DOI:
10.1039/C7RA06958H
(Paper)
RSC Adv., 2017,
7, 46344-46353
Some new azobenzene liquid crystals involving chalcone and ester linkages†
Received
22nd June 2017
, Accepted 26th September 2017
First published on 29th September 2017
Abstract
Five new series of azobenzene derivatives containing thiophene, naphthalene or ferrocene with chalcone and ester linkages have been synthesized and characterized. The photosensitive azobenzene group underwent photoisomerization under UV light, which was monitored by UV-visible spectroscopy. The cyclic voltammograms of compounds containing ferrocene showed a quasi-reversible and diffusion-controlled redox process. These compounds displayed high thermal stability according to the thermogravimetry measurements. According to differential scanning calorimetry, thermal polarizing microscopy and powder X-ray diffraction studies, the compounds containing ferrocene and the compounds with three or no terminal alkoxy chains on the side of the ester group all showed no liquid crystal behaviour. However, the compounds with a terminal alkoxy chain on the side of the ester group or a terminal alkoxy chain at both ends of the molecule exhibited enantiotropic mesophases, but the latter had a narrow mesogenic domain. The increase of the length of the mesogenic unit by replacement of the benzene ring with a naphthalene ring resulted in both an increase in the clearing point and in an increase in the mesophase domain.
1 Introduction
Thermotropic liquid crystals (LCs) are a special category of soft matter; their molecular order and dynamics are intermediate between an isotropic fluid and that of a crystal.1 LC materials are promising candidates for fabricating cost-effective and portable bio/chemical sensors, and for interfacial and optoelectronic applications.2–4 A thermotropic liquid crystal consists of either linear or disclike molecules. The general architecture for linear thermotropic liquid crystals consists of linearly linked ring systems and flexible chains. The rings may be linked to each other either directly or through some linking group (e.g. ester, olefinic, acetylenic, azo, imido) that maintains the linearity of the core.5 Azobenzene, as one of the traditional photochromic moieties, is thermally very stable and can perform photo-induced reversible isomerization under UV irradiation,6–10 So azo compounds have advantages over substances with other linkages like ester, tolane or even the more commonly encountered Schiff's base linkage.11–13 In recent decades, azobenzene based low and high molecular weight liquid crystalline materials have been frequently discussed for their sensitivity of chromophoric group towards light and found to exhibit interesting optical properties, which enable us to study the materials in holography, optical storage device, optical switching and establish widespread applications in display technology.14–19
On the other hand, chalcones are the main precursors for the biosynthesis of flavonoids and isoflavonoids. A variety of organic molecules with chalcone moiety have been evaluated for pharmacological applications due to their good antimicrobial,20 nematocidal,21 anticancer22 and antiplasmodial23 activities. In addition, chalcones are reported to be effective photosensitive materials and have potential nonlinear optical24 and liquid crystal properties.25 Chalcone linkage along with other linkages, such as imine and ester groups, combined to exhibit various mesophases.26–31 However, to the best of our knowledge, there are rare reports about the combination of azo and chalcone linkages using in the liquid-crystalline materials to date.32,33 In this article, we synthesized a series of new liquid crystalline compounds involving chalcone, azo and ester linkages. The designed molecular structures are shown in Fig. 1. Their redox and optical properties were studied. Thermometric data will be evaluated in terms of molecular rigidity and flexibility depending on molecular structure and then mesomorphic properties and behaviors will be compared with structurally similar series.
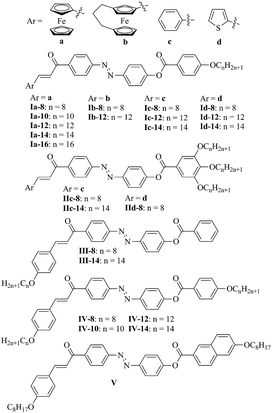 |
| Fig. 1 The structures of compounds I–V. | |
2 Results and discussion
2.1 Photoisomerisation studies
The photoisomerisation studies were carried out on the selective compounds dissolved in the dichloromethane. The initial measurements were carried out on the unexposed materials. The obtained results of the UV-visible absorption spectra (UV-vis) are presented in Fig. 2 and Table S1.†
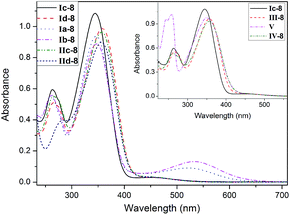 |
| Fig. 2 UV-vis absorption spectra of selective compounds I–V taken at 10−5 M in CH2Cl2. | |
