DOI:
10.1039/C6SC05469B
(Edge Article)
Chem. Sci., 2017,
8, 2885-2889
Dual gold and photoredox catalysed C–H activation of arenes for aryl–aryl cross couplings†
Received
14th December 2016
, Accepted 26th January 2017
First published on 27th January 2017
Abstract
A mild and fully catalytic aryl–aryl cross coupling via gold-catalysed C–H activation has been achieved by merging gold and photoredox catalysis. The procedure is free of stoichiometric oxidants and additives, which were previously required in gold-catalysed C–H activation reactions. Exploiting dual gold and photoredox catalysis confers regioselectivity via the crucial gold-catalysed C–H activation step, which is not present in the unselective photocatalysis-only counterpart.
Introduction
The increased drive to develop more sustainable methods for synthesis has led to a surge in research on C–H functionalisations.1 Within this context, direct aryl C–H functionalisations using gold catalysis2 is a relatively young and overlooked field compared to the more developed palladium, ruthenium and rhodium counterparts. Nevertheless, the mild conditions under which gold-catalysis can activate C–H bonds, as well as the regioselectivity observed in the absence of directing groups,2 provides many golden opportunities for this developing field. In the specific area of aryl–aryl cross-couplings via C–H activation, Lloyd-Jones and Russell elegantly showcased that gold catalysis can be used to site selectively arylate arylsilanes (Scheme 1a).3 More recently, Larrosa disclosed his seminal work on oxidative cross-couplings via double C–H activation to couple electron-poor with electron-rich arenes.4 Despite these advances, there remain several limitations, one of which is the often limited arene substrate scope.2a The other major limitation is the requirement for a stoichiometric oxidant to access the Au(I)/Au(III) cycle required for cross-couplings:2,5 the benefit of employing C–H activation to avoid arene prefunctionalisation is thus somewhat offset by the generation of stoichiometric organic waste from the oxidant, and the use of the latter can also limit functional group tolerance. There is therefore a clear need to develop couplings that do not require stoichiometric oxidants.2a Within this context, we herein disclose the first dual gold and photoredox catalysed aryl–aryl cross coupling via C–H activation (Scheme 1b), which also constitutes the first gold-catalysed C(sp2)-H activation reaction which does not require stoichiometric oxidants.
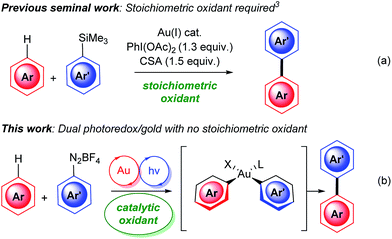 |
| Scheme 1 Gold-catalysed aryl–aryl couplings via C–H activation. | |
The use of dual6 gold and photoredox catalysis7 to access Au(I)/Au(III) catalytic cycles was recently pioneered by Glorius8 and Toste.9–11 Its use in cross-couplings has only very recently been reported: Sonogashira-type couplings12 and Suzuki-type couplings were revealed this year, the latter independently by our group13 and Fouquet.14,15 To the best of our knowledge, however, aryl–aryl couplings via C(sp2)-H activation using dual gold and photoredox catalysis has yet to be achieved, although it was recently attempted by Maestri and Malacria.16 Under their conditions, they instead discovered that the coupling between unactivated arenes and diazonium salts could occur under photocatalysis-only conditions (no gold) through mechanistically distinct formal homolytic aromatic substitutions, which does not involve C–H activation. However, poor regioselectivities (mixtures of ortho, meta and para coupling) were observed and 40 equivalents of arene were generally required for this radical reaction.16 Therefore, aryl–aryl couplings via C(sp2)-H activation involving dual gold and photoredox catalysis is clearly desirable, as it will not only prove for the first time that catalytic oxidants can be utilised in the general field of gold-catalysed C–H activations, but it should also significantly improve the regioselectivities and arene equivalents in the aryl–aryl couplings, compared to the mechanistically distinct photocatalysis-only reaction.
Results and discussion
Since electrophilic Au(III) is known to C–H activate electron rich arenes,3g,17–19 and using insights gained from our previous studies,13 we surmised that a combination of an aryldiazonium salt20 with PPh3AuNTf2 and a photoredox catalyst should furnish an electrophilic aryl Au(III) species (III, Scheme 3) capable of C–H activating a suitable arene in order to form our cross-coupled product (see later for mechanism). We thus initiated our studies using mesitylene 1a as the arene with Ru(bpy)3(PF6)2 as the photoredox catalyst (Table 1). To our delight, the coupling product 3aa was observed in a promising 31% yield (Entry 1).
