DOI:
10.1039/C7SC03130K
(Edge Article)
Chem. Sci., 2017,
8, 7132-7137
Synthesis of azasilacyclopentenes and silanols via Huisgen cycloaddition-initiated C–H bond insertion cascades†
Received
17th July 2017
, Accepted 21st August 2017
First published on 4th September 2017
Abstract
An unusual transition metal-free cascade reaction of alkynyl carbonazidates was discovered to form azasilacyclopentenes. Mild thermolysis afforded the products via a series of cyclizations, rearrangements, and an α-silyl C–H bond insertion (rather than the more common Wolff rearrangement, 1,2-shift, or β-silyl C–H insertion) to form silacyclopropanes. A mechanistic proposal for the sequence was informed by control experiments and the characterization of reaction intermediates. The substrate scope and post-cascade transformations were also explored.
Introduction
Carbenes have been employed in cyclopropanations,1 Büchner ring expansion,2 ylide formation,3 X–H bond insertion,4 and especially C–H bond insertions,5 which have provided a powerful tool for synthetic chemistry.6 Cascade reactions using highly reactive carbenes allow the efficient synthesis of complex molecules.7 Our research group recently reported the formation of bridged polycycles via carbene/alkyne cascade reactions terminated in C–H bond insertion (Scheme 1).8 We were also interested in utilizing this cascade reaction to synthesize nitrogen-containing polycyclic compounds like 7. Instead, a complex sequence with an unusual α-silyl C–H bond insertion formed azasilacyclopentene 8 from carbonazidate 5.
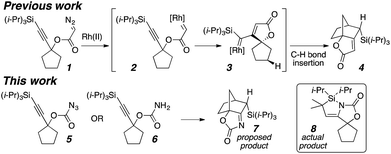 |
| Scheme 1 Carbene- and nitrene-based cascade reactions. | |
Nitrenes have been used for the aziridination of olefins,9 the C–H amination of alkanes,10 and the synthesis of bioactive molecules.11 Pioneering work from Blakey showed nitrene/alkyne cascades proceeded through an endo-cyclization with the alkyne to the postulated vinyl cation/metalloenamine 10a, which potentially could rearrange to the α-iminometallocarbene 10b or react directly (Scheme 2).12 Importantly, metallocarbene 12 could not be accessed via that approach, and α-diazoimines are difficult to synthesize. Carbene 12 could be directly produced from a transition metal catalyzed triazole ring opening.13 The incorporation of C–H bond insertions in these cascade reactions is still rare.14,15 An investigation to generate intermediate 12 and use that highly reactive species and its high potential for complex and interesting transformations has unveiled surprising and novel reactivity. Starting with carbonazidates like 5, carbene 12 was accessed via a metal-free Huisgen cyclization and dediazatization. From this reactive intermediate, C–H bond insertion, bond fragmentations, and pseudopericyclic rearrangements have been identified, and mechanistic intermediates isolated. Reactivity patterns suggest the intermediacy of imino-conjugated triplet carbenes.
 |
| Scheme 2 Nitrene and triazole carbene formation. | |
Results and discussion
The initial goal was to establish a nitrene/alkyne cascade reaction to synthesize nitrogen-containing polycycles like 7. Treating carbamate 13 with Rh2(esp)2 and PhI(OAc)2 resulted in the formation of multiple products: the predicted bridged tricycle 14, propellane 15,16 tetrahydropyranone 16, and silyl vinyl nitrile 17 (Scheme 3).16 A control experiment revealed that acetic acid, produced during the carbamate oxidation, slowly promoted the rearrangement of 14 to propellane 15in situ. This exploratory reaction demonstrated a proof-of-principle for a nitrene/alkyne cascade that results in C–H insertion to generate a C–C bond; however, controlling product selectivity was problematic. Consequently, other nitrene precursors were sought to achieve a more selective outcome.
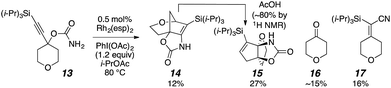 |
| Scheme 3 Cascade reaction of a carbamate. | |
Carbonazidate1718 allowed an improved yield (31%) of tricycle 14 without a catalyst (Scheme 4A). Surprisingly, silanol 19 was also isolated, which was thought to be the result of silica gel-mediated hydrolysis of a labile product during purification since it was not observed in the NMR of the crude reaction material. Dimethyl carbonazidate 20a was prepared to minimize overlapping NMR signals in order to identify the immediate product of the reaction. After 1H and 13C NMR analysis, its structure was determined to be azasilacyclopentene1821a (Scheme 4B). The NMR signals of 21a (vinyl proton: 4.36 ppm; enamine carbons: 108.3 and 150.2 ppm) correlated well to those of oxasilacyclopentene 22.19 In addition, 15N NMR analysis of 15N-enriched azasilacyclopentene 21b (110.5 ppm, no signals from 305 to 375 ppm)20 suggested that the nitrogen was involved in an enamine motif, corroborating the 1H and 13C NMR data. Hydrolysis of 21a to the silanol proved to be facile with a small amount of water.
