DOI:
10.1039/C7RA09036F
(Paper)
RSC Adv., 2017,
7, 45045-45052
Attenuation of perfluorooctanoic acid-induced testicular oxidative stress and apoptosis by quercetin in mice
Received
15th August 2017
, Accepted 14th September 2017
First published on 21st September 2017
Abstract
Perfluorooctane acid (PFOA), a persistent environmental pollutant, is considered to cause adverse health effects. In the present study, we investigated the attenuating effect of quercetin (Que) on PFOA-induced testicular toxicity in mice and its possible mechanisms of action. Mice were orally administered PFOA (10 mg per kg per day) alone or in combination with Que (75 mg per kg per day) for 21 consecutive days. Treatment with Que resulted in restoration of absolute testis weight and epididymal sperm count in PFOA-exposed mice. Furthermore, compared with mice treated with PFOA alone, Que supplementation up-regulated testicular expression of NRF2 and its target antioxidant genes superoxide dismutase (SOD), catalase (CAT) and heme oxygenase-1 (HO-1), with a decrease in malondialdehyde production and an increase in SOD and CAT activities. In addition, treatment with Que up-regulated the expression of anti-apoptotic protein Bcl-2 and down-regulated the expression of pro-apoptotic proteins p53 and Bax in the testes of PFOA-exposed mice. These results suggested that Que protected against PFOA-induced male reproductive toxicity through attenuating oxidative stress and inhibiting apoptosis in the testes of mice.
1. Introduction
Perfluorinated compounds (PFCs) are a class of synthetic chemicals that have been used in a variety of commercial and industrial applications since the 1950s. In recent years, PFCs have received increasing attention due to their ubiquitous occurrence as persistent environmental pollutants. Perfluorooctanoic acid (PFOA), one of the most abundant PFCs in the environment, is mainly used as an emulsifier in the production of fluoropolymers and fluoroelastomers.1 Due to the nonbiodegradable and bioaccumulative properties, it has been detected in worldwide human populations.2–4 The consumption of contaminated food is the most important pathway leading to exposure to PFOA in the general public.5 It is well absorbed following oral exposure,1 and has a half-life of 3.8 years for serum elimination in humans.6 Data from NHANES 2007–2008 and 2009–2010 showed that the geometric mean PFOA concentrations in serum were 4.13 and 3.06 ng mL−1 in the U.S. population, respectively.7 However, occupational exposure raised serum level of PFOA, which could reach 691 ng mL−1 (range, 72–5100 ng mL−1) in fluorochemical production workers.8 In China, high levels of PFOA were detected in serum with median concentrations of 427 ng mL−1 (range, 2.52 to 32
000 ng mL−1) in occupational workers from 2008 to 2012.9
It has been suggested that PFC contamination may detrimentally affect spermatogenesis, disturbing meiotic segregation and DNA integrity in humans.10 A multivariable linear regression analysis demonstrated that in utero exposure to PFOA was associated with lower adjusted sperm concentration and total sperm count in adult men.11 Furthermore, a study of 105 young men indicated that high levels of serum PFOA-PFOS were associated with reduced numbers of normal spermatozoa.12 In animal experiments, PFOA has been shown to disrupt blood–testis barrier,13 destroy testicular steroidogenic machinery,14 and alter polyunsaturated fatty acid composition and induce oxidative stress in mouse epididymis,15 thus resulting in male reproductive dysfunction. We also reported previously that exposure to PFOA disrupted spermatogenesis through inducing testicular oxidative stress and apoptosis in mice.16
Quercetin (Que), an important aglycone flavonoid characterized by the presence of a phenyl benzo(c)pyrone-derived structure, is ubiquitously distributed in vegetables, fruits, tea and red wine and displays a wide range of healthy properties, including anti-oxidation, anti-inflammation and anti-apoptosis.17–19 It has been shown that Que exerts protective effect on hepatotoxicity induced by sodium fluoride and PFOA.20,21 However, the protection by Que against PFOA-induced male reproductive toxicity has not yet been reported. Therefore, the present study was undertaken to investigate the ameliorative effect of Que on PFOA-induced testicular oxidative stress and apoptosis in mice.
2. Materials and methods
2.1 Animals
Eight-week-old male Kunming mice were purchased from the Laboratory Animal Center of Nanchang University. The mice were housed under the standard laboratory conditions and acclimatized for 1 week before the start of experiments. All animal procedures were performed in accordance with the Guidelines for Care and Use of Laboratory Animals of Nanchang University and approved by the Animal Ethics Committee of Nanchang University.
