DOI:
10.1039/C7RA07938A
(Paper)
RSC Adv., 2017,
7, 40829-40833
An indolizine–rhodamine based FRET fluorescence sensor for highly sensitive and selective detection of Hg2+ in living cells†
Received
19th July 2017
, Accepted 16th August 2017
First published on 22nd August 2017
Abstract
An indolizine–rhodamine-based ratiometric fluorescent probe was designed and successfully synthesized. The probe shows a large Stokes shift (204 nm), high sensitivity and high selectivity. The detection limit was calculated to be as low as 8.76 nM. The probe could quickly (5 min) detect Hg2+ over a wide pH range from 5 to 10. Furthermore, it could be used for imaging Hg2+ in living cells.
Introduction
Mercury ions, as a dangerous and widespread global pollutant, can easily pass through skin, respiratory and gastrointestinal tissues into the human body, and damage the central nervous and endocrine systems. Therefore, accurate, rapid and cheap detection of mercury ions in vitro and in vivo with high selectivity and sensitivity is highly demanded.1–3
Several methods have been developed to monitor concentration levels of mercury such as atomic absorption spectroscopy, inductively coupled plasma-atomic emission spectrometry, gas chromatography-inductively coupled plasma-mass spectrometry, atomic fluorescence spectrometry (AFS), and reversed-phase high-performance liquid chromatography.4,5 These methods provide limits of detection at parts-per-billion level. However, their excellent performance is achieved at the expenses of elaborate and time-consuming sample preparation and preconcentration procedures. Most importantly, they cannot be used to detect Hg2+ in living cells. In general, fluorescence is a powerful optical analytical technique suitable for detecting low concentration of analytes.2
Since Tae reported the first Hg2+ probe based on rhodamine derivative,6 a number of intensity-based Hg2+ probes were synthesized. However, a major limitation of these simple “turn off” or “turn on” probes is that variations in probe concentration, probe environment, or excitation intensity may influence the fluorescence intensity measurements. Ratiometric sensors can eliminate most or all ambiguities by selfcalibration of two emission bands.7–10
Most ratiometric probes can be designed to function the following two mechanisms: intramolecular charge transfer (ICT) and Förster resonance energy transfer (FRET). However, some ICT-type ratiometric fluorescent probes have very broad emission spectra, which often lead to serious overlap in the emission peaks before and after interaction with target analytes. The above problems can be avoided by using a FRET-based sensor for which the single excitation wavelength of a donor fluorophore results in emission of the acceptor at a longer wavelength.11–30
In order to further improve biocompatibility, accuracy and detection efficiency of a probe, herein we present an indolizine–rhodamine FRET system TMUHg-2 as a ratiometric and intracellular Hg2+ sensor. A leuco-rhodamine derivative was chosen as a sensitive and selective chemosensor for Hg2+ ions. A highly efficient ring-opening reaction induced by Hg2+ generates the long-wavelength rhodamine fluorophore which can act as the energy acceptor. Indolizine, which was successfully synthesized via a novel tandem reaction by our group,31,32 was chosen as the energy donor because its max emission wavelength matches well with the absorption spectrum of rhodamine (Fig. S1†). As expected, TMUHg-2 could quickly (5 min) detect Hg2+ with high sensitivity and selectivity in a wide pH range from 5 to 10. The detection limit of 8.76 nM was achieved. More importantly, TMUHg-2 could be used for imaging Hg2+ in living cells.
Experimental
Materials and equipments
UV-vis spectra were measured with a Hitachi U-4100 spectrophotometer. Thin-layer chromatography involved silica gel 60 F254 plates (Merck KGaA) and column chromatography involved silica gel (mesh 200–300). All of the pH values were measured with a PHS-3C pH meter. Quartz cuvettes with a 1 cm path length and 3 mL volume were used in fluorescence and UV-vis spectrum measurements. Dry white wine (Changyu Pioneer Wine Company Limited, Yantai) were used with no pretreatment. UV-vis spectra and fluorescence spectra were recorded on a U-3900 UV-vis spectrometer (Hitachi) and RF-5301PC luminescence spectrophotometer (Shimadzu) at room temperature, respectively. 1H NMR and 13C NMR spectra were measured on a Bruker Avance 400 (400 MHz) spectrometer (CDCl3 as solvent and tetramethylsilane (TMS) as an internal standard). HRMS spectra were recorded on a Q-TOF6510 spectrograph (Agilent). All reagents and solvents were purchased from commercial sources and used without further purification. The solutions of metal ions were prepared from chlorizated salts which were dissolved in deionized water. Deionized water was used throughout the process of absorption and fluorescence determination.
