer and then scanned by TPM. Significant fluorescence was observed from 5 to 70 μm depth of the living liver tissues; slight fluorescence also appeared at 80 μm (Fig. 6). These excellent two-photon fluorescence properties suggested that the probe can be applied to monitor Cys in living tissues.
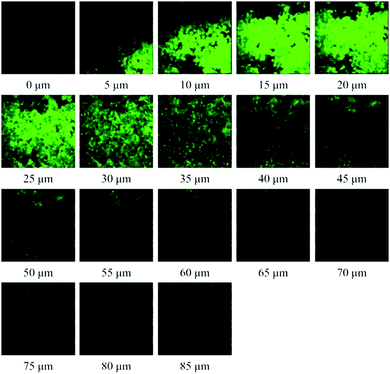 |
| Fig. 6 Two-photon fluorescence images of a fresh mouse liver slice pre-treated with NP-Cys (10 μM) for 30 min in PBS buffer at approximate depths of 0–85 μm. Excitation at 800 nm with fs pulse. | |
Experimental
Materials and instruments
Unless otherwise stated, all the solvents, reagents and materials were obtained from commercial sources and used without other purification. Twice-distilled water was applied to all measurements and experiments. High-resolution electrospray mass spectra (HRMS) were recorded by a Bruker APEX IV-FTMS 7.0T mass spectrometer. NMR spectra were obtained using an AVANCE III 400 MHz Digital NMR Spectrometer with TMS as an internal standard. Electronic absorption spectra were recorded on a LabTech UV Power spectrometer. Fluorescence spectra were obtained with a HITACHI F4600 fluorescence spectrophotometer. The fluorescence images of cells and tissues were collected with Nikon A1MP confocal microscopy with a CCD camera. The pH measurements were taken on a Mettler-Toledo Delta 320 pH meter. Analyses by silica gel plates and column chromatography were carried out over silica gel (mesh 200–300). Both TLC and silica gel were purchased from Qingdao Ocean Chemicals.
Preparation of stock and test solutions
All the experiments were conducted according to the following steps. A stock solution of the probe NP-Cys (10 mM) was prepared in DMF (HPLC grade). The stock solutions of all other analytes were prepared by dissolution in distilled water (10 mL) to afford 10 mM aqueous solutions. The pH of the PBS buffer solution was varied by addition of different volumes of NaOH (0.1 M) or HCl (0.2 M). The stock solutions were freshly prepared and diluted to the desired concentrations by distilled water when needed. Test procedures were implemented according to our previous report.46
Cytotoxicity assays
The living cells line was treated in DMEM (Dulbecco's Modified Eagle Medium) supplied with fetal bovine serum (10%, FBS), penicillin (100 U mL−1) and streptomycin (100 μg mL−1) under an atmosphere of CO2 (5%) and air (95%) at 37 °C. The HeLa cells were then seeded into 96-well plates, and 0, 1, 5, 10, 15, and 20 μM (final concentration) of the probe NP-Cys (99.9% DMEM and 0.1% DMSO) was added. The cells were subsequently cultured at 37 °C in an atmosphere of CO2 (5%) and air (95%) for 24 hours. Then, the HeLa cells were washed with PBS buffer, and DMEM medium (500 μL) was added. Next, MTT (50 μL, 5 mg mL−1) was injected to every well, and the wells were incubated for 4 h. Violet formazan was treated with sodium dodecyl sulfate solution (500 μL) in the H2O–DMF mixture. Absorbance of the solution was measured at 570 nm using a microplate reader. The cell viability was determined by assuming 100% cell viability for cells without NP-Cys.
Cell culture and fluorescence imaging
The living HeLa cells were grown in modified Eagle's medium (MEM) supplied with FBS (10%) under CO2 atmosphere (5%) and air (95%) at 37 °C for 24 h. Before imaging, the HeLa cells were washed with PBS three times and then incubated with NP-Cys (10 μM) for 0.5 h in an atmosphere of CO2 (5%) and air (95%) at 37 °C. For control experiment 1, the cells were pre-incubated with N-ethylmaleimide (1 mM, NEM) for 0.5 h, and then treated with NP-Cys (10 μM) under an atmosphere of CO2 (5%) and air (95%) at 37 °C. For control experiment 2, the cells were pre-treated with Cys (600 μM) for 0.5 h and then incubated with NP-Cys (10 μM) for 0.5 h under an atmosphere of CO2 (5%) and air (95%) at 37 °C. After washing by PBS buffer three times, the ideal fluorescence images were obtained by a Nikon A1MP confocal microscope equipped with a CCD camera.