2.2 Treatments
PFOA (purity 96%) and Que (purity ≥ 95%) were purchased from Sigma-Aldrich (St. Louis., MO, USA). The mice were randomly divided into four groups as follows: (1) control group: mice were given the vehicle only. (2) PFOA group: mice were given PFOA 10 mg per kg per day. (3) Que group: mice were given Que 75 mg per kg per day. (4) PFOA + Que group: mice were given PFOA 10 mg per kg per day and Que 75 mg per kg per day. PFOA and Que were dissolved respectively in water and dimethyl sulfoxide, and were administered intragastrically to each group of animals in a dose volume of 0.2 mL per 20 g body weight once daily for 21 consecutive days.
At the end of treatment period, mice were euthanized by cervical dislocation and testes and epididymides were excised quickly. The testes were fixed in Bouin's fluid or frozen in liquid nitrogen for the subsequent measurements.
2.3 Histopathology
The fixed testicle samples were dehydrated in an ascending series of alcohol, cleared in xylene, embedded in paraffin, and sectioned at 5 μm. The sections were stained with hematoxylin and eosin, and were observed under a microscope (Olympus IX71) for histopathological evaluation.
2.4 Sperm count
The freshly excised epididymis was minced and incubated in physiological saline at 37 °C for 10 min to allow spermatozoa to swim out. Epididymal sperm count was determined with a hemocytometer under a light microscope at ×200 magnification.
2.5 Oxidative stress analysis
The frozen testis was homogenized in ice-cold physiological saline solution with a glass homogenizer. The levels of malondialdehyde (MDA), superoxide dismutase (SOD) and catalase (CAT) in testis homogenate were detected using the commercial available kits (Jiancheng Institute of Biotechnology, Nanjing, China). MDA production was assayed by measuring the levels of thiobarbituric acid reactive substances (TBARS) at 532 nm. SOD activity was detected based on its ability to inhibit the oxidation of oxymine by the xanthine–xanthine oxidase system, and the absorbance was determined at 550 nm. CAT activity was determined by measuring the decrease in absorbance at 240 nm due to H2O2 dismutation. Protein concentrations were determined using the BCA assay kit (Vazyme Biotechnology, Nanjing, China). The analyses were performed with a UV 1800 spectrophotometer (Shimadzu, Japan).
2.6 Quantitative real-time PCR assay
Total RNA was extracted from the frozen testicle tissue using the Trizol solution (Invitrogen, CA). The cDNA was synthesized using the RT reagent kit with gDNA eraser (TaKaRa, China). Quantitative real-time PCR was carried out on an ABI Prism 7500 detection system (PE Applied Biosystems) using the SYBR Green PCR Master Mix (TaKaRa, China). The specific primers used for gene amplification were listed in Table 1. The expression of target genes was determined relative to GAPDH as an internal control, and the relative fold change in the mRNA expression was calculated using the 2−ΔΔCT.
Table 1 Specific primers for real-time PCR analysis
Gene |
Description |
Primer sequence (5′–3′) |
Product length (bp) |
HO-1 |
Forward |
CCTCACAGATGGCGTCACTT |
92 |
Reverse |
GCTGATCTGGGGTTTCCCTC |
92 |
SOD1 |
Forward |
ATCCACTTCGAGCAGAAGGC |
96 |
Reverse |
CTGATGGACGTGGAACCCAT |
96 |
CAT |
Forward |
TTTTGCCTACCCGGACACTC |
154 |
Reverse |
GGGGTAATAGTTGGGGGCAC |
154 |
GAPDH |
Forward |
GGCAAATTCAACGGCACAGT |
84 |
Reverse |
GTCTCGCTCCTGGAAGATGG |
84 |
2.7 Western blot analysis
Total protein was extracted from the frozen testicle tissue using RIPA lysis buffer for immunoblot assay. After separation by SDS-PAGE, protein samples were transferred onto a nitrocellulose membrane and immunoblotted sequentially with antibodies to NRF2, BCL-2, BAX, p53 or GAPDH (Cell Signaling Technology, USA.). After washing in TBST, the membranes were incubated with the secondary antibody conjugated with horseradish peroxidase (Santa Cruz Biotechnology Inc.) for 1 hour at room temperature. Immunoreactive bands were visualized by the enhanced chemiluminescence kit (Thermo Fisher Scientific Inc.), and blot images were acquired using a Bio-Rad gel imaging system.