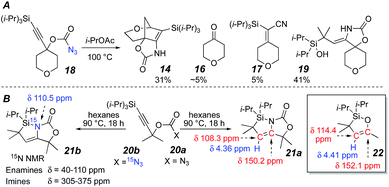 |
| Scheme 4 Cascade reaction of carbonazidates. | |
The products 19 and 21 appeared to be formed via C–H bond insertion at one of the isopropyl groups. In order to bias product formation toward the bridged tricycle by removing all α-silyl C–H bonds, tert-butyldiphenyl silylacetylene carbonazidate 23 was prepared (Scheme 5). The reaction produced five compounds, 24–28. The α-diazo oxazolone 25 and the silanol 28 were crystallized and analyzed by X-ray diffraction to confirm the structures shown.21 In a control experiment, diazoimine 25 was heated again to form 26–28, indicating that it is a viable reaction intermediate and that the vinyl nitrile and ketone products are derived from it. Enyne 24, however, was not observed, demonstrating that it likely formed by elimination from 23.
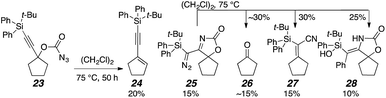 |
| Scheme 5 Avoiding α-silyl C–H bond insertion. | |
These results have been incorporated in a proposed mechanism for the cascade reaction (Scheme 6). Identification of α-diazo imine 25 suggests that a Huisgen cycloaddition22 generated triazole 30, followed by ring opening to 31.13,23 The α-imino carbene 32 (for R3Si = Ph2t-BuSi) could be directly produced via dinitrogen extrusion. Silanol 28 would be produced from 32 by 1,2-phenyl migration (see 33 to 34, Path A)14b and hydration. When R3Si = i-Pr3Si, loss of N2 would form carbene 35, which could proceed through either a transannular ring insertion into the ether-activated methylene (Ha, see Path B), or insertion into the isopropyl C–H bond (Hb, see Path C). The former leads to the bridged tricycles 36 and 14, which can rearrange in the presence of acid to propellane 15 through an elimination/addition sequence.8 The latter pathway would form silacyclopropane 38. Silacyclopropanes are usually formed through silylene transfer,24 but there are a few cases of formation via intramolecular C–H bond insertion, though these intermediates were unstable and were not isolated.25 Likewise, intermediate 38 was not stable, but rearranged26 to azasilacyclopentene 39, which could be observed and characterized spectroscopically (vide supra). Exposure to water rapidly hydrolyzed the weak N–Si bond to give silanol 19.
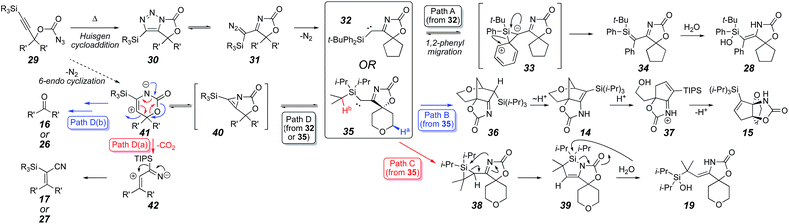 |
| Scheme 6 Proposed mechanism. | |
The isolation of ketone 26 and vinyl nitrile 27 suggested either a 6-endo reaction of a nitrene derived from 29 to form zwitterion 41, or else 41 would be derived from carbenes 32 or 35 (Path D).10–15 Since 26 and 27 were formed from heating diazoimine 25 (Scheme 5), it is likely that the latter route was operative. Therefore, a carbene electrocyclization of 32 (likely pseudopericyclic) would allow the highly strained azirine 40 to be transiently formed.26 Either of the C–N bonds could fragment, with an internal fragmentation forming the ring expanded product 41. A retro-hetero Diels–Alder reaction allows for decarboxylation (Path D(a)), and 1,2-silyl migration in zwitterion 42 would give the vinyl nitriles 17 or 27. Alternatively, C–C bond fragmentation would provide ketones 16 or 26 (Path D(b)). These pathways account for all the observed products and intermediates.