Table 1 Selected optimisation reactions
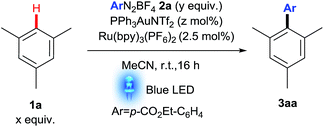
|
Entrya |
x
|
y
|
z
|
Modification |
Yieldb (%) |
Degassed MeCN.
Determined by 1H NMR analysis using dimethylsulfone as internal standard.
|
1 |
1 |
2 |
5 |
— |
31 |
2 |
1 |
1 |
5 |
— |
51 |
3
|
3
|
1
|
5
|
—
|
67
|
4 |
10 |
1 |
5 |
— |
54 |
5 |
3 |
1 |
5 |
Eosin Y instead of [Ru] |
62 |
6 |
3 |
1 |
5 |
Fluorescein instead of [Ru] |
58 |
7
|
3
|
1
|
10
|
—
|
81
|
Crucially, control experiments in the absence of gold catalyst,21 Ru catalyst or light resulted in little or no conversion (see ESI†), confirming that it is a dual gold/photoredox coupling reaction under these conditions (see Scheme 2 for further confirmation). Optimisation studies showed that a small excess of arene 1a is beneficial (Entry 3) but a large excess hampers the reaction in this case (Entry 4). Employment of organic dyes22 eosin Y and fluorescein instead of Ru(bpy)3(PF6)2 proved to be a potentially greener alternative (Entries 5–6), although we opted to continue our studies using the better performing Ru catalyst. Finally, a good 3aa yield of 81% was achieved by increasing the gold catalyst loading (Entry 7).23
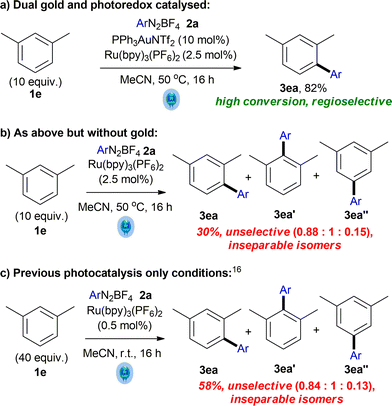 |
| Scheme 2 Dual gold and photoredox catalysis confers regioselectivity. | |
With these optimised conditions in hand, an aryldiazonium substrate scope was carried out (Table 2). Ester-(3aa) and amide-substituted (3ak) substrates, as well as halogenated substrates 3ab–3ae react smoothly (50–80%), as do meta- and para-substituted nitro substrates (3af–3ag). The ortho-substituted 3ah, however, is furnished in a modest 37% yield, presumably due to steric effects. Yields of 3ai and 3aj were moderate under standard conditions, but the yield of 3ai was successfully improved to 60% under more forcing conditions (10 equiv. 1a and 50 °C). Predictably,8a,12b,13 electron-rich aryldiazoniums react more sluggishly, with decreasing yields observed with more electron rich aryls (3am–3an 48%, while 3ao < 26%).
Table 2 Aryldiazonium scope
Method A: 2 (0.1 mmol), arene (3 equiv.) [Ru] and [Au] were dissolved in degassed MeCN, and stirred at rt under blue LED irradiation.
Method B: 3 equiv. of 1a, 50 °C.
Method C: 10 equiv. 1a, 50 °C. Isolated yields reported.
|
|
As for the arene scope, Au(III)-mediated C–H activation is known to proceed via electrophilic aromatic substitution onto Au(III) (see later), thereby rendering electron-poor arenes unsuitable candidates for these conditions. With this in mind, suitable electron neutral and electron rich arenes were evaluated as shown in Table 3. While steric hindrance in the form of double ortho substitution is tolerated in mesitylene 3aa (73%), the yield begins to drop off with increasingly hindered ortho-substituents (3ba, 3ca). Para- and meta-xylene also couples with high yields (82%), as does toluene (3da) and tbutylbenzene (3ea). Predictably, 3da and 3ea are formed as o-/p-isomers, although the major para-3da can be isolated in a good 56% yield. The p-/o- ratio is a good 5.7
:
1 for the more hindered 3ga.