2.8 Statistical analysis
The results were expressed as the mean ± standard deviation. The significance of differences was determined by one-way analysis of variance (ANOVA) and Duncan's multiple-range tests using the GLM procedure of SAS 8.1 software. P < 0.05 was considered statistically significant.
3. Results
3.1 Effect of Que on PFOA-induced changes in testicular histology
As shown in Fig. 1, treatment with PFOA for 21 consecutive days resulted in obvious morphological alterations in the testis of mice, including atrophy of seminiferous tubules, disorganization of seminiferous epithelium, absence of spermatozoa, depletion of spermatogonial cells and detachment of germ cells (Fig. 1B). However, all of these adverse histological changes induced by PFOA were significantly attenuated by simultaneous treatment with Que (Fig. 1D). Typical seminiferous tubular structures with normal arrangement of spermatogenic cells were observed in control mice (Fig. 1A), as well as in mice treated with Que alone (Fig. 1C).
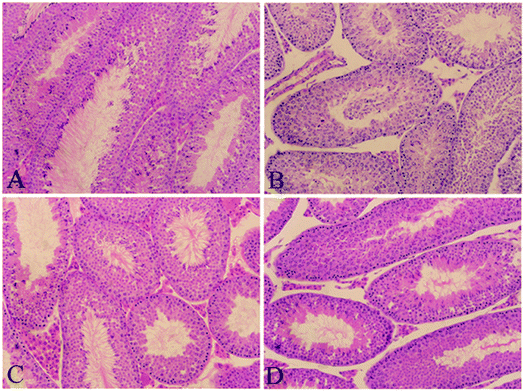 |
| Fig. 1 Histopathological staining. (A) Control group; (B) PFOA group; (C) Que group; (D) PFOA + Que group. Magnification: 200×. | |
3.2 Effect of Que on testicular weight in PFOA-treated mice
Compared with the control group, PFOA treatment for 21 consecutive days caused a significant decrease in absolute testis weight (P < 0.05). However, Que supplementation restored the absolute testis weight in mice exposed to PFOA (P < 0.05) (Fig. 2A). Treatment with PFOA, Que or their combination had no significant effect on testis weight relative to body weight (P > 0.05) (Fig. 2B).
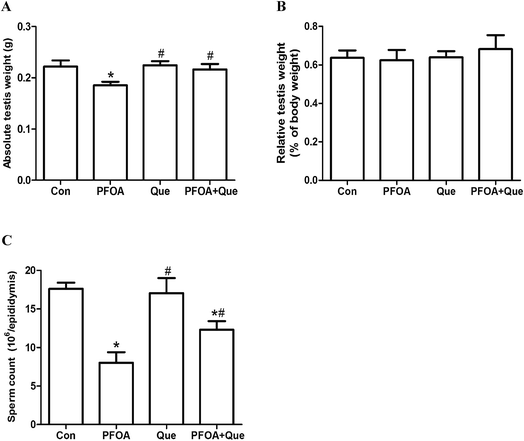 |
| Fig. 2 Effect of Que on absolute testis weight (A), relative testis weight (B) and epididymal sperm count (C) in PFOA-treated mice. Values are expressed as mean ± SD. *P < 0.05, versus control group; #P < 0.05, versus PFOA group. | |
3.3 Effect of Que on epididymal sperm count in PFOA-treated mice
The number of epididymal sperms was measured to evaluate the PFOA-induced reproductive toxicity and the protective effect of Que. Compared with the control, oral administration of PFOA for 21 consecutive days resulted in a significant decrease in epididymal sperm count (P < 0.05). However, simultaneous supplementation of Que markedly increased the number of epididymal sperms in mice exposed to PFOA (P < 0.05). No significant differences in epididymal sperm count were found between control group and Que group (P > 0.05) (Fig. 2C).
3.4 Effect of Que on PFOA-induced testicular oxidative stress
The levels of MDA, SOD and CAT in testis homogenates were determined to evaluate the antioxidative effect of Que on PFOA-induced testicular oxidative stress. After exposure to PFOA for 21 consecutive days, the formation of MDA was significantly increased and activities of SOD and CAT were significantly decreased in the testis, compared with control group (P < 0.05). However, simultaneous administration of Que significantly reduced the production of MDA and enhanced the activities of SOD and CAT in the testis of mice exposed to PFOA (P < 0.05). Compared with control group, Que treatment alone had no significant effect on the production of MDA and activities of SOD and CAT in the testis of mice (P > 0.05) (Fig. 3).