Preparation of fresh mouse liver slices and two-photon fluorescence imaging
The fresh mouse liver slices were obtained from a 14 day-old mouse. The living liver slices were prepared in 400 micron thickness using a vibrating-blade microtome in 25 mM PBS (pH 7.4). The living liver slices were pre-treated with NP-Cys (10 μM) for 0.5 h. Following incubation for 1 h at 37 °C, the slices were washed three times by PBS buffer and imaged. The two-photon fluorescence emission was collected at between 470 and 570 nm upon excitation at 800 nm with a femtosecond laser.
Synthesis of the NP-Cys probe
A mixture of compound 1 (0.5 mmol, 100.0 mg, 1.0 equiv.) and 2 (0.55 mmol, 58.9 mg, 1.1 equiv.) was dissolved in EtOH (5.0 mL). Piperidine (0.55 mmol, 46.8 mg, 1.1 equiv.) was added under N2. The reaction was refluxed for 8 h. Then, the reaction mixture was diluted with distilled water (2.0 mL) and extracted with dichloromethane. The organic layer was washed with saturated sodium chloride, dried over MgSO4, filtered, and concentrated under vacuum. The pure product NP-Cys was obtained by silica column chromatography with a yield of 81%. 1H-NMR (400 MHz, CDCl3): δ 8.74 (d, J = 3.6 Hz, 1H), 8.66 (s, 1H), 8.41 (d, J = 15.2 Hz, 1H), 8.16 (dd, J = 8.4, 2.8 Hz, 1H), 7.92 (d, J = 9.2 Hz, 1H), 7.82–7.80 (m, 3H), 7.54 (d, J = 8.0 Hz, 1H), 7.38–7.35 (m, 1H), 7.22 (dd, J = 8.8, 2.4 Hz, 1H), 7.18 (d, J = 2.4 Hz, 1H), 3.96 (s, 3H). 13C NMR (101 MHz, CDCl3): δ 189.8, 160.0, 153.4, 150.2, 142.3, 137.5, 137.1, 133.3, 131.3, 130.6, 128.0, 127.4, 125.7, 125.5, 125.3, 124.5, 119.8, 105.9, 55.5. HRMS (ESI): m/z calcd for C19H16NO2+ (M + H)+, 290.1176; found, 290.1178.
Conclusions
In summary, a novel turn-on two-photon fluorescent probe was constructed for monitoring Cys over GSH and Hcy in vivo. Ideal properties emerged, including good selectivity, high sensitivity (about 20 min) and low cytotoxicity. Furthermore, the probe NP-Cys was applied to image Cys in living cells and tissues in vivo. The probe NP-Cys could have great effect on the development of impactful sensors for other analytes, and could be used for investigation of biological and pathological functions of Cys in living organisms.
Acknowledgements
This study was financially supported by NSFC (21472067, 21672083), Taishan Scholar Foundation (TS201511041), Natural Science Foundation of Shandong Province (ZR2015PA014) and the startup fund of University of Jinan (309-10004, 160082103).
Notes and references
- S. Shahrokhian, Anal. Chem., 2001, 73, 5972–5978 CrossRef CAS PubMed.
- P. R. Lima, W. J. R. Santos, R. C. S. Luz, F. S. Damos, A. B. Oliveira, M. O. F. Goulart and L. T. Kubota, J. Electroanal. Chem., 2008, 612, 87–96 CrossRef CAS.
- Y. Ogasawara, Y. Mukai, T. Togawa, T. Suzuki, S. Tanabe and K. Ishii, J. Chromatogr. B: Anal. Technol. Biomed. Life Sci., 2007, 845, 157–163 CrossRef CAS PubMed.
- M. Rafii, R. Elango, G. Courtney-Martin, J. D. House, L. Fisher and P. B. Pencharz, Anal. Biochem., 2007, 371, 71–81 CrossRef CAS PubMed.