Only a few examples of intramolecular C–H bond insertion to form silacyclopropanes have been reported, and in those cases these intermediates were also fleeting and inferred from downstream products rather than observed directly.25 For simple silicon-substituted carbenes like 44, the triplet state is significantly stabilized relative to the singlet state.27,28 1,2-Rearrangements to silenes are the most common outcomes for these compounds,29 though cyclopropanations and C–H insertions have also been observed in suitable substrates (see 47 and 51). Intramolecular cyclopropanations appear to be possible with silyl carbenes of either a triplet or singlet nature if pendant olefins are present (47 and 49, respectively). On the other hand, the presence of a carbonyl induces more singlet-like reactivity,28,30 including Wolff rearrangements and X–H insertion reactions.31 Intramolecular C–H bond insertions may also occur for these cases, but the preference for insertion is typically at the β- or γ-position relative to silicon (see 60).32 A single report exists for imine-adjacent silyl carbene formation, which is also derived from a triazole precursor (61). In this case, the sole product was a 1,2-silyl shift to give ketene imine 63. Based on these precedents, products 36 (intramolecular C–H bond insertion) or 66 (1,2-shift: see 34, Scheme 6) would have been expected to dominate this reaction, but instead outcomes derived from silacyclopropane formation (see Schemes 3 and 4A) dominated when C–H bond insertion was possible and the formation of 66 and derivatives thereof were not observed.
The unanticipated formation of the azasilacyclopentenes from the reaction cascade prompted further investigation. The acyclic carbonazidate 20a was selected to bias the reaction for azasilacyclopentene formation by eliminating competitive C–H insertion in an appended ring (Table 1, entries 1–5). A lower yield of 21a resulted from the use of a halogenated solvent, dichloroethane, but the use of hexanes resulted in fewer byproducts and a slightly higher yield (entry 3). However, the use of Rh2(esp)2 or Cp*RuCl(cod)14 was deleterious (entries 4 and 5).
Table 1 Variation of the silyl alkyl group34

|
Yield based on 1H NMR peak integration relative to methyl-4-nitro-benzoate.
Isolated yields (average of 2 trials).
1 mol% Rh2(esp)2.
2 mol% Cp*RuCl(cod).
|
|
We were curious if C–H bond insertion would take place at different positions on larger silyl alkanes, or the α-position was preferred. However, all of the alkyl groups only generated the azasilacyclopentenes corresponding to insertion into the C–H bond alpha to silicon (entries 6–9).19b These results contradict literature examples of singlet or metal carbene insertion into alkylsilanes, which show insertion primarily at the methylene or methine beta to silicon,33 presumably due to the “β-silicon effect”. In the examples in Table 1, none of the available β, γ, or δ-C–H bonds reacted. High regioselectivity was shown even in the isobutyl substrate 69f, where the electron-rich β-methine was untouched.
It appears that unlike the other acyl silyl carbenes in Scheme 7, the carbenes 32 and 35 are triplet carbenes and therefore exhibit diradical character.35 The C–C bond formation would then proceed via hydrogen atom abstraction from the carbon attached to the silicon in 71 to generate an α-silyl radical3672 (Scheme 8), which would be stabilized by backbonding from silicon δ-orbitals.37 Then, the silacyclopropane 73 would be formed from radical combination.
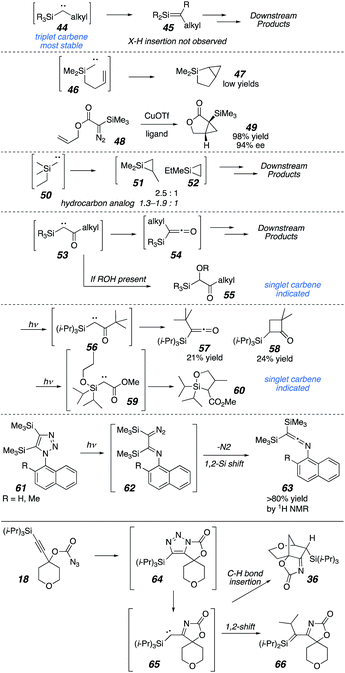 |
| Scheme 7 Typical outcomes for silyl-substituted carbenes. | |
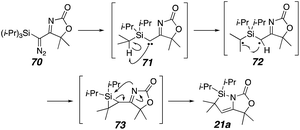 |
| Scheme 8 Proposed triplet carbene/diradical pathway. | |
Next, a series of acyclic, cyclic, and heterocyclic carbonazidates was examined (Table 2). Varying the alkyl groups at R1 and R2 of 74 does not significantly affect the formation of the azasilacyclopentene. Although an allyl group could potentially undergo cyclopropanation with an intermediate silyl carbene,32a,38 only the azasilacyclopentene 75e is seen (entry 5). Cyclic substrates generally provided spiro-oxazolones (entry 6–10). Bridged polycycles were not observed, even for pyrrolidine 74i and thiolane 74j, where the heteroatoms could activate α-C–H bonds for carbene insertion.39 Two substrates with six-membered rings provided a different result, presumably due to greater conformational flexibility to react with the activated C–H bonds compared to the 5-membered rings (entries 11 and 12). For the 3-methyl cyclohexane 74k, the reaction produced a 1
:
1 ratio of azasilacyclopentene 75k and bridged tricycle 77. For the tosyl piperidine 74l, the major isolated product was the silanol 76l, but some bridged bicycle was observed.40
Table 2 Propargylic variation in the substrates34

|
Yield based on 1H NMR peak integration relative to methyl-4-nitro-benzoate.
