DOI:
10.1039/C6RE00225K
(Communication)
React. Chem. Eng., 2017,
2, 304-308
Continuous flow telescopic oxidation of alcohols via generation of chlorine and hypochlorite†
Received
19th December 2016
, Accepted 15th March 2017
First published on 15th March 2017
Abstract
A 3-step continuous flow oxidation of alcohols is demonstrated with continuous generation of chlorine as the first step followed by its use for the flow synthesis of high strength sodium hypochlorite. The solution is subsequently used for oxidation of alcohols in the presence of a catalytic amount of the nitroxyl radical “TEMPO”, which inhibits oxidation at the aldehyde stage. Selective oxidations of eight different alcohols were demonstrated. To achieve identical yields, the aromatic alcohols containing electron withdrawing groups needed a longer residence time than aliphatic alcohols.
Introduction
Oxidation of organic substrates is typically a bi-phasic or tri-phasic reaction depending on the type of oxidizing agent, where mixing and interfacial mass transfer become major issues. Typically, the use of stoichiometric quantities of inorganic oxidants such as KMnO4, K2Cr2O7, and CrO3 produces toxic waste.1,2 A greener approach is to use molecular oxygen, air, bleach or hydrogen peroxide for selective oxidation of alcohols.3,4 In some cases, supported nanoparticles are also used with molecular oxygen or air. Shi et al.5 used La2O3-supported copper nanoparticles for transfer dehydrogenation of primary aliphatic alcohols to aldehydes with a yield of 63% for the oxidation of 1-octanal and 45% for the oxidation of 1-decanal under a N2 atmosphere over a reaction time of 2 hours and 10 hours, respectively. Dehydrogenation of primary and secondary alcohols to aldehydes using unsupported crystalline Re nanoparticles is also reported to give a moderate to high yield through γ-CH activation.6 Platinum nanoparticles dispersed in an amphiphilic polymer are also used for aerobic flow oxidation of alcohols in water.7
Over the last two decades, the use of (2,2,6,6-tetramethylpiperidin-1-yl)oxyl (TEMPO) based selective oxidation has attracted great attention. It is a heterocyclic stable radical which is soluble in water as well as in organic solvents, and was first discovered by Lebelev & Kazarnowskii (1960). The reactive species, oxoammonium cation, formed by oxidation of TEMPO in the presence of either (i) a secondary oxidant such as hypochlorite at low temperatures such as 0–4 °C under slightly basic conditions (Anelli–Montanari process), or (ii) O2 along with active metal catalysts (Ru2+, Mn2+/Co2+ and Cu+),8 or (iii) a mild electric potential (0.7 V versus Ag/AgCl),9 is reported to selectively oxidize a variety of alcohols. TEMPO is expensive and is used in catalytic quantities (0.1–10 mol%). When used in relatively higher quantities, it can only be recovered by costly azeotropic distillation. Alternatively, reusable polymer supported TEMPO with bleach, molecular oxygen and air is reported to be very effective for selective oxidation of alcohols to aldehydes.4 A silica-immobilized TEMPO catalyst with catalytic amounts of HNO3 as a NOx source is also used as a continuous three-phase flow system with O2.10
Here we have used TEMPO along with freshly prepared bleach (pH adjusted to 9.5) for continuous oxidation. In general, bleach is synthesized by reaction of chlorine gas and sodium hydroxide solution, known as the Hooker process. Chlorine, which is highly reactive, not available on demand, unsafe to store in synthesis labs, and used for the preparation of commercially available sodium hypochlorite solution (usually of low strength), is preferred for such oxidation reactions.11,12 One of the ways to overcome the low strength of sodium hypochlorite is to prepare it fresh, which requires chlorine, and to use it immediately. Recent works by Strauss et al.13 and Fukuyama14 have demonstrated the continuous flow synthesis of chlorine using HCl and NaOCl for chlorination reactions. In an almost parallel activity, here we propose an alternate route for the flow synthesis of chlorine through a liquid–solid reaction of hydrochloric acid and manganese dioxide (Scheme 1). KBr is used to enhance the rate of reaction as the hypobromite anion acts as an active oxidizing agent of nitroxide.
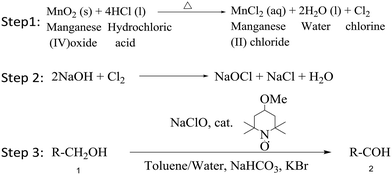 |
| Scheme 1 3-step reaction: 1) chlorine generation, 2) hypochlorite synthesis, 3) alcohol oxidation using the TEMPO catalyst. | |
Experimental
Chlorine generation
70 g MnO2 powder was placed in a 250 mL round bottom flask and heated at 80 °C. To this flask, HCl was continuously added at a fixed flow rate under vigorous stirring. The rate of HCl addition controlled the rate of generation of chlorine. For example, at a 2 mL min−1 flow rate of HCl, the reaction mixture generates Cl2 gas at a flow rate of 51 mL min−1. The gas flow rate was measured using a soap film meter and calibrated for specific inlet flow rates of HCl. The experiments were conducted during the period at which the gas generation rate was within ±1% of the desired flow rate. Every experiment lasted for a maximum of 30 minutes (Fig. 1).