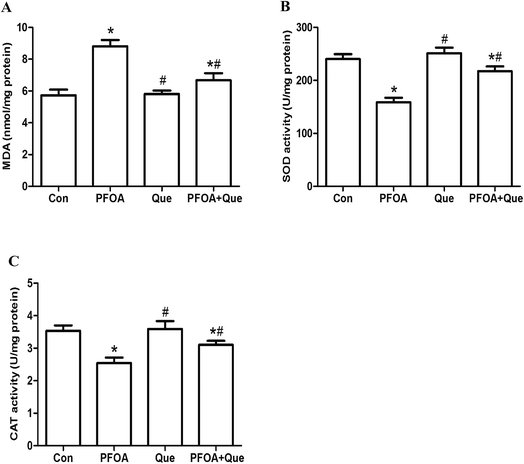 |
| Fig. 3 Effect of Que on testicular levels of MDA (A), SOD (B) and CAT (C) in PFOA-treated mice. Values are expressed as mean ± SD. *P < 0.05, versus control group; #P < 0.05, versus PFOA group. | |
3.5 Effect of Que on testicular expression of NRF2 and antioxidant enzymes in PFOA-treated mice
The expression of NRF2 and its downstream antioxidant genes HO-1, CAT and SOD was detected to analyze the involvement of NRF2-mediated antioxidant pathway. As shown in Fig. 4, oral administration of PFOA for 21 consecutive days significantly decreased the protein expression of NRF2 in the testis of mice (P < 0.05). However, PFOA-induced decrease in NRF2 expression was obviously restored by simultaneous Que treatment (P < 0.05).
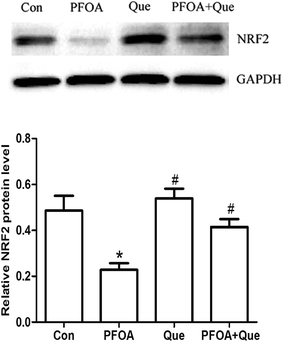 |
| Fig. 4 Effect of Que on protein expression of NRF2 in the testis of PFOA-treated mice. Values are expressed as mean ± SD. *P < 0.05, versus control group; #P < 0.05, versus PFOA group. | |
Moreover, mRNA expression of HO-1, CAT and SOD was markedly suppressed by PFOA exposure (P < 0.05). However, Que treatment significantly increased testicular expression of HO-1, CAT and SOD in mice exposed to PFOA (P < 0.05) (Fig. 5). Compared with control group, the protein expression of NRF2 and the mRNA expression of HO-1, CAT and SOD were up-regulated by Que treatment alone in the testis of mice (P < 0.05).
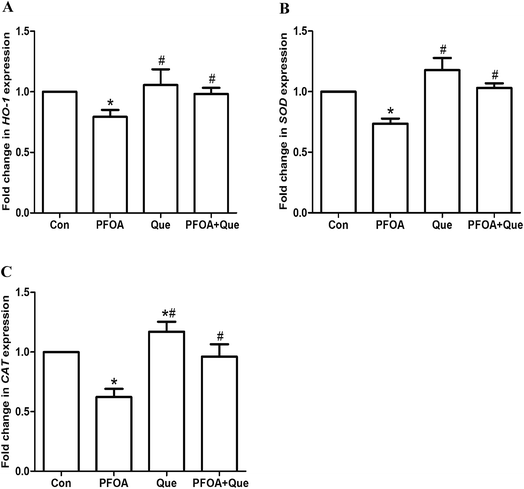 |
| Fig. 5 Effect of Que on mRNA expression of HO-1 (A), SOD (B) and CAT (C) in the testis of PFOA-treated mice. Values are expressed as mean ± SD. *P < 0.05, versus control group; #P < 0.05, versus PFOA group. | |
3.6 Effect of Que on PFOA-induced testicular apoptosis
The expression of p53, BAX and BCL-2 was measured by Western blot to evaluate the protective effect of Que against PFOA-induced testicular apoptosis. As shown in Fig. 6, exposure to PFOA for 21 consecutive days increased the expression of p53, p-p53 and BAX protein and decreased the expression of BCL-2 protein in the testis of mice (P < 0.05). However, the increase in p53, p-p53 and BAX expression and the decrease in BCL-2 expression induced by PFOA were significantly restored by combined treatment with Que (P < 0.05). Compared with control group, Que treatment alone had no significant effect on the expression of BAX and BCL-2 in the testis of mice (P > 0.05).