Table 3 Arene scope
Method A: 2 (0.1 mmol), arene (3 equiv.) [Ru] and [Au] were dissolved in degassed MeCN, and stirred at rt under blue LED irradiation.
Method B: 3 equiv. of 1a, 50 °C.
Method C: 10 equiv. 1a, 50 °C. Isolated yields reported.
|
|
This is in stark contrast to the photocatalysis-only reaction (Scheme 2). In the absence of gold, yield (30%) and selectivity (0.88
:
1
:
0.15 of 3ea
:
3ea′
:
3ea′′) are both very poor (Scheme 2b) compared to the fully selective dual catalytic reaction (Scheme 2a). Adopting the literature photocatalysis-only conditions16 also result in a similarly unselective reaction, although the conversion is improved (58% combined yield of inseparable isomers, Scheme 2c). These controls show the significant benefit of utilising the regioselectivity conferred by the gold C–H activation step in the dual gold and photoredox reaction (Scheme 2a) and is further proof that the reaction described here is not a photocatalysis-only reaction.
Next, mesitylenes bearing electron-withdrawing substituents successfully couple (Table 3, 3ia–3ja, 52–57%), although the yield drops to 37% in the presence of the more withdrawing CN group (3ka). Biphenyl pleasingly reacts exclusively at the para-position to yield triaryl 3la in 56% yield. Finally, very electron rich arenes and heteroarenes such as 1,3,5-trimethoxybenzene and N-methyl indole do not currently cross-couple well under these conditions, due to competing azo coupling (see ESI†).24,25
Pleasingly, however, more electron rich arenes are viable arenes for intramolecular C–H couplings, as exemplified by the formation of 5 in 83% yield (Scheme 3). While these cyclisations have been attempted under photocatalysis-only conditions, reported yields were very low (0–25%) due to competing deazotisation.26 Carbazole277 can also be accessed from 6 in 82% yield, which is of note as traditional Pschorr cyclisations28 do not typically work well for carbazoles. Indeed, 5 and 7 are only formed in 36% and 41% (NMR yields) respectively in the absence of gold. Moreover, the readily oxidisable sulfide (4) and benzyl (6) are tolerated under these conditions, showcasing the potential of dual catalysis to significantly improve the C–H activation cross coupling under mild conditions compared to previously required stoichiometric oxidant conditions.
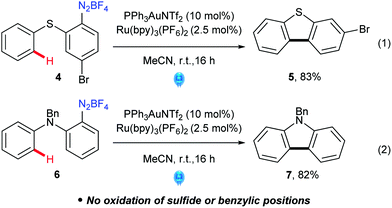 |
| Scheme 3 Intramolecular aryl–aryl coupling via C–H activation. | |
Based on a combination of various literature reports,8a,9a,12b,13,29 a plausible mechanism for the cross-coupling is shown in Scheme 4. Initial oxidation of the Au(I) catalyst Ivia addition of an aryl radical (I → II), is followed by a subsequent SET to form Au(III) intermediate III,30 regenerating the photocatalyst. Alternatively, quantum yield calculations carried out on related dual triphenylphosphine gold/visible-light catalysed systems revealed that species II can also undergo SET with another equivalent of diazonium salt, to simultaneously yield the Au(III) species III along with an aryl radical.12b The arene partner then undergoes electrophilic auration with the Lewis acidic species III to give intermediate IV, which explains the regioselectivities observed.3g,17–19 The corresponding intermediate V then reductively eliminates to form the cross-coupled product 3, while regenerating Au(I) catalyst I.