- W. Wang, O. Rusin, X. Xu, K. K. Kim, J. O. Escobedo, S. O. Fakayode, K. A. Fletcher, M. Lowry, C. M. Schowalter, C. M. Lawrence, F. R. Fronczek, I. M. Warner and R. M. Strongin, J. Am. Chem. Soc., 2005, 127, 15949–15958 CrossRef CAS PubMed.
- W. Wang, J. O. Escobedo, C. M. Lawrence and R. M. Strongin, J. Am. Chem. Soc., 2004, 126, 3400–3401 CrossRef CAS PubMed.
- Y. Sato, T. Iwata, S. Tokutomi and H. Kandori, J. Am. Chem. Soc., 2005, 127, 1088–1089 CrossRef CAS PubMed.
- G. Chen, L. Zhang and J. Wang, Talanta, 2004, 64, 1018–1023 CrossRef CAS PubMed.
- H. Refsum, A. D. Smith, P. M. Ueland, E. Nexo, R. Clarke, J. McPartlin, C. Johnston, F. Engbaek, J. Schneede, C. McPartlin and J. M. Scott, Clin. Chem., 2004, 50, 3–32 CAS.
- H. Chen, Y. Tang and W. Lin, TRAC, Trends Anal. Chem., 2016, 76, 166–181 CrossRef CAS.
- V. S. Lin, W. Chen, M. Xian and C. J. Chang, Chem. Soc. Rev., 2015, 44, 4596–4618 RSC.
- Y. Tang, D. Lee, J. Wang, G. Li, J. Yu, W. Lin and J. Yoon, Chem. Soc. Rev., 2015, 44, 5003–5015 RSC.
- X. Zhou, S. Lee, Z. Xu and J. Yoon, Chem. Rev., 2015, 115, 7944–8000 CrossRef CAS PubMed.
- X. Li, X. Gao, W. Shi and H. Ma, Chem. Rev., 2014, 114, 590–659 CrossRef CAS PubMed.
- H. Li, J. Fan and X. Peng, Chem. Soc. Rev., 2013, 42, 7943–7962 RSC.
- L. Yuan, W. Lin, K. Zheng, L. He and W. Huang, Chem. Soc. Rev., 2013, 42, 622–661 RSC.
- Y. Yang, Q. Zhao, W. Feng and F. Li, Chem. Rev., 2013, 113, 192–270 CrossRef CAS PubMed.
- J. Du, M. Hu, J. Fan and X. Peng, Chem. Soc. Rev., 2012, 41, 4511–4535 RSC.
- H. Li, J. Fan, J. Wang, M. Tian, J. Du, S. Sun, P. Sun and X. Peng, Chem. Commun., 2009, 5904–5906 RSC.
- L.-Y. Niu, Y.-S. Guan, Y.-Z. Chen, L.-Z. Wu, C.-H. Tung and Q.-Z. Yang, J.
Am. Chem. Soc., 2012, 134, 18928–18931 CrossRef CAS PubMed.
- H. S. Jung, T. Pradhan, J. H. Han, K. J. Heo, J. H. Lee, C. Kang and J. S. Kim, Biomaterials, 2012, 1–8 CAS.
- H. Wang, G. Zhou, H. Gai and X. Chen, Chem. Commun., 2012, 48, 8341–8343 RSC.
- Z. Guo, S. Nam, S. Park and J. Yoon, Chem. Sci., 2012, 3, 2760–2765 RSC.
- Z. Yang, N. Zhao, Y. Sun, F. Miao, Y. Liu, X. Liu, Y. Zhang, W. Ai, G. Song, X. Shen, X. Yu, J. Sun and W. Y. Wong, Chem. Commun., 2012, 48, 3442–3444 RSC.
- K.-H. Hong, S.-Y. Lim, M.-Y. Yun, J.-W. Lim, J.-H. Woo, H. Kwon and H.-J. Kim, Tetrahedron Lett., 2013, 3003–3006 CrossRef CAS.
- C. Zhao, X. Li and F. Wang, Chem. – Asian J., 2014, 9, 1777–1781 CrossRef CAS PubMed.
- X. Dai, Q.-H. Wu, P.-C. Wang, J. Tian, Y. Xu, S.-Q. Wang, J.-Y. Miao and B.-X. Zhao, Biosens. Bioelectron., 2014, 59, 35–39 CrossRef CAS PubMed.