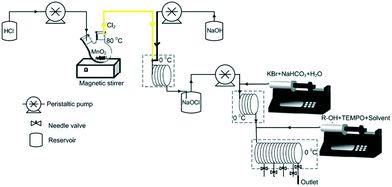 |
| Fig. 1 Schematic of the 3-step continuous flow alcohol oxidation (1.5 equivalents of hypochlorite, pH = 9.5, adjusted by addition of saturated NaHCO3, 5 mol% KBr, and 3 mol% TEMPO). | |
Sodium hypochlorite synthesis
10% NaOH solution was continuously flowed to react with in situ generated Cl2 to yield NaOCl. For this part of the experimental setup, a glass coil (1.5 mm inner diameter and 12 mL total volume) was used. Upon contact with aqueous NaOH, yellow coloured gas was seen to disappear quickly due to the rapid reaction. The generated NaOCl solution (of pH 13.9) was intermittently collected in an amberlite color glass bottle to measure the strength. The strength of the solution was measured through titration with 0.1 M Na2S2O3, using a mixture of 1.5 g of KI, 1 mL glacial acetic acid and 1 mL of water with 1 mL NaOCl. The strength of the hypochlorite solution was found to vary from 7% to 13% depending upon the flow rates of the reactants and the residence time, and it was further adjusted depending upon the need for selective oxidation of alcohol to aldehyde. The rate of formation of the hypochlorite solution was very fast and no chlorine was seen to escape unreacted from the reactor. In the synthesis of sodium hypochlorite, higher concentration of sodium hydroxide, higher flow rate of chlorine along with a longer residence time provides higher strength hypochlorite as more contact time facilitates this exothermic reaction. For example, when compared to a 4 ml reactor tube that gives only 2% solution strength of hypochlorite in 5 s, in a 38 ml reactor (of an identical diameter) the solution strength is 11.52% with a residence time of 36 s. Thus high strength hypochlorite can be synthesized by prolonging the reaction time. However the stability of the solution also depends on the pH.
Alcohol oxidation
As an additional step in the flow synthesis, pH adjustment was carried out to ensure that for a specific alcohol the concentration of the oxidizing agent is controlled. A saturated buffer solution of NaHCO3 and 2 g of KBr dissolved in 120 mL of water was dosed using a syringe pump and mixed together with the NaOCl solution having a pH of 13.9. The flow rate of the buffer solution was adjusted such that the pH of the outlet stream can be varied between 10 and 9.5, which is necessary to retain an adequate strength of the oxidizing agent to avoid over-oxidation. Our analysis showed that a hypochlorite solution of strength between 3.1 and 4.5 was sufficient to achieve controlled oxidation. The third and last part of the experiment was the controlled oxidation of alcohol and this step was performed in a PTFE tube (2 mm inner diameter and 28 ml volume). In the last step, the organic phase comprising a solution of 3 mol% TEMPO catalyst and 3.7 mL alcohol in 30 mL solvent (toluene) was dosed using a separate syringe pump. The pH adjusted NaOCl reacted with the organic solution containing the alcohol. The flow rate of the organic phase was decided based on the concentration of alcohol and the strength of NaOCl in the second stream.
Samples were collected in a quenching solution of Na2S2O3, which decomposed excess NaOCl. The organic phase was separated, washed with water and brine and dried over Na2SO4. The samples were diluted for analysis using gas chromatography (GC). An HP-5 capillary column with a FID detector was used for GC analysis, and bromobenzene was used as an internal standard. The products and by-products were confirmed by GCMS, IR and NMR.
Results and discussion
In oxidation with bleach (hypochlorite), the pH plays an important role and it is necessary to keep it in the range of 9.1 to 9.8. Under highly basic conditions (pH = 13.5), no oxidation reaction takes place, while at acidic pH (<5) the acid is formed as a major product. In order to understand the range of pH that is suitable for the proposed experiments, initially a few batch experiments were performed for the oxidation of 1-octanol with 1.3 mol% TEMPO as a primary oxidant and freshly prepared bleach as a secondary oxidant with the pH adjusted to 9.5. KBr was used in the reaction to enhance the rate of reaction. The experiments carried out at 0 °C for 3 hours resulted in 30% yield of 1-octanal. A longer reaction time resulted in significant over-oxidation. Hence for all further experiments, the pH was maintained at 9.5 and the residence time and temperature were modified to maximize the yield of the respective aldehyde.
