DOI:
10.1039/C7RA09057A
(Paper)
RSC Adv., 2017,
7, 45416-45419
K2CO3-promoted aerobic oxidative cross-coupling of trialkyl phosphites with thiophenols†
Received
16th August 2017
, Accepted 18th September 2017
First published on 25th September 2017
Abstract
Convenient, practical and economical phosphorylation of thiols has been achieved via halogen- and metal-free K2CO3-promoted aerobic oxidative cross-coupling of trialkyl phosphites, dimethyl phenylphosphonite, or methyl diphenylphosphinite with thiophenols using air as the oxidant at room temperature. This transformation provides a straightforward route to the construction of phosphorus–sulfur bonds with wide functional group compatibility, which affords phosphorothioates in up to 94% yield.
In recent years, the development of new methods to construct phosphorus–sulfur bonds has been of particular interest due to the wide applications of organophosphorus–sulfur compounds in biological chemistry, organic synthesis, and agrochemistry.1 A variety of useful synthetic methods have been well documented. The traditional preparation of this class of compounds mainly relies on the use of toxic and moisture sensitive reagents or pre-functionalized substrates (e.g., R2P(O)X, RSX and RSSR).2 On the other hand, the cross-dehydrogenative coupling (CDC) between P(O)H compounds and commercially available thiols is gradually developed (Fig. 1, eqn (1)).3–7 In 2013, Kaboudin reported a copper-catalyzed coupling of H-phosphonates with thiols in the presence of Et3N.3 In 2015, Pan reported a TBPB-promoted oxidative coupling of secondary phosphine oxides or H-phosphonates with thiols.4 In 2016, Li and Zhang reported a visible-light-mediated oxidative coupling of P(O)H compounds with thiols in the presence of a photocatalyst.5 Interestingly, Chen and Han recently reported an oxidant-free Pd-catalyzed dehydrogenative phosphorylation of thiols.6 Most recently, Song and Jiao reported a Cs2CO3-catalyzed aerobic oxidative CDC reaction of phosphonates with thiols.7 The possible mechanism showed that disulfides are reaction intermediates generated via the oxidative coupling of thiols in the presence of Cs2CO3 and O2.7 Undoubtedly, this strategy represents more straightforward, efficient, and atom-economic to construct phosphorus–sulfur bonds. However, the CDC reactions suffered from limitations with regard to high cost and less availability of P(O)H compounds. Thus, the development of a convenient, practical and economical protocol for the synthesis of phosphorothioates is still a significant issue.
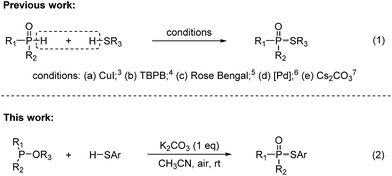 |
| Fig. 1 Current developments in the synthesis of phosphorothioates from phosphites and thiols. | |
With our recent studies on the construction of carbon–sulfur, phosphorus–aryl, phosphorus–fluorine, and phosphorus–oxygen bonds,8 we have been interested in studying the construction of phosphorus–sulfur bonds based on an economical approach. In comparison with P(O)H compounds, trialkyl phosphites are relatively inexpensive and readily available as phosphorus nucleophiles, which have also been widely used for the synthesis of various organophosphorus compounds.9 In consideration of searching phosphorus nucleophiles instead of P(O)H compounds, we envision that the interaction between trialkyl phosphites and thiols might produce phosphorothioates via the elimination of an alcohol. Herein, we report an alternative approach to the synthesis of phosphorothioates based on K2CO3-promoted aerobic oxidative cross-coupling of trialkyl phosphites with thiophenols using air as the oxidant at room temperature.
According to the reaction conditions of the CDC reactions between dialkyl phosphites and thiols developed by Song and Jiao,7 our hypothesis was tested by using a model reaction of triethyl phosphite 1a with p-toluenethiol 2a in solvents under air atmosphere at 40 °C, and the results were shown in Table 1. Initially, the reaction of 1a with 2a in CH3CN under air in the absence of bases gave only trace amounts of the desired coupling product 3aa (entry 1). When Cs2CO3 (1 equiv.) was added, the reaction proceeded smoothly to afford 3aa in 68% yield, while the oxidation product 4a was also obtained in 24% yield (entry 2). When the reaction was carried out under N2, only trace amounts of 3aa were detected (entry 3). This result demonstrates that this reaction involved an aerobic oxidative cross-coupling. Switching the solvent from CH3CN to THF, dioxane, or DMSO decreased the yield of 3aa (entries 4–6). We then turned to screen other bases (entries 7–10). To our delight, the use of cheaper base K2CO3 gave 3aa in excellent yield (98%, entry 7), while the yield of the side product 4a was significantly decreased. We then carried out the reaction at room temperature, the yield of 3aa was only slightly decreased (97%, entry 11). The catalytic efficiency of K2CO3 was then tested. When 0.5 or 0.1 equiv. of K2CO3 was introduced, 3aa was still obtained in 87% or 81% yield (entries 12 and 13). It is noteworthy that disulfide 5a, which was generated via an aerobic oxidative homocoupling of thiol 2a,10 was observed in all cases (in the presence of bases and air). Finally, we concluded that the optimized combination for the cross-coupling reaction of trialkyl phosphites with thiols was to use 1 equiv. of K2CO3 as the base, CH3CN as the solvent, and the reaction was set at room temperature under air atmosphere (entry 11).