Isolated yields (average of 2 trials).
Hexanes, 90 °C.
Isopropyl acetate, 100 °C.
Hexanes, 100 °C.
|
|
Preliminary tests were made for transformations of the azasilacyclopentene products. Methyl silyl ether 79 is obtained in good yield by ring opening of 21a in methanol. Treatment of 21a with CuBr2 generated spiro-hemiaminal 80.16 Silanol 69a also produced 80 with CuBr2, suggesting that 21a is first hydrolyzed by adventitious water before spirocycle formation (Scheme 9).
 |
| Scheme 9 Reactions of azasilacyclopentene. | |
Conclusions
A novel approach for the synthesis of azasilacyclopentenes via a Huisgen cycloaddition-initiated cascade reaction terminating in an α-silyl C–H bond insertion has been discovered. Control experiments suggest a likely mechanism with triplet silylcarbene intermediates undergoing C–H bond insertion at the carbon attached to silicon to form transient silacyclopropanes. Further reaction of the azasilacyclopentene showed the formation of interesting products. Ongoing efforts are seeking selective synthesis of bridged polycycles and vinyl nitriles from the same precursors.
Conflicts of interest
There are no conflicts of interest to declare.
Acknowledgements
The authors thank the Welch Foundation (grant E-1744) and the NSF (grant CHE-1352439) for funding. J.-L. S. is grateful to CBIP (UH) and the Studying Abroad Scholarship of Taiwan for fellowships. They are also grateful to Dr Ilja Popovs for discussion of the spectroscopic characterization of mechanistic intermediates.
Notes and references
-
(a) C. Qin, V. Boyarskikh, J. H. Hansen, K. I. Hardcastle, D. G. Musaev and H. M. L. Davies, J. Am. Chem. Soc., 2011, 133, 19198 CrossRef CAS PubMed;
(b) B. T. Parr and H. M. L. Davies, Angew. Chem., Int. Ed., 2013, 52, 10044 CrossRef CAS PubMed;
(c) S.-H. Hou, Y.-Q. Tu, L. Liu, F.-M. Zhang, S.-H. Wang and X.-M. Zhang, Angew. Chem., Int. Ed., 2013, 52, 11373 CrossRef CAS PubMed;
(d) H. Lu, W. I. Dzik, X. Xu, L. Wojtas, B. de Bruin and X. P. Zhang, J. Am. Chem. Soc., 2011, 133, 8518 CrossRef CAS PubMed;
(e) V. N. G. Lindsay, C. Nicolas and A. B. Charette, J. Am. Chem. Soc., 2011, 133, 8972 CrossRef CAS PubMed;
(f) A. DeAngelis, R. Panish and J. M. Fox, Acc. Chem. Res., 2016, 49, 115 CrossRef CAS PubMed.
-
(a) P. Panne and J. M. Fox, J. Am. Chem. Soc., 2007, 129, 22 CrossRef CAS PubMed;
(b) A. L. Crombie, J. L. Kane, K. M. Shea and R. L. Danheiser, J. Org. Chem., 2004, 69, 8652 CrossRef CAS PubMed.
-
(a) X. Xu, P. Y. Zavalij and M. P. Doyle, Angew. Chem., Int. Ed., 2012, 51, 9829 CrossRef CAS PubMed;
(b) J. Barluenga, G. Lonzi, L. Riesgo, L. A. López and M. Tomás, J. Am. Chem. Soc., 2010, 132, 13200 CrossRef CAS PubMed;
(c) X. Wang, X. Xu, P. Y. Zavalij and M. P. Doyle, J. Am. Chem. Soc., 2011, 133, 16402 CrossRef CAS PubMed;
(d) X. Xu, P. Y. Zavalij and M. P. Doyle, J. Am. Chem. Soc., 2013, 135, 12439 CrossRef CAS PubMed;
(e) C. Qin and H. M. L. Davies, J. Am. Chem. Soc., 2013, 135, 14516 CrossRef CAS PubMed.
