DOI:
10.1039/C7RA10819B
(Paper)
RSC Adv., 2017,
7, 54013-54016
Metal-free direct thiocarbamation of imidazopyridines with carbamoyl chloride and elemental sulfur†
Received
30th September 2017
, Accepted 18th November 2017
First published on 23rd November 2017
Abstract
The combination of elemental sulfur and carbamoyl chloride was found to act as a carbamothioyl group surrogate for direct thiocarbamation of imidazopyridines in the absence of a metal catalyst.
Thiocarbamates are a class of important biologically active molecules, being widely used as herbicides (e.g. thiobencarb, orbencarb, molinate), fungicides, pesticides, and antiviral agents.1 Traditional methods for the synthesis of thiocarbamates mainly involve the reaction of thiols with carbamoyl chloride, or the nucleophilic addition of isocyanates with thiols.2 Iodine-catalyzed reaction of isocyanides with thiosulfonates is also an effective pathway to thiocarbamates.3 Transition metal-catalyzed or metal-free carbonylation of carbon monoxide with thiols and amines is an alternative to access thiocarbamates.4,5 Although odorous thiols are effective for the synthesis of thiocarbamates, their toxicity and instability are still a long-standing problem. The development of benign agents and straightforward pathways for the synthesis of thiocarbamates is greatly desired. Direct thiocarbamation of imidazopyridines is of great interest because imidazopyridines are important drug skeletons possessing a broad range of biological activities.6,7 Great efforts have been devoted to the thiolation of imidazopyridines using thiols or disulphides.8,9 In 2016, our group also developed a I2/FeF3 catalyzed direct dithiocarbamation of imidazoheterocycles with tetramethylthiuram disulphide.9 However, no example for the direct mono-thiocarbamation of heterocycles is reported. The C–H thiocarbamation reactions remains to be explored. The facing challenge is that thiocarbamating agents are not available for such transformation. The development of carbamothioyl group surrogates for the direct thiocarbamation of imidazopyridines is a solution to this problem. As shown in Scheme 1, three possible pathways for retrosynthesis of imidazopyridines-thiocarbamates are given. In path 1, carbamothioic S-acid is extremely unstable and commercially unavailable. In path 2, 2-phenylimidazo[1,2-a]pyridine-3-thiol is not easily prepared. We envisaged that whether the combination of elemental sulfur and carbamoyl chloride could play as carbamothioyl groups surrogate for the thiocarbamation of imidazopyridines (path 3). This one-pot three-component thiocarbamation reaction is greatly desired. Because these substrates are commercially available and readily prepared, particularly the elemental sulfur is an eco-friendly agent for the synthesis of sulfur-containing compounds.10–12 Very recently, our group also developed a metal-free oxidative dual C–H thiolation of imidazopyridines with inert alkanes or ethers using elemental sulfur.12a This research progress encourages us to develop a metal-free direct thiocarbamation of imidazopyridines using the combination of elemental sulfur and carbamoyl chloride as carbamothioyl groups surrogate (Scheme 1, path 3).