Cell culture and imaging
Glioma cells (The department of pharmacology of Taishan Medical University, China, provided the cell line.) were cultured in RPMI-1640 containing 10% fetal bovine serum at 37 °C in a 5% CO2/95% air incubator. For living cells imaging experiments, the growth medium was removed and replaced with RPMI-1640 without FBS. The cells were treated and incubated with 2 μM of TMUHg-2 at 37 °C under 5% CO2 for 1 h. The cells were washed three times with PBS and then cell images were obtained via a confocal microscope from FV1000 (Olympus) at excitation of 405 nm.
Compound 1 and 2 were synthesized according to the literature.31–34
Synthesis of compound 3
Compound 1 (203 mg, 1 mmol) was dissolved in CH2Cl2 (20 mL), and then EDC (288 mg, 1.5 mmol) and DMAP (30 mg, 0.2 mmol) were added. Subsequently, compound 2 (470 mg, 1 mmol) was added, and the reaction mixture was stirred at room temperature for 6 h. Then the solvent was removed under reduced pressure to afford crude compound 3, which was purified on a silica gel column (C2H5OH
:
CH2Cl2 = 1
:
200) to afford pure compound 3 (491 mg, 75%). 1H NMR (400 MHz, CDCl3), δ 7.95–7.91 (m, 2H), 7.85 (s, 1H), 7.53 (s, 1H), 7.47 (m, 2H), 7.08 (m, 1H), 6.95 (s, 1H), 6.68 (m, 2H), 6.56 (s, 2H), 6.45 (m, 2H), 6.32 (m, 1H), 3.81 (s, 4H), 3.66 (s, 2H), 3.35 (q, J = 8.0 Hz, 4H), 3.27 (s, 4H), 2.57 (s, 3H), 1.17 (t, J = 8.0 Hz, 6H). 13C NMR (100 MHz, CDCl3), δ 194.62, 168.81, 166.21, 153.51, 151.63, 151.17, 148.95, 132.65, 131.46, 129.84, 129.60, 128.37, 128.16, 128.05, 125.59, 125.01, 123.74, 123.10, 120.54, 116.27, 112.18, 111.84, 110.06, 108.30, 104.11, 103.13, 102.55, 97.88, 65.51, 48.78, 44.37, 27.74, 12.53.
Synthesis of the probe TMUHg-2
Compound 3 (131 mg, 0.2 mmol) in DMF (1.5 mL) was added to a solution of phenyl isothiocyanate (0.1 mL, 0.65 mmol) in DMF (1.5 mL). The reaction mixture was stirred for 12 h at room temperature. After the solvent was evaporated under reduced pressure, the crude product was column chromatographed on silica gel (C2H5OH
:
CH2Cl2 = 1
:
200) to give the 80 mg (50%) of TMUHg-2. Mp: 170–171 °C. 1H NMR (400 MHz, CDCl3) δ 8.04 (d, J = 8.0 Hz, 1H), 7.93 (d, J = 8.0 Hz, 1H), 7.86 (s, 1H), 7.68 (m, 1H), 7.62 (m, 1H), 7.54 (s, 1H), 7.48 (s, 1H), 7.19 (m, 2H), 7.11 (m, 1H), 7.05 (d, J = 8.0 Hz, 2H), 6.96 (s, 1H), 6.89 (s, 1H), 6.69 (m, 2H), 6.53 (m, 3H), 6.44 (s, 1H), 6.30 (d, J = 8.0 Hz, 1H), 3.80 (s, 4H), 3.34 (m, 4H), 3.26 (s, 4H), 2.58 (s, 3H), 1.15 (t, J = 8.0 Hz, 6H). 13C NMR (100 MHz, CDCl3) δ 194.7, 168.8, 162.5, 154.1, 153.9, 152.1, 149.6, 149.4, 134.4, 131.5, 129.6, 129.3, 129.0, 128.4, 127.6, 125.6, 125.0, 124.7, 124.0, 120.6, 116.3, 112.4, 111.9, 108.7, 103.4, 102.6, 98.2, 66.8, 48.5, 44.4, 27.8, 12.5. HRMS: 790.3168 ([M + H]+); calcd for C46H44N7O4S: 790.3175.