-
(a) Z. Qu, W. Shi and J. Wang, J. Org. Chem., 2004, 69, 217 CrossRef CAS PubMed;
(b) A. DeAngelis, O. Dmitrenko and J. M. Fox, J. Am. Chem. Soc., 2012, 134, 11035 CrossRef CAS PubMed;
(c) T. C. Maier and G. C. Fu, J. Am. Chem. Soc., 2006, 128, 4594 CrossRef CAS PubMed;
(d) S.-F. Zhu, B. Xu, G.-P. Wang and Q.-L. Zhou, J. Am. Chem. Soc., 2012, 134, 436 CrossRef CAS PubMed;
(e) B. Liu, S.-F. Zhu, W. Zhang, C. Chen and Q.-L. Zhou, J. Am. Chem. Soc., 2007, 129, 5834 CrossRef CAS PubMed;
(f) R. Sambasivan and Z. T. Ball, J. Am. Chem. Soc., 2010, 132, 9289 CrossRef CAS PubMed.
-
(a) P. Herrmann and T. Bach, Chem. Soc. Rev., 2011, 40, 2022 RSC;
(b) M. P. Doyle, R. Duffy, M. Ratnikov and L. Zhou, Chem. Rev., 2010, 110, 704 CrossRef CAS PubMed;
(c) J. H. Hansen, T. M. Gregg, S. R. Ovalles, Y. Lian, J. Autschbach and H. M. L. Davies, J. Am. Chem. Soc., 2011, 133, 5076 CrossRef CAS PubMed;
(d) H. Wang, G. Li, K. M. Engle, J.-Q. Yu and H. M. L. Davies, J. Am. Chem. Soc., 2013, 135, 6774 CrossRef CAS PubMed;
(e) H. M. L. Davies and D. Morton, Chem. Soc. Rev., 2011, 40, 1857 RSC;
(f) Y. Lian and H. M. L. Davies, J. Am. Chem. Soc., 2011, 133, 11940 CrossRef CAS PubMed.
-
(a) H. M. L. Davies and J. R. Denton, Chem. Soc. Rev., 2009, 38, 3061 RSC;
(b) J. Yamaguchi, A. D. Yamaguchi and K. Itami, Angew. Chem., Int. Ed., 2012, 51, 8960 CrossRef CAS PubMed;
(c) P. Lu, Z. Gu and A. Zakarian, J. Am. Chem. Soc., 2013, 135, 14552 CrossRef CAS PubMed;
(d) P. Lu, A. Mailyan, Z. Gu, D. M. Guptill, H. Wang, H. M. L. Davies and A. Zakarian, J. Am. Chem. Soc., 2014, 136, 17738 CrossRef CAS PubMed;
(e) O. Daugulis, J. Roane and L. D. Tran, Acc. Chem. Res., 2015, 48, 1053 CrossRef CAS PubMed.
-
(a) A. Padwa and M. D. Weingarten, Chem. Rev., 1996, 96, 223 CrossRef CAS PubMed;
(b) T. R. Hoye, J. Am. Chem. Soc., 1991, 113, 4343 CrossRef CAS;
(c) Z. Zheng and L. Zheng, Org. Chem. Front., 2015, 2, 1556 RSC;
(d) A. Padwa, Chem. Soc. Rev., 2009, 38, 3072 RSC.
-
(a) S. Jansone-Popova and J. A. May, J. Am. Chem. Soc., 2012, 134, 17877 CrossRef CAS PubMed;
(b) S. Jansone-Popova, P. Q. Le and J. A. May, Tetrahedron, 2014, 70, 4118 CrossRef CAS;
(c) P. Q. Le and J. A. May, J. Am. Chem. Soc., 2015, 137, 12219 CrossRef CAS PubMed.
-
(a) J. W. Rigoli, C. D. Weatherly, J. M. Alderson, B. T. Vo and J. M. Schomaker, J. Am. Chem. Soc., 2013, 135, 17238 CrossRef CAS PubMed;
(b) A. A. Desai and W. D. Wulff, J. Am. Chem. Soc., 2010, 132, 13100 CrossRef CAS PubMed;
(c) M. R. Fructos, E. Álvarez, M. M. Díaz-Requejo and P. J. Pérez, J. Am. Chem. Soc., 2010, 132, 4600 CrossRef CAS PubMed;
(d) L. Maestre, W. M. C. Sameera, M. M. Díaz-Requejo, F. Maseras and P. J. A. Pérez, J. Am. Chem. Soc., 2013, 135, 1338 CrossRef CAS PubMed;
(e) K. Guthikonda and J. Du Bois, J. Am. Chem. Soc., 2002, 124, 13672 CrossRef CAS PubMed.