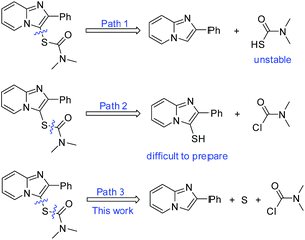 |
| Scheme 1 Retrosynthesis of imidazopyridines-thiocarbamates. | |
Our studies began with the three-component thiolation of imidazopyridine 1a with elemental sulfur and dimethylcarbamoyl chloride. The reaction proceeded with two equivalents of DABCO in DCE at 120 °C for 24 hours, affording product 3 in 26% yield (Table 1, entry 1). Other solvents, such as DMSO, DMF, toluene, dioxane, and CH3CN, were also investigated (Table 1, entries 2–6). Solvent effect has great influence on the yield. No product was found when the reaction was conducted in DMSO or toluene (Table 1, entries 2 and 4). The reaction in DMF only gave product 3 in 9% yield (Table 1, entry 3). In the reaction using DMSO as the solvent, 1,1,3,3-tetramethylurea was observed by GC-MS analysis. It suggests that carbamoyl chloride is unstable in DMSO at 120 °C, which may be the reason for no product formation. In other poor reactions, most of the starting materials 1a was recovered (Table 1, entries 3–5). These results demonstrate that high polar (e.g. DMSO and DMF) and low polar solvents (e.g. toluene) are not suitable for this transformation under the same conditions. Interestingly, CH3CN was found to be effective for this reaction, giving the desired product 3 in 78% yield (Table 1, entry 6). The suitable polarity of CH3CN may facilitate the bonds cleavage of S8 and stabilize reaction intermediates to promote the thiocarbamation. The reaction depends on not only the solvent effect but also the reaction temperature. Decreasing the temperature resulted in a sharp decrease in yield, e.g. 65% yield at 100 °C, 33% yield at 80 °C (Table 1, entries 7 and 8). Other bases including Et3N, Bu3N, pyridine, DMAP, KOH, and K2CO3 were examined, but these bases were less effective than DABCO (Table 1, entries 9–14). Reducing the loading of DABCO led to a decrease in the yield (entries 15 and 16). When DABCO was reduced to 1 or 0.5 equivalent, the yield was decreased to 72% and 68%, respectively. In the absence of DABCO, the reaction still occurred in CH3CN at 120 °C, affording the product 3 in 48% yield (Table 1, entry 17). DABCO used here may play as an acid-binding agent to facilitate the transformation, because the in situ formed hydrogen chloride may suppress the reaction.
Table 1 Screening of optimal conditionsa
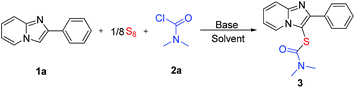
|
Entry |
Solvent |
Base (eq.) |
Temp (°C) |
Yield (%) |
Reaction conditions: 1a (0.2 mmol), S8 (0.4 mmol, 2 equiv.), 2a (0.4 mmol, 2 equiv.), base, solvent (2 mL) at 120 °C for 24 h. DABCO = 1,4-diazabicyclo[2.2.2]octane, DMAP = 4-dimethylaminopyridine. |
1 |
DCE |
DABCO (2) |
120 |
26 |
2 |
DMSO |
DABCO (2) |
120 |
0 |
3 |
DMF |
DABCO (2) |
120 |
9 |
4 |
Toluene |
DABCO (2) |
120 |
0 |
5 |
Dioxane |
DABCO (2) |
120 |
3 |
6 |
CH3CN |
DABCO (2) |
120 |
78 |
7 |
CH3CN |
DABCO (2) |
100 |
65 |
8 |
CH3CN |
DABCO (2) |
80 |
33 |
9 |
CH3CN |
Et3N (2) |
120 |
55 |
10 |
CH3CN |
Bu3N (2) |
120 |
61 |
11 |
CH3CN |
Pyridine (2) |
120 |
65 |
12 |
CH3CN |
DMAP (2) |
120 |
68 |
13 |
CH3CN |
KOH (2) |
120 |
32 |
14 |
CH3CN |
K2CO3 (2) |
120 |
27 |
15 |
CH3CN |
DABCO (1) |
120 |
72 |
16 |
CH3CN |
DABCO (0.5) |
120 |
68 |
17 |
CH3CN |
— |
120 |
48 |
With this thiocarbamation procedure in hand, the imidazopyridine scope was investigated (Table 2). A variety of imidazopyridines were subjected to the reaction with elemental sulfur and carbamoyl chloride (products 3–15). Substrates bearing a methyl, methoxy, or fluoro group were well tolerated affording the corresponding products in moderate to good yields (products 4–7). Substrates bearing two substituents also have good reactivity under reaction conditions (products 8–15). For example, the substrate bearing a methyl and a cyano groups gave product 13 in 70% yield, and the substrate bearing a methoxy and a chloro groups afforded product 14 in 78% yield. To our delight, pyrrolidine-1-carbonyl chloride also underwent the thiocarbamation smoothly to afford their corresponding products 16–22 in moderate yields. Functional groups, including methoxy, fluoro, chloro, and cyano groups, were well tolerated in the reaction. However, imidazothiazoles are less effective than imidazopyridines, affording poor yields under reaction conditions (products 23 and 24). The reaction may depend on the electronic effect of the imidazole ring. The electron-rich substrate facilitates the reaction. Both 2-ethylimidazo[1,2-a]pyridine and 2-ethylimidazo[2,1-b]thiazole are not suitable substrates for such transformation. These results demonstrate that the conjugated effect between the imidazole ring and the benzene ring may be a key factor to the reactivity. The electronic effect of substituent on the benzene ring has influence on the yield to some extent. Electron-donating groups gave higher yields than electron-withdrawing ones (e.g. product 10 vs. product 13, product 18 vs. product 22, product 20 vs. product 21).