Results and discussion
Synthesis of the probe TMUHg-2
The synthetic route to access probe TMUHg-2 is shown in Scheme 1. The reaction of Rhodamine B hydrazide 3 and phenyl isothiocyanate in DMF afforded probe TMUHg-2 as pink powder in 50% yield. The probe TMUHg-2 was characterized by 1H NMR, 13C NMR and HRMS (see ESI†).
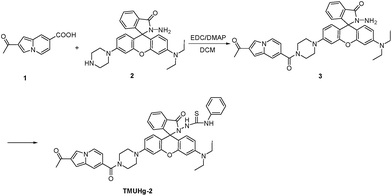 |
| Scheme 1 The synthesis of probe TMUHg-2. | |
The Fig. 1 demonstrated that TMUHg-2 was characteristic of high selectivity toward Hg2+ over other competitive metal ions and could serve as a “naked-eye’’ chemodosimeter. The competitive cations did not lead to any significant absorption changes in the visible region. However, a clear color change from colourless to pink was observed upon the addition of 1 equiv. of Hg2+.
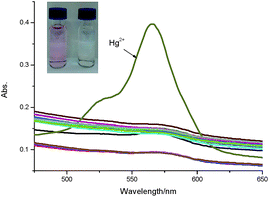 |
| Fig. 1 Absorbance spectra of TMUHg-2 (10 μM) in the absence and presence of 1 equiv. of different metal ions in C2H5OH/H2O (2/8, v/v) solution. | |
The absorption spectra of TMUHg-2 with varying Hg2+ concentrations (0–5 equiv.) in C2H5OH/water (2/8, v/v) were also recorded to further investigate the interaction of TMUHg-2 and Hg2+ (Fig. S2†). When Hg2+ was added to the solution of TMUHg-2 (10 μM), a new absorption band centered at 564 nm appeared with increasing intensity, which could be ascribed to the formation of the ring-opened form of TMUHg-2.
Fluorescence spectra
The selectivity of TMUHg-2 for Hg2+ was further observed in the fluorescent spectra. TMUHg-2 alone exhibited an emission of the indolizine centered about 434 nm when excited at 380 nm indicating the spirocyclic form of rhodamine moiety (Fig. 2). Addition of 1 equiv. of Hg2+ into the solution immediately resulted in a significant enhancement of fluorescent intensity at 584 nm and an obvious decrease of fluorescent intensity at 434 nm. Other competitive cations did not lead to any significant fluorescent changes. The phenomenon suggested that the Rhodamine was induced to be transformed into the open-ring structure from the spirolactam form by Hg2+.
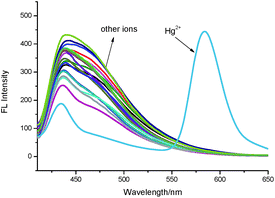 |
| Fig. 2 Fluorescence spectra of TMUHg-2 (1 μM) in the absence and presence of 1 equiv. of different metal ions in C2H5OH/H2O (2/8, v/v) solution (λex = 380 nm, slit = 15 nm/10 nm). | |
The fluorescence titration of TMUHg-2 was recorded as shown in Fig. 3. With the increasing concentrations of Hg2+, the intensity of fluorescence at 434 nm gradually decreased, while the band centering around 584 nm became prominent. The Stoke shift was 204 nm and the two emission peaks were well separated (150 nm). The results demonstrated that the developed FRET system effectively avoided the emission spectra overlap and ensured accuracy and high resolution in determining of Hg2+. More importantly, the fluorescence intensity ratio (I584/I434) increased dramatically with increasing amount of Hg2+, which changed about 54.6 times (from 0.046 to 2.51) when the concentration of Hg2+ was increased from 0 to 1 μM. There was a good linear relationship (Fig. S3†) in the concentration range from 0.02 μM to 0.10 μM of Hg2+ (R2 = 0.9858) and the detection limit was calculated to be as low as 8.76 nM. Also, it was investigated that the fluorescence responded in the presence of various coexistent anions (Fig. 4). It is gratifying to note that all the tested anions have no obvious interference with the fluorescence response of TMUHg-2 toward Hg2+. Moreover, the competitive experiments of the background metal ions were also studied. As shown in Fig. 5, the background metal ions showed very low interference with the detection.