Table 1 Optimization of reaction conditionsa,b

|
Entry |
Base (equiv.) |
Solvent |
T (°C) |
Yield 3aa (%) |
Yield 4a (%) |
Reaction conditions: 1a (0.20 mmol), 2a (0.46 mmol), base, solvent (1 mL), open air, 5 h. Yield based on 1a was determined by 31P NMR analysis of crude products using an internal standard. The reaction was carried out under N2. |
1 |
|
CH3CN |
40 |
Trace |
Trace |
2 |
Cs2CO3 (1) |
CH3CN |
40 |
68 |
24 |
3c |
Cs2CO3 (1) |
CH3CN |
40 |
Trace |
Trace |
4 |
Cs2CO3 (1) |
THF |
40 |
18 |
3 |
5 |
Cs2CO3 (1) |
Dioxane |
40 |
28 |
4 |
6 |
Cs2CO3 (1) |
DMSO |
40 |
Trace |
Trace |
7 |
K2CO3 (1) |
CH3CN |
40 |
98 |
1 |
8 |
Na2CO3 (1) |
CH3CN |
40 |
71 |
16 |
9 |
K3PO4 (1) |
CH3CN |
40 |
55 |
14 |
10 |
Et3N (1) |
CH3CN |
40 |
82 |
8 |
11 |
K2CO3 (1) |
CH3CN |
rt |
97 |
1 |
12 |
K2CO3 (0.5) |
CH3CN |
rt |
87 |
8 |
13 |
K2CO3 (0.1) |
CH3CN |
rt |
81 |
12 |
We then set out to explore the generality of the cross-coupling of trialkyl phosphites with thiols. We first applied the optimized conditions to the coupling of triethyl phosphite 1a with a variety of thiols 2, and the results are illustrated in Table 2. The results showed that thiophenol substrates bearing different groups such as alkyl groups, OMe, NH2, Br, Cl, F, CF3, and NO2 at para, ortho or meta or at both positions of aromatic rings, as well as 2-naphthalenethiol and thiophene-2-thiol, were all well tolerated. The corresponding products 3aa–3ax were isolated in moderate to high yields, indicating that the electronic and steric effects were not evident in this reaction. However, the phosphorylation of aliphatic thiols such as benzylthiol and cyclohexylthiol failed to give the desired 3ay or 3az. The scale-up of the reaction of 1a with 2a was also attempted. When we increased the scale of the reaction from 0.4 to 4 mmol, 3aa was also isolated in good yield (65%).
Table 2 Scope of thiolsa,b

|
Reaction conditions: 1a (0.40 mmol), 2 (0.92 mmol), and K2CO3 (0.40 mmol) in CH3CN (2 mL) stirring at room temperature under air for 5–12 h. Isolated yield based on 1a. The reaction was performed in a 4 mmol scale. |
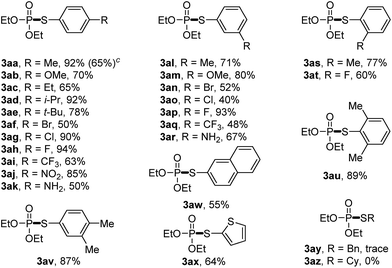 |
We then turned to explore the generality of the cross-coupling of trialkyl phosphites and their derivatives with p-toluenethiol 2a and/or p-chlorobenzenethiol 2g under the optimized conditions, and the results were shown in Table 3. Trimethyl phosphite 1b afforded the desired 3ba and 3bg in low yields probably due to its relatively weak nucleophilicity, while triisopropyl phosphite 1c and tributyl phosphite 1d smoothly gave the desired 3ca, 3cg, 3da, and 3dg in high yields. However, triphenyl phosphite 1e failed to give the coupling product 3ea. In addition, the cross-coupling of P(III) compounds bearing one or two methoxy substituents were also attempted. Pleasingly, the protocol was found to work well when dimethyl phenylphosphonite 1f or methyl diphenylphosphinite 1g was employed as the phosphorus nucleophile. The corresponding coupling products 3fa and 3ga were isolated in 45% and 55% yields, respectively. Notably, the side products 4 and disulfides 5 were detected in most cases of Tables 2 and 3.