Table 2 Direct thiocarbamation of imidazopyridinesa
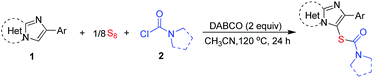
|
Reaction conditions: 1 (0.2 mmol), 2 (0.4 mmol, 2 equiv.), S8 (2 equiv.), DBACO (2 equiv.), in CH3CN (2 mL) at 120 °C for 24 h. |
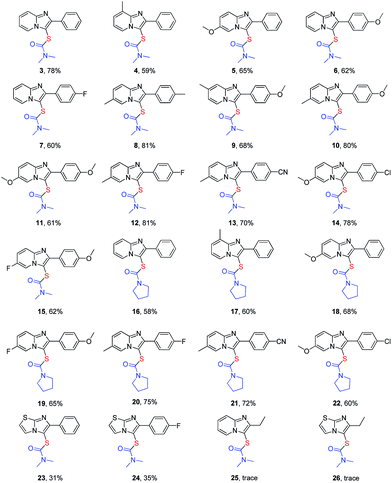 |
In order to elucidate the reaction mechanism, the reaction of imidazopyridine 1a with S8 was conducted in the absence of carbamoyl chloride. LC-MS analysis showed that a small amount of di-imidazopyridinyl sulfide and di-imidazopyridinyl disulfide are formed under reaction conditions. Based on present results and previous reports concerning the thiolation using elemental sulfur,12 a possible mechanism has been proposed as shown in Scheme 2. Under heating conditions, imidazopyridine 1a may has a resonance structure A, which undergoes a nucleophilic reaction with S8 to produce intermediate B (Scheme 2, eqn (1)). The polysulfide intermediate B reacts with A to provide intermediate C (Scheme 2, eqn (2)). Finally, the newly formed intermediate C undergoes a thioesterification with carbamoyl chloride to provide imidazopyridines-thiocarbamates (Scheme 2, eqn (3)).
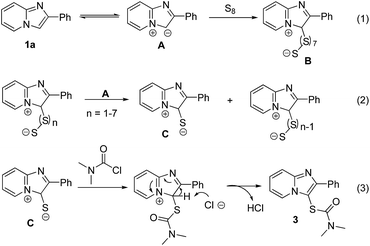 |
| Scheme 2 A possible reaction mechanism. | |
Conclusion
In summary, the combination of elemental sulfur and carbamoyl chloride has been developed as the carbamothioyl groups surrogate for direct thiocarbamation of imidazopyridines. This metal-free three-component thiocarbamation reaction proceeds well in the presence of DABCO, giving their corresponding products in moderate to good yields. It is noteworthy that elemental sulfur is an inexpensive, nontoxic, odorless, stable and easy to handle powder; elemental sulfur also allows for an efficient synthesis of sulfur-containing compounds in terms of atom economy. These newly formed imidazopyridines-thiocarbamates may have significantly biological activity, which will be of great interest in medicinal chemistry. The direct thiocarbamation of heterocycles with elemental sulfur and formamide is underway in our lab.
Conflicts of interest
There are no conflicts to declare.
Acknowledgements
We gratefully thank the NSFC (No. 21202121), Science Technology Program Project of Guangdong Province (No. 2016B020204005) and Guangzhou Science Technology Program Project (No. 201607010142) for their financial support.