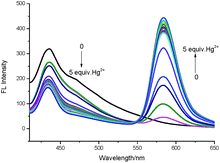 |
| Fig. 3 Fluorescence spectra of TMUHg-2 (1 μM) in response to Hg2+ (0.0–5.0 equiv.) in C2H5OH/H2O (2/8, v/v) solution (λex = 380 nm, slit = 15 nm/10 nm). | |
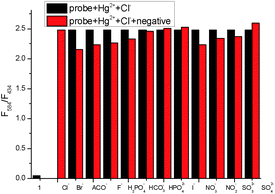 |
| Fig. 4 The ratiometric fluorescence responses F584/F434 of TMUHg-2 (1 μM) upon the addition of 1 μM HgCl2 in the presence of 1 μM background anions in C2H5OH/H2O (2/8, v/v) solution. | |
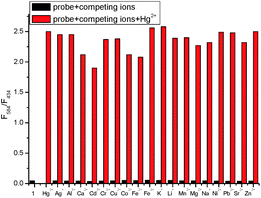 |
| Fig. 5 Metal-ion selectivity of TMUHg-2 in C2H5OH/H2O (2/8, v/v) solution. The black bars represent the fluorescence emission ratio (F584/F434) of a solution of TMUHg-2 (1 μM) and 1 equiv. of other metal ions. The red bars show the F584/F434 ratio after the addition of 1 equiv. of Hg2+ to the solution containing TMUHg-2 (1 μM) and different metal ions (1 μM) (λex = 380 nm, silt = 15 nm/10 nm). | |
Kinetic study
A time course of the fluorescence response of TMUHg-2 upon addition of Hg2+ is shown in Fig. 6. The kinetics of fluorescence intensity ratiometric changes of F584/F434 indicated that it could complete in 5 min so that it could be used as a ratiometric fluorescent probe for the fast detection of Hg2+.
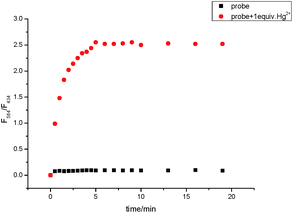 |
| Fig. 6 Time dependent increase of TMUHg-2 (1 μM) fluorescence intensities after addition of various amounts of Hg2+ in C2H5OH/H2O (2/8, v/v) solution (λex = 380 nm, silt = 15 nm/10 nm). | |
Effect of pH
In order to find a suitable pH span in which TMUHg-2 can selectively detect Hg2+ efficiently, the acid titration experiments were performed. As illustrated in Fig. 7, the free probe and the probe in the presence of Hg2+ did not show obvious changes of the fluorescence intensity ratio (F584/F434) between pH 5.0 and 10.0, suggesting that the probe TMUHg-2 might be used to detect Hg2+ in approximate physiological conditions.
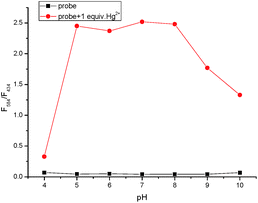 |
| Fig. 7 The ratiometric fluorescence responses (F584/F434) of free TMUHg-2 (1 μM) and in the presence of 1 equiv. of Hg2+ in C2H5OH/H2O (2/8, v/v) solution with different pH conditions (λex = 380 nm, silt = 15 nm/10 nm). | |
Mechanism
Based on the previous work,6 we envisioned that probe TMUHg-2 is in the spiro ring-closing state and the FRET must be off before reacting with Hg2+. Addition of Hg2+ may induce the rhodamine acceptor to be in the ring-opened form via Hg2+-promoted reaction of thiosemicarbazides to form 1,3,4-oxadiazoles and the FRET must be on (Scheme 2). The form of TMUHg-2-Hg2+ was also confirmed by HRMS analysis (Fig. S13 and S14†). When HgCl2 was added to TMUHg-2 in CH3OH, the peak of [TMUHg-2 + H]+ (790.3168) disappeared, and at the same time, a new peak of 756.3332 appeared, which suggested the formation of the compound [TMUHg-2-Hg − H]+
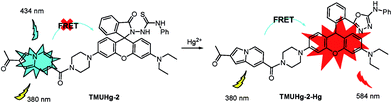 |
| Scheme 2 Proposed sensing mechanism of TMUHg-2 with Hg2+. | |
Cell imaging
The MTT assays (Fig. S4†) suggest that the ratiometric probe has low cytotoxicity to the cells. In light of the high sensitivity, selectivity and low cytotoxicity of TMUHg-2 for detecting Hg2+, the probe was applied to ratiometric fluorescence imaging in living cells. Glioma cells were incubated with only TMUHg-2 (2 μM) for 2 h at 37 °C, and the cells showed strong fluorescence in the blue channel (Fig. 8a) and weak fluorescence in the red channel (Fig. 8b). However, when cells were incubated with TMUHg-2 for 2 h, then incubated with Hg2+ for 30 min, the bright blue fluorescence darkened (Fig. 8d) and the faint red fluorescence brightened (Fig. 8e).