-
(a) E. Milczek, N. Boudet and S. Blakey, Angew. Chem., Int. Ed., 2008, 47, 6825 CrossRef CAS PubMed;
(b) D. N. Zalatan and J. Du Bois, J. Am. Chem. Soc., 2008, 130, 9220 CrossRef CAS PubMed;
(c) Q. Nguyen, T. Nguyen and T. G. Driver, J. Am. Chem. Soc., 2013, 135, 620 CrossRef CAS PubMed;
(d) A. Nörder, S. A. Warren, E. Herdtweck, S. M. Huber and T. Bach, J. Am. Chem. Soc., 2012, 134, 13524 CrossRef PubMed;
(e) D. N. Zalatan and J. Du Bois, J. Am. Chem. Soc., 2009, 131, 7558 CrossRef CAS PubMed.
-
(a) G. Dequirez, V. Pons and P. Dauban, Angew. Chem., Int. Ed., 2012, 51, 7384 CrossRef CAS PubMed;
(b) A. Sloan Devlin and J. Du Bois, Chem. Sci., 2013, 4, 1059 RSC;
(c) J. J. Fleming, M. D. McReynolds and J. Du Bois, J. Am. Chem. Soc., 2007, 129, 9964 CrossRef CAS PubMed;
(d) A. Hinman and J. Du Bois, J. Am. Chem. Soc., 2003, 125, 11510 CrossRef CAS PubMed;
(e) J. V. Mulcahy and J. Du Bois, J. Am. Chem. Soc., 2008, 130, 12630 CrossRef CAS PubMed;
(f) K. W. Quasdorf, A. D. Huters, M. W. Lodewyk, D. J. Tantillo and N. K. Garg, J. Am. Chem. Soc., 2012, 134, 1396 CrossRef CAS PubMed.
-
(a) A. R. Thornton and S. B. Blakey, J. Am. Chem. Soc., 2008, 130, 5020 CrossRef CAS PubMed;
(b) A. R. Thornton, V. I. Martin and S. B. Blakey, J. Am. Chem. Soc., 2009, 131, 2434 CrossRef CAS PubMed;
(c) A. H. Stoll and S. B. Blakey, J. Am. Chem. Soc., 2010, 132, 2108 CrossRef CAS PubMed;
(d) A. H. Stoll and S. B. Blakey, Chem. Sci., 2011, 2, 112 RSC;
(e) N. Mace, A. R. Thornton and S. B. Blakey, Angew. Chem., Int. Ed., 2013, 52, 5836 CrossRef CAS PubMed;
(f) A. Boyer, J. Org. Chem., 2015, 80, 4771 CrossRef CAS PubMed.
-
(a) J. E. Spangler and H. M. L. Davies, J. Am. Chem. Soc., 2013, 135, 6802 CrossRef CAS PubMed;
(b) J. S. Alford, J. E. Spangler and H. M. L. Davies, J. Am. Chem. Soc., 2013, 135, 11712 CrossRef CAS PubMed;
(c) B. T. Parr, S. A. Green and H. M. L. Davies, J. Am. Chem. Soc., 2013, 135, 4716 CrossRef CAS PubMed;
(d) T. Miura, Y. Funakoshi and M. Murakami, J. Am. Chem. Soc., 2014, 136, 2272 CrossRef CAS PubMedE. E. Schultz and R. Sarpong, J. Am. Chem. Soc., 2013, 135, 4696 CrossRef CAS PubMed;
(e) T. Miura, T. Tanaka, K. Hiraga, S. G. Stewart and M. Murakami, J. Am. Chem. Soc., 2013, 135, 13652 CrossRef CAS PubMed;
(f) T. Horneff, S. Chuprakov, N. Chernyak, V. Gevorgyan and V. V. Fokin, J. Am. Chem. Soc., 2008, 130, 14972 CrossRef CAS PubMed;
(g) J. S. Alford and H. M. L. Davies, J. Am. Chem. Soc., 2014, 136, 10266 CrossRef CAS PubMed.
-
(a) S. Chuprakov, J. A. Malik, M. Zibinsky and V. V. Fokin, J. Am. Chem. Soc., 2011, 133, 10352 CrossRef CAS PubMed;
(b) B. C. Boren, S. Narayan, L. K. Rasmussen, L. Zhang, H. Zhao, Z. Lin, G. Jia and V. V. Fokin, J. Am. Chem. Soc., 2008, 130, 8923 CrossRef CAS PubMed.