Table 3 Scope of P(III) compoundsa,b
The above experimental results (Table 1, entries 1 and 3) showed that both bases and air are indispensable for the cross-coupling reaction. To gain more insight into the mechanism of this reaction, a couple of control experiments were then conducted (Scheme 1). In consideration of the generation of disulfides in all cases, the reaction of thiol 2a with K2CO3 under air was carried out, leading to the formation of disulfide 5a in quantitative yield (eqn (1)), which suggests that K2CO3 might increase the oxidation rate of thiols with dioxygen.11,12 In addition, the reaction of 1a with disulfide 5a under air or N2 in the absence of K2CO3 also gave 3aa in high yield (eqn (2) and (3)). The above results suggest that disulfide might be an intermediate in the cross-coupling reaction.
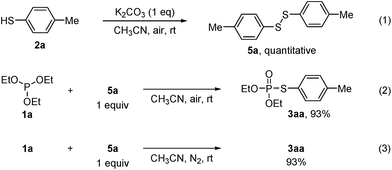 |
| Scheme 1 Mechanistic studies. | |
According to the literatures and our observations, together with the generation of side products 4, a plausible reaction mechanism is outlined in Scheme 2. Initially, thiyl radical is generated from a single electron oxidation of thiol 2 in the presence of dioxygen, followed by the loss of the proton with the assistance of K2CO3. Thiyl radical could undergo homocoupling to produce disulfide 5.7,11–13 Then the nucleophilic attack of P(III) compound 1 on disulfide 5 affords phosphonium cation 6, which reacts with water to form the unstable intermediate 7. Subsequent alcohol elimination of 7 gives the desired phosphorothioate 3 (path a), whereas the thiophenol elimination affords the oxidation product 4 (path b).
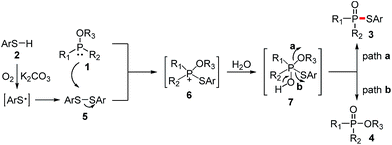 |
| Scheme 2 Proposed mechanism. | |
Conclusions
In conclusion, we have developed the K2CO3-promoted aerobic oxidative cross-coupling of trialkyl phosphites, dimethyl phenylphosphonite, or methyl diphenylphosphinite with thiophenols, which provides a convenient, practical and economical protocol for the synthesis of phosphorothioates with wide functional group compatibility. We envision that the reaction mode outlined here will have potential applications in organic synthesis. Further studies on the transformations of thiols with electrophiles are ongoing and will be reported in due course.
Conflicts of interest
There are no conflicts to declare.
Acknowledgements
This work was supported by the Science and Technology Planning Project of Guangdong Province (No. 2015A020211026 and 2017A010103044), 100 Young Talents Programme of Guangdong University of Technology (220413506), and the Open Fund of the Key Laboratory of Functional Molecular Engineering of Guangdong Province (2016kf07, South China University of Technology).