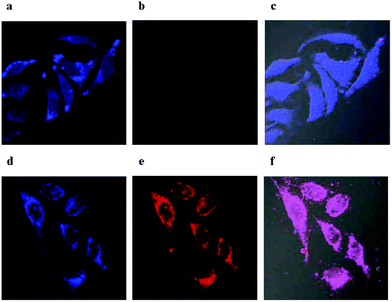 |
| Fig. 8 Images of glioma cells treated with the probe. Fluorescence image of glioma cells incubated with probe (2 μM) for 2 h ((a) the blue channel, (b) the red channel); (c) overlay image of (a and b) and bright field; fluorescence image of glioma cells incubated with probe (2 μM) for 2 h and then further incubated with Hg2+ (2 μM) for 30 min ((d) the blue channel, (e) the red channel); (f) overlay image of (d and e) and bright field. | |
Conclusions
In summary, a new indolizine–rhodamine-based Hg2+ ratiometric fluorescent probe was designed and successfully synthesized. The probe TMUHg-2 showed high selectivity and high sensitivity toward Hg2+. The detection limit was calculated to be as low as 8.76 nM. It could quickly (5 min) detect Hg2+ over a wide pH range from 5 to 10. Remarkably, the novel ratiometric fluorescent probes exhibited a large Stokes shift (204 nm) which can avoid auto-fluorescence, serious self-quenching and fluorescence detection errors. Furthermore, the probe has been successfully used for recognition of Hg2+ in cells.
Conflicts of interest
There are no conflicts to declare.
Acknowledgements
This work was supported by the Natural Science Foundation of China (21602153), the Science Fund of Shandong Province for Excellent Young Scholars (ZR201702180206) and Higher Educational Science and Technology Program of Shandong Province (J13LM01).
Notes and references
- E. M. Nolan and S. J. Lippard, Chem. Rev., 2008, 108, 3443 CrossRef CAS PubMed.
- Y. M. Yang, Q. Zhao, W. Feng and F. Y. Li, Chem. Rev., 2013, 113, 192 CrossRef CAS PubMed.
- H. R. Yang, C. M. Han, X. J. Zhu, Y. Liu, K. Y. Zhang, S. J. Liu, Q. Zhao, F. Y. Li and W. Huang, Adv. Funct. Mater., 2016, 26, 1945 CrossRef CAS.
- J. L. Chen, A. F. Zheng, A. H. Chen, Y. C. Gao, C. Y. He, X. M. Kai, G. H. Wu and Y. C. Chen, Anal. Chim. Acta, 2007, 599, 134 CrossRef CAS PubMed.
- K. H. Chu, Y. Zhou, Y. Fang, L. H. Wang, J. Y. Li and C. Yao, Dyes Pigm., 2013, 98, 339 CrossRef CAS.
- Y. K. Yang, K. J. Yook and J. Tae, J. Am. Chem. Soc., 2005, 127, 16760 CrossRef CAS PubMed.
- M. H. Lee, J. S. Kim and J. L. Sessler, Chem. Soc. Rev., 2015, 44, 4185 RSC.
- J. L. Fan, M. M. Hu, P. Zhan and X. J. Peng, Chem. Soc. Rev., 2013, 42, 29 RSC.
- L. Yuan, W. Y. Lin, K. B. Zheng and S. S. Zhu, Acc. Chem. Res., 2013, 46, 1462 CrossRef CAS PubMed.
- L. W. He, B. L. Dong, Y. Liu and W. Y. Lin, Chem. Soc. Rev., 2016, 45, 6449 RSC.
- X. L. Zhang, Y. Xiao and X. H. Qian, Angew. Chem., Int. Ed., 2008, 47, 8025 CrossRef CAS PubMed.
- P. Wang, L. Y. Zhao, H. G. Shou, J. Y. Wang, P. L. Zheng, K. Jia and X. B. Liu, Sens. Actuators, B, 2016, 230, 337 CrossRef CAS.
- M. Wang, J. Wen, Z. H. Qin and H. M. Wang, Dyes Pigm., 2015, 120, 208 CrossRef CAS.