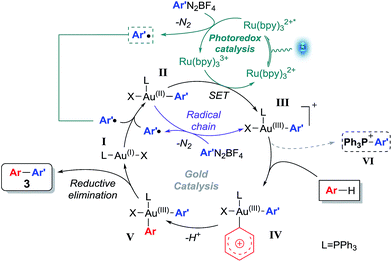 |
| Scheme 4 Plausible mechanism. | |
In order to lend support to this mechanism, two reactions were set up using equimolar amounts of PPh3AuNTf2, 1a and 2d, and 2.5 mol% of Ru(bpy)3(PF6)2 in the presence and absence of light respectively. 31P NMR monitoring reveals that a new signal at δ 23.1 ppm appears for the irradiated reaction (Fig. 1), but is absent from the dark reaction. The transient species III is highly unstable31 and cannot be isolated, however, the signal at δ 23.1 ppm corresponds to species VI
32 which is formed by reductive elimination of III.9a The detection of VI therefore implies that III is present in the reaction.9a,13
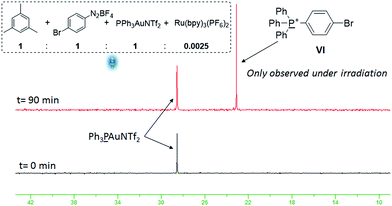 |
| Fig. 1
31P NMR studies in CD3CN. | |
Furthermore, control experiments using various gold(I) species fail to form the coupling product 3 (see ESI†), lending further support to the hypothesis that intermediate III is the key species in the crucial electrophilic auration step. In addition, control experiments shown in Scheme 2 confirm that the mechanism is distinct from the formal homolytic aromatic substitutions observed in the photocatalysis-only reactions, since the regioselectivity observed supports the electrophilic auration step shown in Scheme 4 rather than the former, which is unselective. Additionally, the involvement of an aryl cation intermediate from the aryldiazonium salt 2
33 can also be discounted by the fact that electron withdrawing aryldiazoniums react more readily than their electron rich counterparts (Table 2).
Conclusions
In conclusion, we have developed the first dual gold/photoredox method for aryl–aryl cross coupling via direct C–H activation of arenes under mild conditions. The use of dual catalysis has allowed us to address and overcome a major limitation encountered with gold-catalysed C–H activations: the requirement for stoichiometric oxidants and its corresponding waste. As is the case with current gold-catalysed C–H activation reactions,2 the arene substrate scope for the intermolecular coupling still has its limitations (although the intramolecular version shows great promise) and addressing this issue remains a future challenge for the field. Nevertheless, we envisage that the development of the first fully catalytic system constitutes significant progress for the field of gold-catalysed C–H activation and functionalisation of arenes. In addition, control experiments show that exploiting dual gold and photoredox catalysis confers regioselectivity via the crucial gold-catalysed C–H activation step, which is not present in the unselective photocatalysis-only counterpart.
Acknowledgements
We gratefully acknowledge the Leverhulme Trust (RPG-2014-345) for funding and Heriot-Watt University for a James Watt Scholarship (DRS). Mass spectrometry data was acquired at the EPSRC UK National Mass Spectrometry Facility at Swansea University.
Notes and references
- Selected review:
(a) H. M. L. Davies and D. Morton, J. Org. Chem., 2016, 81, 343 CrossRef CAS PubMed;
(b) T. Gensch, M. N. Hopkinson, F. Glorius and J. Wencel-Delord, Chem. Soc. Rev., 2016, 45, 2900 RSC;
(c) J. F. Hartwig and M. A. Larsen, ACS Cent. Sci., 2016, 2, 281 CrossRef CAS PubMed.
- Reviews:
(a) S. Kramer, Chem.–Eur. J., 2016, 22, 15584 CrossRef CAS PubMed;
(b) T. C. Boorman and I. Larrosa, Chem. Soc. Rev., 2011, 40, 1910 RSC;
(c) T. de Haro and C. Nevado, Synthesis, 2011, 2530 CAS.
-
(a) L. T. Ball, G. C. Lloyd-Jones and C. A. Russell, Science, 2012, 337, 1644 CrossRef CAS PubMed;
(b) L. T. Ball, G. C. Lloyd-Jones and C. A. Russell, J. Am. Chem. Soc., 2014, 136, 254 CrossRef CAS PubMed;
(c) A. J. Cresswell and G. C. Lloyd-Jones, Chem.–Eur. J., 2016, 22, 12641 CrossRef CAS PubMed; see also:
(d) K. Hata, H. Ito, Y. Segawa and K. Itami, Beilstein J. Org. Chem., 2015, 11, 2737 CrossRef CAS PubMed; intramolecular:
(e) T. J. A. Corrie, L. T. Ball, C. A. Russell and G. C. Lloyd-Jones, J. Am. Chem. Soc., 2017, 139, 245 CrossRef CAS PubMed; using arylboronic acids:
(f) M. Hofer, A. Genoux, R. Kumar and C. Nevado, Angew. Chem., Int. Ed., 2017, 56, 1021 CrossRef CAS PubMed;
(g) Q. Wu, C. Du, Y. Huang, X. Liu, Z. Long, F. Song and J. You, Chem. Sci., 2015, 6, 288 RSC.