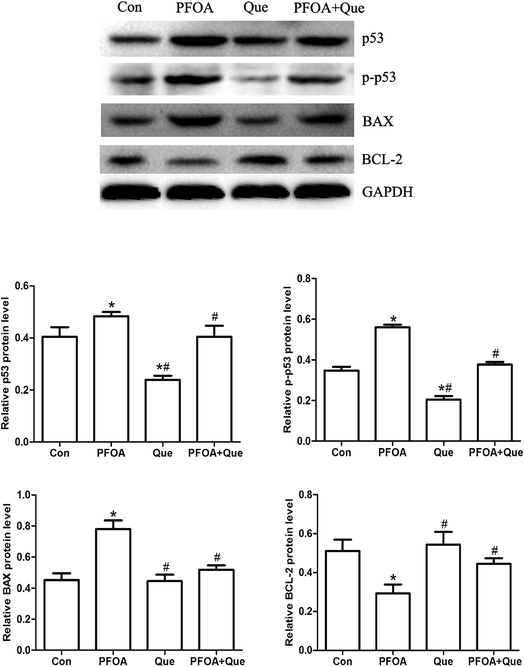 |
| Fig. 6 Effect of Que on protein expression of p53, p-p53, BAX and BCL-2 in PFOA-treated mice. Values are expressed as mean ± SD. *P < 0.05, versus control group; #P < 0.05, versus PFOA group. | |
4. Discussion
Exposure to environmental toxicants is one of the major causes for male infertility. As a stable compound extensively distributed in the environment, PFOA has been suggested to induce male reproductive dysfunction in humans and animals.11–16 Que, a natural antioxidant, has received considerable attention due to its potential health benefits. The presence of the catechol moiety and free hydroxyl groups in the Que structure is responsible for its antioxidant and free radical scavenger potential.17 Studies have shown that Que protects against testicular toxicity induced by various environmental pollutants in rats via its antioxidant property.19,22,23 Environmental pollutants, such as carbon tetrachloride, 2,3,7,8-tetrachlorodibenzo-p-dioxin and polychlorinated biphenyl, can result in testicular histopathological lesions and decreased reproductive organ weights.24–28 In the present study, PFOA-induced testicular damage in mice was evidenced by decreased testicular weight, reduced epididymal sperm count and disorganized seminiferous epithelium, which might be consequences of elevated oxidative stress and excessive apoptosis in the testis. It has been demonstrated that PFOA exposure reduces testosterone biosynthesis and production.14 The reduction in testosterone levels may be a potential reason for the decrement in testicular weight observed in this study. However, the adverse alterations induced by PFOA were restored by combined treatment with Que, suggesting that Que exerted ameliorative effect on PFOA-induced reproductive and developmental toxicity in mice.
Oxidative stress is a major pathological mechanism responsible for both male infertility and DNA damage in the germ line.29 It derives from the generation of reactive oxygen species (ROS) in excess of the antioxidant capacity of cells and results in severe oxidative damage to DNA, protein and lipids.30 MDA, a product of lipid peroxidation, is frequently used as an indicator of oxidative stress. Antioxidant enzymes, such as SOD and CAT play an important role in the elimination of ROS and provide the primary defense against oxidative stress. In this study, exposure to PFOA induced an increase in the production of MDA with a decrease in the activities of antioxidant enzymes CAT and SOD in the testis of mice, which may be due to the overproduction of ROS and the deficiency of antioxidant defenses, thereby resulting in testicular oxidative damage. However, the increased formation of MDA and decreased activities of SOD and CAT were significantly restored by simultaneous treatment with Que, suggesting that Que attenuated PFOA-induced oxidative damage in the testis of mice.