Notes and references
-
(a) N. N. Melnikov, Chemistry of Pesticides, Springer-Verlag, New York, 1971 Search PubMed;
(b) L. D. Quin, A Guide to Organophosphorus Chemistry, Wiley Interscience, New York, 2000 Search PubMed;
(c) P. J. Murphy, Organophosphorus Reagents, Oxford University Press, Oxford, UK, 2004 Search PubMed;
(d) N.-S. Li, J. K. Frederiksen and J. A. Piccirilli, Acc. Chem. Res., 2011, 44, 1257 CrossRef CAS PubMed;
(e) J. F. Milligan and O. C. Uhlenbeck, Biochemistry, 1989, 28, 2849 CrossRef CAS PubMed;
(f) S. H. Vollmer, M. B. Walner, K. V. Tarbell and R. F. Colman, J. Biol. Chem., 1994, 269, 8082 CAS;
(g) S. Cogoi, V. Rapozzi, F. Quadrifoglio and L. Xodo, Biochemistry, 2001, 40, 1135 CrossRef CAS PubMed;
(h) G. G. Durgam, T. Virag, M. D. Walker, R. Tsukahara, S. Yasuda, K. Liliom, L. A. van Meeteren, W. H. Moolenaar, N. Wilke, W. Siess, G. Tigyi and D. D. Miller, J. Med. Chem., 2005, 48, 4919 CrossRef CAS PubMed;
(i) P. Carta, N. Puljic, C. Robert, A.-L. Dhimane, L. Fensterbank, E. Lacôte and M. Malacria, Org. Lett., 2007, 9, 1061 CrossRef CAS PubMed;
(j) M. Piekutowska and Z. Pakulski, Carbohydr. Res., 2008, 343, 785 CrossRef CAS PubMed;
(k) V. K. Pandey, A. Dwivedi, O. P. Pandey and S. K. Sengupta, J. Agric. Food Chem., 2008, 56, 10779 CrossRef CAS PubMed;
(l) T. Ruman, K. Długopolska, A. Jurkiewicz, D. Rut, T. Frączyk, J. Cieśla, A. Leś, Z. Szewczuk and W. Rode, Bioorg. Chem., 2010, 38, 74 CrossRef CAS PubMed;
(m) A. M. Lauer, F. Mahmud and J. Wu, J. Am. Chem. Soc., 2011, 133, 9119 CrossRef CAS PubMed;
(n) A. M. Lauer and J. Wu, Org. Lett., 2012, 14, 5138 CrossRef CAS PubMed;
(o) M. W. Loranger, S. A. Beaton, K. L. Lines and D. L. Jakeman, Carbohydr. Res., 2013, 379, 43 CrossRef CAS PubMed;
(p) T. S. Kumar, T. Yang, S. Mishra, C. Cronin, S. Chakraborty, J.-B. Shen, B. T. Liang and K. A. Jacobson, J. Med. Chem., 2013, 56, 902 CrossRef CAS PubMed;
(q) Y. Qiu, J. C. Worch, D. N. Chirdon, A. Kaur, A. B. Maurer, S. Amsterdam, C. R. Collins, T. Pintauer, D. Yaron, S. Bernhard and K. J. T. Noonan, Chem.–Eur. J., 2014, 20, 7746 CrossRef CAS PubMed.
-
(a) F. R. Atherton and A. R. Todd, J. Chem. Soc., 1947, 674 RSC;
(b) J. Michalski and J. Wasiak, J. Chem. Soc., 1962, 5056 RSC;
(c) R. G. Harvey, H. I. Jacobson and E. V. Jensen, J. Am. Chem. Soc., 1963, 85, 1618 CrossRef CAS;
(d) P.-Y. Renard, H. Schwebel, P. Vayron, L. Josien, A. Valleix and C. Mioskowski, Chem.–Eur. J., 2002, 8, 2910 CrossRef CAS PubMed;
(e) B. Kaboudin, Tetrahedron Lett., 2002, 43, 8713 CrossRef CAS;
(f) J.-D. Ye, C. D. Barth, P. S. R. Anjaneyulu, T. Tuschl and J. A. Piccirilli, Org. Biomol. Chem., 2007, 5, 2491 RSC;
(g) Y.-X. Gao, G. Tang, Y. Cao and Y.-F. Zhao, Synthesis, 2009, 1081 Search PubMed;
(h) G. Wang, R. Shen, Q. Xu, M. Goto, Y. Zhao and L.-B. Han, J. Org. Chem., 2010, 75, 3890 CrossRef CAS PubMed;
(i) Y.-J. Ouyang, Y.-Y. Li, N.-B. Li and X.-H. Xu, Chin. Chem. Lett., 2013, 24, 1103 CrossRef CAS;
(j) B. Xiong, Y. Zhou, C. Zhao, M. Goto, S.-F. Yin and L.-B. Han, Tetrahedron, 2013, 69, 9373 CrossRef CAS;
(k) Y.-C. Liu and C.-F. Lee, Green Chem., 2014, 16, 357 RSC;
(l) G. Kumaraswamy and R. Raju, Adv. Synth. Catal., 2014, 356, 2591 CrossRef CAS;
(m) D. S. Panmand, A. D. Tiwari, S. S. Panda, J.-C. M. Monbaliu, L. K. Beagle, A. M. Asiri, C. V. Stevens, P. J. Steel, C. D. Hall and A. R. Katritzky, Tetrahedron Lett., 2014, 55, 5898 CrossRef CAS;
(n) J. Bai, X. Cui, H. Wang and Y. Wu, Chem. Commun., 2014, 50, 8860 RSC;
(o) S. Li, T. Chen, Y. Saga and L.-B. Han, RSC Adv., 2015, 5, 71544 RSC;
(p) X. Bi, J. Li, F. Meng, H. Wang and J. Xiao, Tetrahedron, 2016, 72, 706 CrossRef CAS;
(q) M. Xia and J. Cheng, Tetrahedron Lett., 2016, 57, 4702 CrossRef CAS;
(r) Y.-m. Lin, G.-p. Lu, G.-x. Wang and W.-b. Yi, J. Org. Chem., 2017, 82, 382 CrossRef CAS PubMed;
(s) Y. Moon, Y. Moon, H. Choi and S. Hong, Green Chem., 2017, 19, 1005 RSC.