The effect of the concentration of TEMPO on the conversion and selectivity of the continuous flow oxidation of 1-octanol was studied. Initially, experiments were carried out without using TEMPO, which resulted in over 98% conversion with only 49% 1-octanal. With the 1 mol% TEMPO catalyst for an identical residence time, the conversion was over 98% while the selectivity to 1-octanal was 88%. Further enhancement in the concentration of TEMPO to 3 mol% resulted in 95% yield of the desired product and over 99% conversion in a residence time of 8.6 minutes (Fig. 2). However in the presence of excess hypochlorite, further oxidation of the aldehyde is unavoidable as in such cases hypochlorite acts as a primary oxidant. Octanoic acid and octyl ester are the major impurities and details in the form of GC mass spectra and NMR are given in the ESI.†
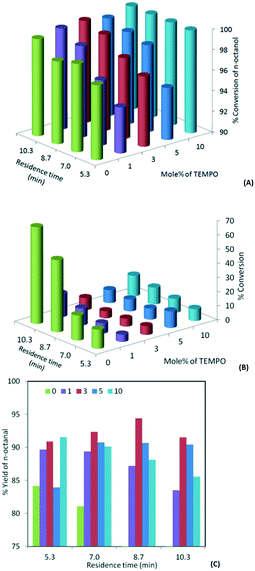 |
| Fig. 2 Effect of TEMPO% and residence time on octanol oxidation. (A) % conversion of n-octanol, (B) % selectivity to impurities (mainly acid and ester) and (C) % yield of the desired aldehyde (yields lower than 75% are not shown here). | |
In the case of oxidation of (trans)-3,7-dimethyl-2,6-octadien-1-ol (geraniol), it gives 2 isomers, neral (2f′) and geranial (2f). Geraniol oxidation was performed with 3, 5 and 10 mol% TEMPO which gave different isomeric ratios of neral and geranial isomers. At a residence time of 6.75 min, with 3 mol% and 10 mol% TEMPO, the yield of neral was 62.4% and 5.7%. However, the yield of geranial did not vary that much. The maximum yield of geranial was 22.3%, which was achieved at a residence time of 6.75 min with 5 mol% TEMPO. Between the two aldehydes, neral showed a higher tendency to decompose with higher TEMPO% (Fig. 3).
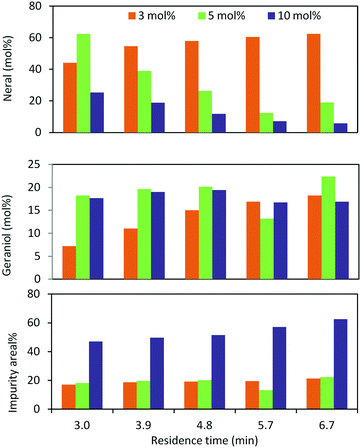 |
| Fig. 3 Effect of TEMPO% and residence time on geraniol. | |
A few more primary (1a–c), secondary (1d and 1f) and aromatic (1e, g and h) alcohols were oxidized using this three step flow approach. The observations are given in Table 1. For primary and secondary alcohols this TEMPO/bleach system works efficiently, giving 80–99% yield of the corresponding aldehydes and ketones. However, for aromatic alcohols, this oxidation strategy needed a longer residence time and still the yield of the respective aldehydes was lower than that of the aliphatic aldehydes. Further work on extending this integrated telescopic approach to the synthesis of a few perfumery aldehydes is in progress.
Table 1 3 mol% TEMPO, 1.5 equivalents of hypochlorite (pH = 9.5, adjusted by addition of saturated NaHCO3) and 5 mol% KBr. [For 1g and 1h, TEMPO is 5 mol% and 7 mol%, respectively]. [For entries 1e and 1h, the yield is based on GC area%]. [%Y – yield of aldehyde, %X – % conversion of alcohol, τ – residence time (min)]
Reactants |
Product |
%Y (%X) |
τ (min) |
|
|
95 (99.3) |
8.7 |
|
|
90 (94.1) |
6.6 |
|
|
95.3 (100) |
5.6 |
|
|
99 (99.2) |
4.9 |
|
|
89 (96.3) |
5.0 |
|
|
80 (100) |
15 |
|
|
99.8 (100) |
4.0 |
|
|
90.1 (99) |
15 |
Conclusions
A three step continuous flow oxidation of alcohols is demonstrated with continuous generation of chlorine as the first step through the reaction of hydrochloric acid and manganese dioxide followed by its use for the flow synthesis of high strength sodium hypochlorite, with continuous pH adjustment. The solution is subsequently used for the oxidation of alcohols in the presence of a catalytic amount of the nitroxyl radical “TEMPO” which inhibits oxidation at the aldehyde stage. Selective oxidations of eight different aliphatic and aromatic alcohols have been demonstrated with high yields in the range of 80–99% for aliphatic alcohols, while aromatic alcohols containing electron withdrawing groups needed a relatively longer residence time for a similar conversion. The approach helps to generate high strength hypochlorite solution that can be used for a variety of reactions. It is necessary to note that for specific oxidation reactions using TEMPO one would need to maintain a specific pH of the solution. This implies that just having high strength hypochlorite solution is not sufficient but the pH needs to be adjusted as well to facilitate the subsequent reaction. Since the approach generates pure chlorine in the gas phase it can be used for efficient chlorination reactions in flow and relevant work will be reported separately.