- Y. Liu, S. Zhang, X. Lv, Y.-Q. Sun, J. Liu and W. Guo, Analyst, 2014, 139, 4081–4087 RSC.
- J. Liu, Y.-Q. Sun, Y. Huo, H. Zhang, L. Wang, P. Zhang, D. Song, Y. Shi and W. Guo, J. Am. Chem. Soc., 2014, 136, 574–577 CrossRef CAS PubMed.
- M. Zheng, H. Huang, M. Zhou, Y. Wang, Y. Zhang, D. Ye and H.-Y. Chen, Chem. – Eur. J., 2015, 21, 1–8 CrossRef.
- M. Zhang, M. Yu, F. Li, M. Zhu, M. Li, Y. Gao, L. Li, Z. Liu, J. Zhang, D. Zhang, T. Yi and C. Huang, J. Am. Chem. Soc., 2007, 129, 10322–10323 CrossRef CAS PubMed.
- Y. H. Lee, W. X. Ren, J. Han, K. Sunwoo, J.-Y. Lim, J.-H. Kim and J. S. Kim, Chem. Commun., 2015, 51, 14401–14404 RSC.
- X. He, X. Wu, W. Shi and H. Ma, Chem. Commun., 2016, 52, 9410–9413 RSC.
- S. Areti, S. K. Verma, J. Bellare and C. P. Rao, Anal. Chem., 2016, 88, 7259–7267 CrossRef CAS PubMed.
- F. Kong, B. Hu, Y. Gao, K. Xu, X. Pan, F. Huang, Q. Zheng, H. Chen and B. Tang, Chem. Commun., 2015, 51, 3102–3105 RSC.
- B. Zhang, C. Ge, J. Yao, Y. Liu, H. Xie and J. Fang, J. Am. Chem. Soc., 2015, 137, 757–769 CrossRef CAS PubMed.
- X. Wang, J. Lv, X. Yao, Y. Li, F. Huang, M. Li, J. Yang, X. Ruan and B. Tang, Chem. Commun., 2014, 50, 15439–15442 RSC.
- X.-F. Yang, Q. Huang, Y. Zhong, Z. Li, H. Li, M. Lowry, J. O. Escobedoc and R. M. Strongin, Chem. Sci., 2014, 5, 2177–2183 RSC.
- J. Liu, Y.-Q. Sun, Y. Huo, H. Zhang, L. Wang, P. Zhang, D. Song, Y. Shi and W. Guo, J. Am. Chem. Soc., 2014, 136, 574–577 CrossRef CAS PubMed.
- L. Song, T. Jia, W. Lu, N. Jia, W. Zhang and J. Qian, Org. Biomol. Chem., 2014, 12, 8422–8427 CAS.
- R. Joseph, B. Ramanujam, A. Acharya and C. P. Rao, J. Org. Chem., 2009, 74, 8181–8190 CrossRef CAS PubMed.
- R. K. Pathak, K. Tabbasum, V. K. Hinge and C. P. Rao, Chem. – Eur. J., 2011, 17, 13999–14003 CrossRef CAS PubMed.
- R. K. Pathak, V. K. Hinge, K. Mahesh, A. Rai, D. Panda and C. P. Rao, Anal. Chem., 2012, 84, 6907–6913 CrossRef CAS PubMed.
- V. V. S. Mummidivarapu, D. S. Yarramala, K. K. Kondaveeti and C. P. Rao, J. Org. Chem., 2014, 79, 10477–10486 CrossRef CAS PubMed.
- S. Areti, R. Teotiab and C. P. Rao, Analyst, 2015, 140, 7391–7398 RSC.
- W. Lin, L. Yuan, Z. Cao, Y. Feng and L. Long, Chem. – Eur. J., 2009, 15, 5096–5103 CrossRef CAS PubMed.
- M. Ren, B. Deng, J.-Y. Wang, Z.-R. Liu and W. Lin, J. Mater. Chem. B, 2015, 3, 6746–6752 RSC.
Footnote |
† Electronic supplementary information (ESI) available. See DOI: 10.1039/c6tb02610a |
|
This journal is © The Royal Society of Chemistry 2017 |
Click here to see how this site uses Cookies. View our privacy policy here.