Nuclear factor-erythroid-2-related factor 2 (NRF2) is a key transcription factor that binds to antioxidant response elements (ARE) and protects against cytotoxicity caused by oxidative stress via the induction of defensive genes encoding stress-responsive enzymes and antioxidant proteins, such as SOD, CAT and HO-1.31 As an adaptive mechanism, NRF2 is quickly up-regulated in response to endogenous and exogenous stresses, but is down-regulated in cells exposed to an overwhelming or long-lasting oxidative stress.32 It has been suggested that NRF2 is important to spermatogenesis and may serve as a useful biomarker in the prediction of male infertility.33 NRF2 knockout mice had increased levels of testicular lipid peroxidation and decreased levels of antioxidants, which resulted in the disruption of spermatogenesis.34 Furthermore, low expression of NRF2 was observed in the spermatozoa of patients with asthenozoospermia and oligoasthenozoospermia.35 Many environmental contaminants such as cadmium, 4-nitrophenol and fluoride have been reported to induce testicular toxicity by reducing NRF2 expression in the testis.36–38 A previous study also demonstrated that PFOS-induced hepatotoxicity is associated with the inhibition of NRF2 expression.39 In the present study, exposure to PFOA for 21 consecutive days significantly reduced the expression of NRF2 and its target antioxidant genes SOD, CAT and HO-1 in the testis of mice, suggesting that NRF2-mediated antioxidant pathway plays a critical role in preventing testicular toxicity and the up-regulation of NRF2 may be a potential strategy to ameliorate testicular injury caused by PFOA.
Oxidative stress may be the central element in the regulation of the apoptotic pathways triggered by environmental stressors.40 The tumor suppressor protein p53 is an important redox-active transcription factor. It regulates intracellular redox state and induces apoptosis by a pathway that is dependent on ROS production.41 When p53 expression is relatively low, p53 enhances the protein level of NRF2 and its target genes to promote cell survival. However, at high levels or extended exposure of ROS, p53 expression is enhanced and NRF2-mediated survival response is inhibited, resulting in p53-dependent apoptosis.42 Therefore, PFOA-induced inhibition in NRF2 expression may be due to the excessive oxidative stress and increased p53 expression in the testis. In the present study, exposure to PFOA up-regulated the expression of pro-apoptotic p53 and Bax and down-regulated the expression of anti-apoptotic Bcl-2 in the testis of mice. The results demonstrated that PFOA treatment induced testicular cell apoptosis, which may be a consequence of decreased induction of NRF2-mediated antioxidant enzymes and increased oxidative stress in mice exposed to PFOA. However, the increased p53 and Bax expression and decreased Bcl-2 expression were significantly restored by simultaneous treatment with Que, indicating that Que attenuates PFOA-induced testicular toxicity by inhibiting apoptosis.
Previous studies have demonstrated that the up-regulation and activation of NRF2 attenuates diabetes-induced testicular oxidative damage and germ cell apoptosis.43,44 Que is considered to be a strong antioxidant due to its ability to scavenge free radicals. Molecular evidence has revealed that Que not only up-regulates the expression of NRF2 mRNA and protein, but also stabilizes NRF2 protein by inhibiting the ubiquitination and proteasomal turnover of NRF2.45 In our study, Que supplementation induced a significant increase in the expression of NRF2 and its downstream target genes HO-1, SOD and CAT in the testis of PFOA-exposed mice, suggesting that Que plays a protective role against PFOA-caused testicular toxicity via NRF2-mediated induction of antioxidant enzymes.
5. Conclusions
In conclusion, PFOA exposure resulted in testicular damage in mice by inducing excessive oxidative stress and apoptosis. However, Que protected against PFOA-induced testicular toxicity through attenuating oxidative stress and inhibiting apoptosis. The mechanism may be involved in the activation of NRF2-mediated antioxidant pathway. These findings propose a potential application of Que in the prevention and treatment of PFOA-induced male reproductive toxicity.
Conflicts of Interest
The authors declare that there are no conflicts of interest.
Acknowledgements
This study was supported by the National Natural Science Foundation of China (No. 81560248), Jiangxi Provincial Natural Science Foundation (No. 20161BAB205208 and 20142BAB215005) and National Undergraduate Training Program for Innovation and Entrepreneurship (No. 201610403041).
References
- C. Lau, K. Anitole, C. Hodes, D. Lai, A. Pfahles-Hutchens and J. Seed, Toxicol. Sci., 2007, 99, 366–394 CrossRef CAS PubMed.
- K. Kannan, S. Corsolini, J. Falandysz, G. Fillmann, K. S. Kumar, B. G. Loganathan, M. A. Mohd, J. Olivero, N. V. Wouwe, J. H. Yang and K. M. Aldous, Environ. Sci. Technol., 2004, 38, 4489–4495 CrossRef CAS PubMed.
- L. W. Yeung, M. K. So, G. Jiang, S. Taniyasu, N. Yamashita, M. Song, Y. Wu, J. Li, J. P. Giesy, K. S. Guruge and P. K. Lam, Environ. Sci. Technol., 2006, 40, 715–720 CrossRef CAS PubMed.