Notes and references
-
(a) B. Lindgren, G. Lindgren, E. Artursson, G. Puu, J. Fredriksson and M. Andersson, J. Enzyme Inhib., 1985, 1, 1–11 CrossRef CAS PubMed;
(b) K. Ishikawa, I. Okuda and S. Kuwatsuka, Agric. Biol. Chem., 1973, 37, 165–173 CrossRef CAS;
(c) J. Kochansky and C. F. Cohen, J. Agric. Entomol., 1990, 7, 293–304 CAS;
(d) A. Goel, S. J. Mazur, R. J. Fattah, T. L. Hartman, J. A. Turpin, M. Huang, W. G. Rice, E. Appella and J. K. Inman, Bioorg. Med. Chem. Lett., 2002, 12, 767–770 CrossRef CAS PubMed.
-
(a) H. K. Kim and A. Lee, Tetrahedron Lett., 2016, 57, 4890–4892 CrossRef CAS;
(b) H. K. Kim and A. Lee, Org. Biomol. Chem., 2016, 14, 7345–7353 RSC;
(c) M. I. Takumi and S. Noboru, Tetrahedron Lett., 1994, 50, 5669–5680 CrossRef.
- P. Mampuys, Y. P. Zhu, S. Sergeyev, E. Ruijter, R. Orru and S. Van Doorslaer, Org. Lett., 2016, 18, 2808–2811 CrossRef CAS PubMed.
-
(a) W. D. Jones, K. A. Reynolds, C. K. Sperry and R. J. Lachicotte, Organometallics, 2000, 19, 1661–1669 CrossRef CAS;
(b) J. Jacob, K. A. Reynolds, W. D. Jones, S. A. Godleski and R. R. Valente, Organometallics, 2001, 20, 1028–1031 CrossRef CAS;
(c) Y. A. Nishiyama and N. Sonoda, J. Org. Chem., 2005, 70, 2551–2554 CrossRef CAS PubMed.
-
(a) T. Mizuno, T. Iwai and T. Ito, Tetrahedron, 2004, 60, 2869–2873 CrossRef CAS;
(b) T. Mizuno, T. Iwai and Y. Ishino, Tetrahedron, 2005, 61, 9157–9163 CrossRef CAS;
(c) X. P. Zhang and S. W. Lu, Chem. Lett., 2005, 34, 606–607 CrossRef CAS.
- E. G. Cecile and G. Alain, Mini-Rev. Med. Chem., 2007, 7, 888–899 CrossRef.
- J. Koubachi, S. E. Kazzouli, M. Bousmina and G. Guillaumet, Eur. J. Org. Chem., 2014, 2014, 5119–5138 CrossRef CAS.
- For selected references, see:
(a) Y. Siddaraju and K. R. Prabhu, J. Org. Chem., 2016, 81, 7838–7846 CrossRef CAS PubMed;
(b) J. Rafique, S. Saba, A. R. Rosario and A. L. Braga, Chem.–Eur. J., 2016, 22, 11854–11862 CrossRef CAS PubMed;
(c) X. M. Ji, S. J. Zhou, F. Chen, X. G. Zhang and R. Y. Tang, Synthesis, 2015, 47, 659–671 CrossRef CAS;
(d) M. A. Hiebel and S. Berteina Raboin, Green Chem., 2015, 17, 937–944 RSC;
(e) C. Ravi, D. Chandra Mohan and S. Adimurthy, Org. Lett., 2014, 16, 2978–2981 CrossRef CAS PubMed;
(f) S. Mitra, M. Ghosh, S. Mishra and A. Hajra, J. Org. Chem., 2015, 80, 8275–8281 CrossRef CAS PubMed;
(g) D. Yang, K. Yan, W. Wei, G. Li, S. Lu, C. Zhao, L. Tian and H. Wang, J. Org. Chem., 2015, 80, 11073–11079 CrossRef CAS PubMed;
(h) Q. Wang, Z. Qi, F. Xie and X. Li, Adv. Synth. Catal., 2015, 357, 355–360 CrossRef CAS.