- Y. Fang, Y. Zhou, J. Y. Li, Q. Q. Rui and C. Yao, Sens. Actuators, B, 2015, 215, 350 CrossRef CAS.
- B. L. Dong, X. Z. Song, C. Wang, X. Q. Kong, Y. H. Tang and W. Y. Lin, Anal. Chem., 2016, 88, 4085 CrossRef CAS PubMed.
- D. D. Cheng, W. Q. Zhao, H. Z. Yang, Z. P. Huang, X. L. Liu and A. X. Han, Tetrahedron Lett., 2016, 57, 2655 CrossRef CAS.
- L. W. He, S. S. Zhu, Y. Liu, Y. N. Xie, Q. Y. Xu, H. P. Wei and W. Y. Lin, Chem.–Eur. J., 2015, 21, 12181 CrossRef CAS PubMed.
- L. W. He, X. L. Yang, Y. Liu and W. Y. Lin, Anal. Methods, 2016, 8, 8022 RSC.
- L. Yuan, W. Y. Lin, Y. N. Xie, B. Chen and S. S. Zhu, J. Am. Chem. Soc., 2012, 134, 1305 CrossRef CAS PubMed.
- Q. J. Ma, X. B. Zhang, X. H. Zhao, Z. Jin, G. J. Mao, G. L. Shen and R. Q. Yu, Anal. Chim. Acta, 2010, 663, 85 CrossRef CAS PubMed.
- L. W. He, W. Y. Lin, Q. Y. Xu and H. P. Wei, Chem. Commun., 2015, 51, 1510 RSC.
- Y. L. Liu, X. Lv, Y. Zhao, M. L. Chen, J. Liu, P. Wang and W. Guo, Dyes Pigm., 2012, 92, 909 CrossRef CAS.
- P. H. Xie, F. Q. Guo, L. Y. Wang, S. Yang, D. H. Yao and G. Y. Yang, J. Fluoresc., 2015, 25, 319 CrossRef CAS PubMed.
- D. D. Cheng, W. Q. Zhao, H. Z. Yang, Z. P. Huang, X. L. Liu and A. X. Han, Tetrahedron Lett., 2016, 57, 2655 CrossRef CAS.
- W. Y. Lin, X. W. Cao, Y. D. Ding, L. Yuan and L. L. Long, Chem. Commun., 2010, 46, 3529 RSC.
- W. Y. Lin, X. W. Cao, Y. D. Ding, L. Yuan and Q. X. Yu, Org. Biomol. Chem., 2010, 8, 3618 CAS.
- J. H. Song, M. X. Huai, C. C. Wang, Z. H. Xu, Y. F. Zhao and Y. Ye, Spectrochim. Acta, Part A, 2015, 139, 549 CrossRef CAS PubMed.
- C. C. Wang, D. Zhang, X. V. Huang, P. G. Ding, Z. J. Wang, Y. F. Zhao and Y. Ye, Sens. Actuators, B, 2014, 198, 33 CrossRef CAS.
- G. C. Li, G. Q. Gao, J. Y. Cheng, X. P. Chen, Y. F. Zhao and Y. Ye, Luminescence, 2016, 31, 992 CrossRef CAS PubMed.
- J. Tang, S. G. Ma, D. Zhang, Y. Q. Liu, Y. F. Zhao and Y. Ye, Sens. Actuators, B, 2016, 236, 109 CrossRef CAS.
- Y. Q. Ge, J. Jia, H. Yang, G. L. Zhao, F. X. Zhan and J. W. Wang, Heterocycles, 2009, 78, 725 CrossRef CAS.
- Y. Q. Ge, A. K. Liu, J. Dong, G. Y. Duan, X. Q. Cao and F. Y. Li, Sens. Actuators, B, 2017, 247, 46 CrossRef CAS.
- Y. Q. Ge, R. X. Ji, S. L. Shen, X. Q. Cao and F. Y. Li, Sens. Actuators, B, 2017, 245, 875 CrossRef CAS.
- Y. Q. Ge, X. L. Zheng, R. X. Ji, S. L. Shen and X. Q. Cao, Anal. Chim. Acta, 2017, 965, 103 CrossRef CAS PubMed.
Footnote |
† Electronic supplementary information (ESI) available: 1H NMR, 13C NMR and MS spectra of probe, and additional cell images. See DOI: 10.1039/c7ra07938a |
|
This journal is © The Royal Society of Chemistry 2017 |
Click here to see how this site uses Cookies. View our privacy policy here.