- V. N. G. Lindsay, H. M. F. Viart and R. Sarpong, J. Am. Chem. Soc., 2015, 137, 8368 CrossRef CAS PubMed.
- Structure confirmed via single crystal X-ray diffraction.
-
(a) R. Singh, J. N. Kolev, P. A. Sutera and R. Fasan, ACS Catal., 2015, 5, 1685 CrossRef CAS PubMed;
(b) T. Heinisch and T. R. Ward, Eur. J. Inorg. Chem., 2015, 2015, 3406 CrossRef CAS;
(c) T. Bach, B. Schlummer and K. Harms, Chem.–Eur. J., 2001, 7, 2581 CrossRef CAS PubMed;
(d) D. P. Uccello, S. M. Miller, N. A. Dieterich, A. F. Stepan, S. Chung, K. A. Farley, B. Samas, J. Chen and J. I. Montgomery, Tetrahedron Lett., 2011, 52, 4247 CrossRef CAS.
- Z. Nevárez and K. A. Woerpel, Org. Lett., 2007, 9, 3773 CrossRef PubMed.
-
(a) S. A. Calad and K. A. Woerpel, J. Am. Chem. Soc., 2005, 127, 2046 CrossRef CAS PubMed;
(b) R. Bruckmann and G. Maas, Chem. Ber., 1987, 120, 635 CrossRef.
-
G. C. Kevy and R. L. Lichter, Nitrogen-15 Nuclear Magnetic Resonance Spectroscopy, J. Wiley, New York, NY, 1979 Search PubMed.
- Crystal structures have been deposited in the Cambridge Crystallographic Data Centre, reference numbers 1477662–1477666 and 1477728.†.
-
(a) S. Bräse, C. Gil, K. Knepper and V. Zimmermann, Angew. Chem., Int. Ed., 2005, 44, 5188 CrossRef PubMed;
(b) R. G. Brisbois, A. M. Bergan, A. J. Ellison, P. Y. Griffin, K. C. Hackbarth and S. R. Larson, Tetrahedron Lett., 2013, 54, 272 CrossRef CAS.
- G. Mitchell and C. W. Rees, J. Chem. Soc., Perkin Trans. 1, 1987, 413 RSC.
-
(a) A. K. Franz and K. A. Woerpel, Acc. Chem. Res., 2000, 33, 813 CrossRef CAS PubMed;
(b) T. G. Driver, A. K. Franz and K. A. Woerpel, J. Am. Chem. Soc., 2002, 124, 6524 CrossRef CAS PubMed;
(c) J. Ćiraković, T. G. Driver and K. A. Woerpel, J. Am. Chem. Soc., 2002, 124, 9370 CrossRef;
(d) T. G. Driver and K. A. Woerpel, J. Am. Chem. Soc., 2003, 125, 10659 CrossRef CAS PubMed.
-
(a) J. W. Connolly and G. J. Urry, J. Org. Chem., 1964, 29, 619 CrossRef CAS;
(b) J. W. Connolly, J. Organomet. Chem., 1968, 11, 429 CrossRef CAS.
-
(a) R. Huisgen, Angew. Chem., Int. Ed. Engl., 1980, 19, 947 CrossRef;
(b) H. Ji, L. Li, X. Xu, S. Ham, L. A. Hammad and D. M. Birney, J. Am. Chem. Soc., 2009, 131, 528 CrossRef CAS PubMed.
-
G. Maas, Silicon-Substituted Carbenes, John Wiley & Sons, Ltd, Chichester, UK, 2nd edn, 2009, vol. I Search PubMed.
- A. Nemirowski and P. R. Schreiner, J. Org. Chem., 2007, 72, 9533 CrossRef CAS.
-
(a) W. Ando, T. Hagiwara and T. Migita, J. Am. Chem. Soc., 1973, 95, 7518 CrossRef CAS;
(b) W. Ando, A. Sekiguchi, J. Ogiwara and T. Migita, J. Chem. Soc., Chem. Commun., 1975, 145 RSC.
- K. Hirai, T. Itoh and H. Tomioka, Chem. Rev., 2009, 109, 3275 CrossRef CAS PubMed.