- H. Fromme, S. A. Tittlemier, W. Volkel, M. Wilhelm and D. Twardella, Int. J. Hyg. Environ. Health, 2009, 212, 239–270 CrossRef CAS PubMed.
- D. Trudel, L. Horowitz, M. Wormuth, M. Scheringer, I. T. Cousins and K. Hungerbuhler, Risk Anal., 2008, 28, 251–269 CrossRef PubMed.
- G. W. Olsen, J. M. Burris, D. J. Ehresman, J. W. Froehlich, A. M. Seacat, J. L. Butenhoff and L. R. Zobel, Environ. Health Perspect., 2007, 115, 1298–1305 CrossRef CAS PubMed.
- J. A. Gleason, G. B. Post and J. A. Fagliano, Environ. Res., 2015, 136, 8–14 CrossRef CAS PubMed.
- A. M. Calafat, Z. Kuklenyik, J. A. Reidy and L. L. Needham, Environ. Sci. Technol., 2007, 115, 1596–1602 CAS.
- J. Fu, Y. Gao, L. Cui, T. Wang, Y. Liang, G. Qu, B. Yuan, Y. Wang, A. Zhang and G. Jiang, Sci. Rep., 2016, 6, 38039 CrossRef CAS PubMed.
- L. Governini, C. Guerranti, V. De Leo, L. Boschi, A. Luddi, M. Gori, R. Orvieto and P. Piomboni, Andrologia, 2015, 47, 1012–1019 CrossRef CAS PubMed.
- A. Vested, C. H. Ramlau-Hansen, S. F. Olsen, J. P. Bonde, S. L. Kristensen, T. I. Halldorsson, G. Becher, L. S. Haug, E. H. Ernst and G. Toft, Environ. Health Perspect., 2013, 121, 453–458 Search PubMed.
- U. N. Joensen, R. Bossi, H. Leffers, A. A. Jensen, N. E. Skakkebæk and N. Jørgensen, Environ. Health Perspect., 2009, 117, 923–927 CrossRef CAS PubMed.
- Y. Lu, B. Luo, J. Li and J. Dai, Arch. Toxicol., 2016, 90, 971–983 CrossRef CAS PubMed.
- H. Zhang, Y. Lu, B. Luo, S. Yan, X. Guo and J. Dai, J. Proteome Res., 2014, 13, 3370–3385 CrossRef CAS PubMed.
- Y. Lu, Y. Pan, N. Sheng, A. Z. Zhao and J. Dai, Chemosphere, 2016, 158, 143–153 CrossRef CAS PubMed.
- W. Liu, B. Yang, L. Wu, W. Zou, X. Pan, T. Zou, F. Liu, L. Xia, X. Wang and D. Zhang, Biol. Reprod., 2015, 93, 41 Search PubMed.
- S. F. Nabavi, G. L. Russo, M. Daglia and S. M. Nabavi, Food Chem., 2015, 179, 305–310 CrossRef CAS PubMed.
- A. W. Boots, G. R. Haenen and A. Bast, Eur. J. Pharmacol., 2008, 585, 325–337 CrossRef CAS PubMed.
- M. Sönmez, G. Türk, S. Çeribaşı, M. Çiftçi, A. Yüce, M. Güvenç, Ş. Ö. Kaya, M. Çay and M. Aksakal, Andrologia, 2014, 46, 848–858 CrossRef PubMed.
- S. M. Nabavi, S. F. Nabavi, S. Eslami and A. H. Moghaddam, Food Chem., 2012, 132, 931–935 CrossRef CAS.
- W. Zou, W. Liu, B. Yang, L. Wu, J. Yang, T. Zou, F. Liu, L. Xia and D. Zhang, Int. Immunopharmacol., 2015, 28, 129–135 CrossRef CAS PubMed.
- M. F. Abd-Ellah, H. A. Aly, H. A. Mokhlis and A. H. Abdel-Aziz, Hum. Exp. Toxicol., 2016, 35, 232–243 CAS.
- F. Ben Abdallah, H. Fetoui, N. Zribi, F. Fakhfakh and L. Keskes, Environ. Toxicol., 2013, 28, 673–680 CrossRef CAS PubMed.
- M. Sönmez, G. Türk, A. O. Çeribasi, F. Sakin and A. Ateşşahin, Drug Chem. Toxicol., 2011, 34, 347–356 CrossRef PubMed.
- A. Ateşşahin, G. Türk, S. Yilmaz, M. Sönmez, F. Sakin and A. O. Çeribasi, Basic Clin. Pharmacol. Toxicol., 2010, 106, 479–489 CrossRef PubMed.