-
(a) X. C. Cambeiro, N. Ahlsten and I. Larrosa, J. Am. Chem. Soc., 2015, 137, 15636 CrossRef CAS PubMed; stoichiometric version:
(b) X. C. Cambeiro, T. C. Boorman, P. Lu and I. Larrosa, Angew. Chem., Int. Ed., 2013, 52, 1781 CrossRef CAS PubMed.
- Review on Au(I)/Au(III) catalysis: M. N. Hopkinson, A. D. Gee and V. Gouverneur, Chem.–Eur. J., 2011, 17, 8248 CrossRef CAS PubMed.
- For reviews on cooperative photoredox catalysis, see:
(a) X. Lang, J. Zhao and X. Chen, Chem. Soc. Rev., 2016, 45, 3026 RSC;
(b) M. N. Hopkinson, B. Sahoo, J.-L. Li and F. Glorius, Chem.–Eur. J., 2014, 20, 3874 CrossRef CAS PubMed.
- Reviews on photoredox gold catalysis:
(a) M. N. Hopkinson, A. Tlahuext-Aca and F. Glorius, Acc. Chem. Res., 2016, 49, 2261 CrossRef CAS PubMed;
(b)
T. McCallum, S. Rohe and L. Barriault, Synlett, DOI:10.1055/s-0036-1588644.
-
(a) B. Sahoo, M. N. Hopkinson and F. Glorius, J. Am. Chem. Soc., 2013, 135, 5505 CrossRef CAS PubMed; see also:
(b) M. N. Hopkinson, B. Sahoo and F. Glorius, Adv. Synth. Catal., 2014, 356, 2794 CrossRef CAS;
(c) A. Tlahuext-Aca, M. N. Hopkinson, R. A. Garza-Sanchez and F. Glorius, Chem.–Eur. J., 2016, 22, 5909 CrossRef CAS PubMed.
-
(a) X.-Z. Shu, M. Zhang, Y. He, H. Frei and F. D. Toste, J. Am. Chem. Soc., 2014, 136, 5844 CrossRef CAS PubMed; see also:
(b) Y. He, H. Wu and F. D. Toste, Chem. Sci., 2015, 6, 1194 RSC.
- For other examples of dual gold/photoredox catalysed reactions, see ref. 12–14 and:
(a) S. Cai, K. Yang and D. Z. Wang, Org. Lett., 2014, 16, 2606 CrossRef CAS PubMed;
(b) D. V. Patil, H. Yun and S. Shin, Adv. Synth. Catal., 2015, 357, 2622 CrossRef CAS;
(c) Z. Xia, O. Khaled, V. Mouriès-Mansuy, C. Ollivier and L. Fensterbank, J. Org. Chem., 2016, 81, 7182 CrossRef CAS PubMed;
(d) J. Um, H. Yun and S. Shin, Org. Lett., 2016, 18, 484 CrossRef CAS PubMed;
(e) B. Alcaide, P. Almendros, E. Busto and A. Luna, Adv. Synth. Catal., 2016, 358, 1526 CrossRef CAS.
- For an elegant alternative, examples of photoredox using only Au catalysts:
(a) L. Huang, F. Rominger, M. Rudolph and A. S. K. Hashmi, Chem. Commun., 2016, 52, 6435 RSC;
(b) L. Huang, M. Rudolph, F. Rominger and A. S. K. Hashmi, Angew. Chem., Int. Ed., 2016, 55, 4808 CrossRef CAS PubMed;
(c) T. McCallum and L. Barriault, Chem. Sci., 2016, 7, 4754 RSC;
(d) G. Revol, T. McCallum, M. Morin, F. Gagosz and L. Barriault, Angew. Chem., Int. Ed., 2013, 52, 13342 CrossRef CAS PubMed;
(e) J. Xie, S. Shi, T. Zhang, N. Mehrkens, M. Rudolph and A. S. K. Hashmi, Angew. Chem., Int. Ed., 2015, 54, 6046 CrossRef CAS PubMed.
-
(a) S. Kim, J. Rojas-Martin and F. D. Toste, Chem. Sci., 2016, 7, 85 RSC;
(b) A. Tlahuext-Aca, M. N. Hopkinson, B. Sahoo and F. Glorius, Chem. Sci., 2016, 7, 89 RSC.