Acknowledgements
Yachita Sharma thanks CSIR for JRF (CSIR letter No. 31/011/(0726)/2012-EMR-1 dated on 24.02.2012). All the authors gratefully acknowledge the financial support from the Indus Magic Program of CSIR's 12th FYP.
References
- J. R. Sedelmeier, S. V. Ley, I. R. Baxendale and M. Baumann, KMnO4-mediated oxidation as a continuous flow process, Org. Lett., 2010, 12(16), 3618–3621 CrossRef CAS PubMed.
- M. Zhao, J. Li, Z. Song, R. Desmond, D. M. Tschaen, E. J. Grabowski and P. J. Reider, A novel chromium trioxide catalyzed oxidation of primary alcohols to the carboxylic acids, Tetrahedron Lett., 1998, 39(30), 5323–5326 CrossRef CAS.
- L. Vanoye, A. Aloui, M. Pablos, R. Philippe, A. Percheron, A. Favre-Réguillon and C. de Bellefon, A Safe and Efficient Flow Oxidation of Aldehydes with O2, Org. Lett., 2013, 15(23), 5978–5981 CrossRef CAS PubMed.
- M. Gilhespy, M. Lok and X. Baucherel, Polymer-supported nitroxyl radical catalysts for the hypochlorite and aerobic oxidation of alcohols, Catal. Today, 2006, 117(1), 114–119 CrossRef CAS.
- R. Shi, F. Wang, Y. Li, X. Huang and W. Shen, A highly efficient Cu/La2O3 catalyst for transfer dehydrogenation of primary aliphatic alcohols, Green Chem., 2010, 12(1), 108–113 RSC.
- J. Yi, J. T. Miller, D. Y. Zemlyanov, R. Zhang, P. J. Dietrich, F. H. Ribeiro, S. Suslov and M. M. Abu-Omar, A Reusable Unsupported Rhenium Nanocrystalline Catalyst for Acceptorless Dehydrogenation of Alcohols through γ-C–H Activation, Angew. Chem., 2014, 126(3), 852–855 CrossRef.
- T. Osako, K. Torii and Y. Uozumi, Aerobic flow oxidation of alcohols in water catalyzed by platinum nanoparticles dispersed in an amphiphilic polymer, RSC Adv., 2015, 5(4), 2647–2654 RSC.
- R. A. Sheldon and I. W. Arends, Organocatalytic oxidations mediated by nitroxyl radicals, Adv. Synth. Catal., 2004, 346(9–10), 1051–1071 CrossRef CAS.
- A. E. De Nooy, A. C. Besemer and H. van Bekkum, On the use of stable organic nitroxyl radicals for the oxidation of primary and secondary alcohols, Synthesis, 1996, 1996(10), 1153–1176 CrossRef.
- C. Aellig, D. Scholz, S. Conrad and I. Hermans, Intensification of TEMPO-mediated aerobic alcohol oxidations under three-phase flow conditions, Green Chem., 2013, 15(7), 1975–1980 RSC.
- R. Jones, B. Wills and C. Kang, Chlorine gas: an evolving hazardous material threat and unconventional weapon, West. J. Emerg. Med., 2010, 11(2), 151–156 Search PubMed.
- C. W. White and J. G. Martin, Chlorine gas inhalation: human clinical evidence of toxicity and experience in animal models, Proc. Am. Thorac. Soc., 2010, 7(4), 257–263 CrossRef CAS PubMed.
- F. J. Strauss, D. Cantillo, J. Guerra and C. O. Kappe, A laboratory-scale continuous flow chlorine generator for organic synthesis, React. Chem. Eng., 2016, 417–476 Search PubMed.
- T. Fukuyama, M. Tokizane, A. Matsui and I. Ryu, A greener process for flow C–H chlorination of cyclic alkanes using in situ generation and on-site consumption of chlorine gas, React. Chem. Eng., 2016, 613–615 CAS.
Footnote |
† Electronic supplementary information (ESI) available. See DOI: 10.1039/c6re00225k |
|
This journal is © The Royal Society of Chemistry 2017 |
Click here to see how this site uses Cookies. View our privacy policy here.