- B. Kaboudin, Y. Abedi, J.-y. Kato and T. Yokomatsu, Synthesis, 2013, 45, 2323 CrossRef CAS.
- J. Wang, X. Huang, Z. Ni, S. Wang, J. Wu and Y. Pan, Green Chem., 2015, 17, 314 RSC.
- J.-G. Sun, H. Yang, P. Li and B. Zhang, Org. Lett., 2016, 18, 5114 CrossRef CAS PubMed.
- Y. Zhu, T. Chen, S. Li, S. Shimada and L.-B. Han, J. Am. Chem. Soc., 2016, 138, 5825 CrossRef CAS PubMed.
- S. Song, Y. Zhang, A. Yeerlan, B. Zhu, J. Liu and N. Jiao, Angew. Chem., Int. Ed., 2017, 56, 2487 CrossRef CAS PubMed.
-
(a) Q. Chen, X. Wang, C. Wen, Y. Huang, X. Yan and J. Zeng, RSC Adv., 2017, 7, 39758 RSC;
(b) Q. Chen, X. Yan, Z. Du, K. Zhang and C. Wen, J. Org. Chem., 2016, 81, 276 CrossRef CAS PubMed;
(c) Q. Chen, X. Yan, C. Wen, J. Zeng, Y. Huang, X. Liu and K. Zhang, J. Org. Chem., 2016, 81, 9476 CrossRef CAS PubMed;
(d) Q. Chen, J. Zeng, X. Yan, Y. Huang, C. Wen, X. Liu and K. Zhang, J. Org. Chem., 2016, 81, 10043 CrossRef CAS PubMed;
(e) Q. Chen, J. Zeng, X. Yan, Y. Huang, Z. Du, K. Zhang and C. Wen, Tetrahedron Lett., 2016, 57, 3379 CrossRef CAS.
- For selected examples, see:
(a) R. A. Dhokale and S. B. Mhaske, Org. Lett., 2013, 15, 2218 CrossRef CAS PubMed;
(b) J. Ballester, J. Gatignol, G. Schmidt, C. Alayrac, A.-C. Gaumont and M. Taillefer, ChemCatChem, 2014, 6, 1549 CrossRef CAS;
(c) T. Balalas, C. Peperidou, D. J. Hadjipavlou-Litina and K. E. Litinas, Synthesis, 2016, 48, 281 CAS;
(d) N. A. Dangroo, A. A. Dar, R. Shankar, M. A. Khuroo and P. L. Sangwan, Tetrahedron Lett., 2016, 57, 2717 CrossRef CAS;
(e) R. S. Shaikh, S. J. S. Düsel and B. König, ACS Catal., 2016, 6, 8410 CrossRef CAS;
(f) P. Huang and J. Xu, RSC Adv., 2016, 6, 63736 RSC;
(g) M.-T. Chen, X. You, L.-G. Bai and Q.-L. Luo, Org. Biomol. Chem., 2017, 15, 3165 RSC.
- J. L. G. Ruano, A. Parra and J. Alemán, Green Chem., 2008, 10, 706 RSC and references cited therein.
- W.-L. Dong, G.-Y. Huang, Z.-M. Li and W.-G. Zhao, Phosphorus, Sulfur Silicon Relat. Elem., 2009, 184, 2058 CrossRef CAS.
-
(a) T. J. Wallance and A. Schriesheim, J. Org. Chem., 1962, 27, 1514 CrossRef;
(b) T. J. Wallance, A. Schriesheim and W. Bartok, J. Org. Chem., 1963, 28, 1311 CrossRef.
- H. Wang, Q. Lu, C. Qian, C. Liu, W. Liu, K. Chen and A. Lei, Angew. Chem., Int. Ed., 2016, 55, 1094 CrossRef CAS PubMed.
Footnote |
† Electronic supplementary information (ESI) available: General information and copies of 1H, 13C, and 31P NMR spectra. See DOI: 10.1039/c7ra09057a |
|
This journal is © The Royal Society of Chemistry 2017 |
Click here to see how this site uses Cookies. View our privacy policy here.