-
(a) W. Ando, A. Sekiguchi, T. Hagiwara, T. Migita, V. Chowdhry, F. H. Westheimer, S. L. Kammula, M. Green and M. Jones, J. Am. Chem. Soc., 1979, 101, 6393 CrossRef CAS;
(b) R. Brückmann, K. Schneider and G. Maas, Tetrahedron, 1989, 45, 5517 CrossRef;
(c) M. J. Davies, C. J. Moody and R. J. Taylor, J. Chem. Soc., Perkin Trans. 1, 1991, 1 RSC;
(d) M. Alt and G. Maas, Tetrahedron, 1994, 50, 7435 CrossRef CAS;
(e) S. P. Marsden and W. K. Pang, Tetrahedron Lett., 1998, 39, 6077 CrossRef CAS;
(f) D. C. Braddock, D. M. Badine, T. Gottschalk, A. Matsuno and M. Rodriguez-Lens, Synlett, 2003, 345 CrossRef CAS.
-
(a) U. Schöllkopf, D. Hoppe, N. Rieber and V. Jacobi, Eur. J. Org. Chem., 1969, 730, 1 Search PubMed;
(b) R. Brückmann, K. Schneider and G. Maas, Tetrahedron, 1989, 45, 5517 CrossRef.
-
(a) D. Seyferth, S. S. Washburne and C. J. Attridge, J. Am. Chem. Soc., 1970, 92, 4405 CrossRef CAS;
(b) P. Wang and J. Adams, J. Am. Chem. Soc., 1994, 116, 3296 CrossRef CAS;
(c) Y. Hatanaka, M. Watanabe, S.-Y. Onozawa, M. Tanaka and H. Sakurai, J. Org. Chem., 1998, 63, 422 CrossRef CAS PubMed.
- Although azasilacyclopentenes can be observed in the NMR and GC/MS of the crude reaction mixture, they do not survive silica gel chromatography. Thus, NMR-based yields are reported for the azasilacyclopentenes and isolated yields are reported for the corresponding silanols.
-
(a) M. J. Ellis and M. F. G. Stevens, J. Chem. Soc., Perkin Trans. 1, 2001, 3174 RSC;
(b) D. J. Hagan, D. Chan, C. H. Schwalbe and M. F. G. Stevens, J. Chem. Soc., Perkin Trans. 1, 1998, 915 RSC.
- R. Walsh, Acc. Chem. Res., 1981, 14, 246 CrossRef CAS.
-
(a) J. W. Wilt, O. Kolewe and J. F. Kraemer, J. Am. Chem. Soc., 1969, 91, 2624 CrossRef CAS;
(b) T. Manabe, S.-I. Yanagi, K. Ohe and S. Uemura, Organometallics, 1998, 17, 2942 CrossRef CAS;
(c) Y. J. Lee, R. Linf and P. S. Mariano, J. Org. Chem., 1996, 61, 3304 CrossRef CAS;
(d) S. Bogen, L. Fensterbank and M. Malacria, J. Org. Chem., 1999, 64, 819 CrossRef CAS PubMed;
(e) P. Grieco, Y. Yokoyama and E. Williams, J. Org. Chem., 1978, 43, 1285 CrossRef;
(f) M. Cocivera and E. Grunwald, J. Am. Chem. Soc., 1965, 87, 2071 CrossRef;
(g) P. J. Krusic and J. K. Kochi, J. Am. Chem. Soc., 1969, 91, 6161 CrossRef CAS.
-
(a) W. Ando, A. Sekiguchi, T. Hagiwara and T. Migita, J. Chem. Soc., Chem. Commun., 1974, 372 RSC;
(b) T. J. Barton, J. A. Kilgour, R. G. Gallucci, A. J. Rothschild, J. Slutsky, A. D. Wolf and M. Jones, J. Am. Chem. Soc., 1975, 97, 657 CrossRef CAS;
(c) G. Maas, B. Daucher, A. Maier and V. Gettwert, Chem. Commun., 2004, 26, 238 RSC;
(d) S. Inoue, K. Nagatani, H. Tezuka, Y. Hoshino and M. Nakada, Synlett, 2017, 28, 1065 CrossRef CAS.
-
i-PrOAc was used for solubility.
- The azasilylcyclopentene intermediate could not accurately be quantified.
Footnotes |
† Electronic supplementary information (ESI) available: Full experimental details and compound characterizations, including X-ray diffraction data, are provided. CCDC 1477662–1477666 and 1477728. For ESI and crystallographic data in CIF or other electronic format see DOI: 10.1039/c7sc03130k |
‡ Current affiliation: Chemical Science Division, Oak Ridge National Laboratory, Oak Ridge, Tennessee 37831-6119, UAS. |
|
This journal is © The Royal Society of Chemistry 2017 |
Click here to see how this site uses Cookies. View our privacy policy here.