- J. Jiao, L. Wei, X.-M. Ji, M.-L. Hu and R.-Y. Tang, Adv. Synth. Catal., 2016, 358, 268–275 CrossRef CAS.
- Metal-catalyzed thiolation using elemental sulphur, see:
(a) F. J. Chen, G. Liao, X. Li, J. Wu and B. F. Shi, Org. Lett., 2014, 16, 5644–5647 CrossRef CAS PubMed;
(b) R. Han and G. L. Hillhouse, J. Am. Chem. Soc., 1998, 120, 7657–7658 CrossRef CAS;
(c) C. Chen, L. Chu and F. L. Qing, J. Am. Chem. Soc., 2012, 134, 12454–12457 CrossRef CAS PubMed;
(d) Z. Zhou, Y. Liu, J. Chen, E. Yao and J. Cheng, Org. Lett., 2016, 18, 5268–5271 CrossRef CAS PubMed;
(e) A. S. Reddy and K. C. Kumara Swamy, Org. Lett., 2015, 17, 2996–2999 CrossRef PubMed;
(f) M. Arisawa, T. Ichikawa and M. Yamaguchi, Org. Lett., 2012, 14, 5318–5321 CrossRef CAS PubMed;
(g) C. Chen, Y. Xie, L. Chu, R. W. Wang, X. Zhang and F. L. Qing, Angew. Chem., Int. Ed., 2012, 51, 2492–2495 CrossRef CAS PubMed;
(h) Y. Huang, X. He, X. Lin, M. Rong and Z. Weng, Org. Lett., 2014, 16, 3284–3287 CrossRef CAS PubMed;
(i) F. Shibahara, T. Kanai, E. Yamaguchi, A. Kamei, T. Yamauchi and T. Murai, Chem.–Asian J., 2014, 9, 237–244 CrossRef CAS PubMed;
(j) Z. Weng, W. He, C. Chen, R. Lee, D. Tan, Z. Lai, D. Kong, Y. Yuan and K.-W. Huang, Angew. Chem., Int. Ed., 2013, 52, 1548–1552 CrossRef CAS PubMed;
(k) F. Xiao, S. Chen, C. Li, H. Huang and G.-J. Deng, Adv. Synth. Catal., 2016, 358, 3881–3886 CrossRef CAS.
- Metal-free thiolation using elemental sulphur, see:
(a) G. Zhang, H. Yi, H. Chen, C. Bian, C. Liu and A. Lei, Org. Lett., 2014, 16, 6156–6159 CrossRef CAS PubMed;
(b) Z. Wang, Y. Wang, W. X. Zhang, Z. Hou and Z. Xi, J. Am. Chem. Soc., 2009, 131, 15108–15109 CrossRef CAS PubMed;
(c) T. B. Nguyen, L. Ermolenko and A. Al-Mourabit, Org. Lett., 2013, 15, 4218–4221 CrossRef CAS PubMed;
(d) M. Wang, Q. Fan and X. Jiang, Org. Lett., 2016, 18, 5756–5759 CrossRef CAS PubMed.
- Thiolation of imidazopyridines using elemental sulphur, see:
(a) J. R. Zhang, Y. Y. Liao, J. C. Deng, K. Y. Feng, M. Zhang, Y. Y. Ning, Z. W. Lin and R. Y. Tang, Chem. Commun., 2017, 53, 7784–7787 RSC;
(b) J. Li, C. Li, S. Yang, Y. An, W. Wu and H. Jiang, J. Org. Chem., 2016, 81, 7771–7783 CrossRef CAS PubMed;
(c) C. Ravi, N. N. K. Reddy, V. Pappula, S. Samanta and S. Adimurthy, J. Org. Chem., 2016, 81, 9964–9972 CrossRef CAS PubMed.
Footnotes |
† Electronic supplementary information (ESI) available. See DOI: 10.1039/c7ra10819b |
‡ Equal contribution to this work. |
|
This journal is © The Royal Society of Chemistry 2017 |