- G. Türk, S. Çeribaşı, M. Sönmez, M. Çiftçi, A. Yüce, M. Güvenç, Ş. Ö. Kaya, M. Çay and M. Aksakal, Toxicol. Ind. Health, 2016, 32, 126–137 CrossRef PubMed.
- A. Yüce, G. Türk, S. Çeribaşı, M. Güvenç, M. Çiftçi, M. Sönmez, Ş. Ö. Kaya, M. Çay and M. Aksakal, Andrologia, 2014, 46, 263–272 CrossRef PubMed.
- F. G. Bulmuş, F. Sakin, G. Türk, M. Sönmez and K. Servi, Toxicol. Environ. Chem., 2013, 95, 1019–1029 CrossRef.
- R. J. Aitken, T. B. Smith, M. S. Jobling, M. A. Baker and G. N. De Iuliis, Asian J. Androl., 2014, 16, 31–38 CrossRef CAS PubMed.
- J. L. Martindale and N. J. Holbrook, J. Cell. Physiol., 2002, 192, 1–15 CrossRef CAS PubMed.
- Y. J. Surh, J. K. Kundu and H. K. Na, Planta Med., 2008, 74, 1526–1539 CrossRef CAS PubMed.
- Y. Zhao, Y. Tan, J. Dai, B. Li, L. Guo, J. Cui, G. Wang, X. Shi, X. Zhang and N. Mellen, Toxicol. Lett., 2011, 200, 100–106 CrossRef CAS PubMed.
- K. Chen, Z. Mai, Y. Zhou, X. Gao and B. Yu, Tohoku J. Exp. Med., 2012, 228, 259–266 CrossRef CAS PubMed.
- B. N. Nakamura, G. Lawson, J. Y. Chan, J. Banuelos, M. M. Cortes, Y. D. Hoang, L. Ortiz, B. A. Rau and U. Luderer, Free Radical Biol. Med., 2010, 49, 1368–1379 CrossRef CAS PubMed.
- L. Zhang, Z. Liu, X. Li, P. Zhang, J. Wang, D. Zhu, X. Chen and L. Ye, Int. J. Clin. Exp. Pathol., 2015, 8, 14198 CAS.
- S. H. Yang, M. Long, L. H. Yu, L. Li, P. Li, Y. Zhang, Y. Guo, F. Gao, M. D. Liu and J. B. He, Int. J. Mol. Sci., 2016, 17, 1703 CrossRef PubMed.
- Y. Zhang, M. Song, X. Rui, S. Pu, Y. Li and C. M. Li, Toxicol. Rep., 2015, 2, 664–676 CrossRef CAS.
- S. Thangapandiyan and S. Miltonprabu, Asian Pac. J. Reprod., 2015, 4, 272–287 CrossRef.
- C. Wan, R. Han, L. Liu, F. Zhang, F. Li, M. Xiang and W. Ding, Toxicol. Appl. Pharmacol., 2016, 295, 85–93 CrossRef CAS PubMed.
- R. Franco, R. Sanchez-Olea, E. M. Reyes-Reyes and M. I. Panayiotidis, Mutat. Res., Genet. Toxicol. Environ. Mutagen., 2009, 674, 3–22 CrossRef CAS PubMed.
- T. M. Johnson, Z. X. Yu, V. J. Ferrans, R. A. Lowenstein and T. Finkel, Proc. Natl. Acad. Sci. U. S. A., 1996, 93, 11848–11852 CrossRef CAS.
- W. Chen, T. Jiang, H. Wang, S. Tao, A. Lau, D. Fang and D. D. Zhang, Antioxid. Redox Signaling, 2012, 17, 1670 CrossRef CAS PubMed.
- Y. Wang, Z. Zhang, W. Guo, W. Sun, X. Miao, H. Wu, X. Cong, K. A. Wintergerst, X. Kong and L. Cai, Am. J. Physiol.: Endocrinol. Metab., 2014, 307, E14–E23 CrossRef CAS PubMed.
- X. Jiang, Y. Bai, Z. Zhang, Y. Xin and L. Cai, Toxicol. Appl. Pharmacol., 2014, 279, 198–210 CrossRef CAS PubMed.
- S. Tanigawa, M. Fujii and D. X. Hou, Free Radical Biol. Med., 2007, 42, 1690–1703 CrossRef CAS PubMed.
|
This journal is © The Royal Society of Chemistry 2017 |