- V. Gauchot and A.-L. Lee, Chem. Commun., 2016, 52, 10163 RSC.
- T. Cornilleau, P. Hermange and E. Fouquet, Chem. Commun., 2016, 52, 10040 RSC.
- R. Cai, M. Lu, E. Y. Aguilera, Y. Xi, N. G. Akhmedov, J. L. Petersen, H. Chen and X. Shi, Angew. Chem., Int. Ed., 2015, 54, 8772 CrossRef CAS PubMed.
- F. Gomes, V. Narbonne, F. Blanchard, G. Maestri and M. Malacria, Org. Chem. Front., 2015, 2, 464 RSC.
- M. S. Kharasch and H. S. Isbell, J. Am. Chem. Soc., 1931, 53, 3053 CrossRef CAS.
- Selected reactions involving Au(iii)-promoted C–H activation, see ref. 3, 4 and:
(a) D. Qiu, Z. Zheng, F. Mo, Q. Xiao, Y. Tian, Y. Zhang and J. Wang, Org. Lett., 2011, 13, 4988 CrossRef CAS PubMed;
(b) A. Pradal, P. Y. Toullec and V. Michelet, Org. Lett., 2011, 13, 6086 CrossRef CAS PubMed;
(c) T. d. Haro and C. Nevado, J. Am. Chem. Soc., 2010, 132, 1512 CrossRef PubMed;
(d) J. P. Brand and J. Waser, Angew. Chem., Int. Ed., 2010, 49, 7304 CrossRef CAS PubMed;
(e) J. P. Brand, J. Charpentier and J. Waser, Angew. Chem., Int. Ed., 2009, 48, 9346 CrossRef CAS PubMed.
- Oxidative homocoupling: A. Kar, N. Mangu, H. M. Kaiser, M. Beller and M. K. Tse, Chem. Commun., 2008, 386 RSC.
- Review of photocatalysed arylations with aryldiazoniums: D. P. Hari and B. König, Angew. Chem., Int. Ed., 2013, 52, 4734 CrossRef CAS PubMed.
- Mechanistically distinct
photoredox coupling between electron rich heterocycles and aryldiazoniums: D. P. Hari, P. Schroll and B. König, J. Am. Chem. Soc., 2012, 134, 2958 CrossRef CAS PubMed.
- D. A. Nicewicz and T. M. Nguyen, ACS Catal., 2013, 4, 355 CrossRef.
- The same reaction carried out in an open flask with non-degassed solvents yielded 51% of 3aa.
- L. M. Anderson, A. R. Butler and A. S. McIntosh, J. Chem. Soc., Perkin Trans. 2, 1987, 1239 RSC.
- T. F. Spande and G. G. Glenner, J. Am. Chem. Soc., 1973, 95, 3400 CrossRef CAS.
- H. Cano-Yelo and A. Deronzier, J. Photochem., 1987, 37, 315 CrossRef CAS.
- Alternative photocatalytic approach: A. C. Hernandez-Perez and S. K. Collins, Angew. Chem., Int. Ed., 2013, 52, 12696 CrossRef CAS PubMed.
- K. K. Laali and M. Shokouhimehr, Curr. Org. Synth., 2009, 6, 193 CrossRef CAS.
- Q. Zhang, Z.-Q. Zhang, Y. Fu and H.-Z. Yu, ACS Catal., 2016, 6, 798 CrossRef CAS.
- A. Tlahuext-Aca, M. N. Hopkinson, C. G. Daniliuc and F. Glorius, Chem.–Eur. J., 2016, 22, 11587 CrossRef CAS PubMed.
- Reductive elimination steps involving Au(III) can be extremely fast, see: W. J. Wolf, M. S. Winston and F. D. Toste, Nat. Chem., 2014, 6, 159 CrossRef CAS PubMed.
- D. Marcoux and A. B. Charette, J. Org. Chem., 2008, 73, 590 CrossRef CAS PubMed.
- P. S. J. Canning, H. Maskill, K. McCrudden and B. Sexton, Bull. Chem. Soc. Jpn., 2002, 75, 789 CrossRef CAS.
Footnote |
† Electronic supplementary information (ESI) available: Experimental procedures, full optimisation tables, control reaction, mechanistic studies, characterisation data and copies of NMR spectra of new compounds. See DOI: 10.1039/c6sc05469b |
|
This journal is © The Royal Society of Chemistry 2017 |