Compounds Ia-8 and Ib-8 exhibited three absorption bands, whereas others exhibited two absorption bands. The absorption bands in ultraviolet area were attributed to the π–π* electronic transitions. For the compound V, the absorption band centered at ∼260 nm (see Fig. 2 insert) had large molar absorption coefficient after introducing the naphthalene ring. The absorption due to Fe(d)-π* electronic transitions in compounds Ia-8 and Ib-8 also appeared at the range of 260 nm. In addition, the same absorption at 346 nm for compounds Ia, Ib and Ic indicated that the introduction of ferrocenyl almost had no effect on this absorption. However, this band shifted bathochromically about 10 nm after introduction of thiophene ring (compounds Id and IId) or alkoxy group on the side of chalcone (compounds III, IV and V). Furthermore, compounds Ia-8 and Ib-8 with ferrocenyl showed very broad absorption bands at 480–620 nm due to d–d type transitions of the electrons of the iron atoms, and this band for the [3]ferrocenophane-containing Ib-8 was stronger than that of ferrocene-containing 1a-8.34
Next, the compounds were irradiated with UV light (365 nm). As an example, the spectral changes of Ic are presented in Fig. 3. With increasing time of irradiation the band at 342 nm strongly decreased. This change in the UV-vis spectrum suited the reduction of concentration of E isomers in azo group in irradiated mixture. At the same time, the increase of the band at 440 nm (n–π* electron transition) corresponded to the growth of the concentration of Z isomer in azo group in investigated mixture. Moreover, the equilibrium between Z and E isomers in azo group was established after 75 s of irradiation. The isosbestic points were observed at 312 and 408 nm, corresponding to E–Z isomerization as these suggesting that only two isomers obtained. Furthermore, another absorption band exhibited chalcone unit in double bond underwent isomerization around 263 nm.35 Table 1 shows the summary of time taken for the selected compounds for E–Z photoisomerisation with their calculated conversion efficiency (CE).7 As shown in Table 1, 1a-8 took the longest time (157 s) for E–Z conversion with the CE of 27.3% after exposure to UV light, whereas Ic-8 gave 45.1% of CE when bulky ferrocene was replaced by a benzene ring. However, Id-8, IV-8 and V-8 took shorter time (40–50 s) with the low CE of 8–10% after introducing alkoxy chain in benzene ring. The possible reason is the bulky ferrocene and alkoxy chain increasing the energy of Z isomer.
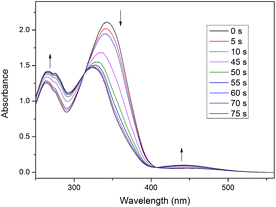 |
| Fig. 3 Changes of absorption spectra of Ic-8 in CH2Cl2 during UV exposure. | |
Table 1 Time taken for the azo derivatives for E–Z isomerisation with their calculated conversion efficiency
Compd. |
E–Z (time) (s) |
CE (%) |
Ia-8 |
157 |
27.3 |
Ic-8 |
75 |
45.1 |
Id-8 |
40 |
9.6 |
IV-8 |
50 |
10.2 |
V-8 |
40 |
8.7 |
After irradiation samples were left in the dark to allow thermal back relaxation. This process for Ic-8 is presented in Fig. 4. It can be seen that back transition from Z to E form was much slower than the isomerisation during the UV irradiation, and the equilibrium for compound Ic-8 was not achieved yet after 1485 min. Similar phenomena were observed in other compounds. Long thermal back relaxation allows us to realize that optical storage devices with these materials which need longer periods.
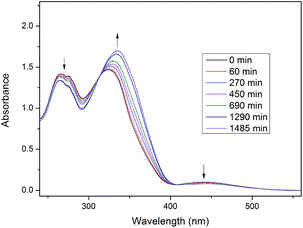 |
| Fig. 4 Changes of absorption spectra of Ic-8 in CH2Cl2 during thermal back relaxation. | |
2.2 Electrochemical investigation
The electrochemical properties of representative compounds containing ferrocene (Ia-8, Ia-12 and Ib-12) were studied by cyclic voltammetry (CV) using CH2Cl2 as the solvent containing 0.1 M tetra-n-butylammoniumhexafluorophosphate (TBAPF6) as a supporting electrolyte. Electrochemical data are shown in Table 2. Selected CV curves are shown in Fig. 5. All redox-active ferrocenyl groups exhibited one-electron transfer processes with formal redox potentials E1/2 = 146–148 mV for Ia-8, Ia-12 and E1/2 = 79 mV for Ib-12 vs. Fc/Fc+, which indicates that lengthening of the alkoxy chain has little effect on the formal redox potentials of ferrocene. However, as compared to Ia-8 and Ia-12, the potentials for Ib-12 cathodically shifted about 70 mV thereby suggesting easy oxidation by loss of an electron for [3]ferrocenophane-containing derivatives. A reasonable explanation is the effect of trimethylene group as an electron donor in [3]ferrocenophane-containing derivative leading to the lower oxidation potential of ferrocene.36 From Table 2, we found the ipc/ipa ratios approached 1, but the ΔE value was in the range of 143–149 mV indicating a quasi-reversible redox step for studied compounds. In addition, as shown in Fig. 6, the redox potentials of Ib-8 slightly influenced by the scan rate in a range from 10 to 300 mV s−1, and both the anodic and cathodic peak currents are linear to the square root of scan rates, indicating a diffusion-controlled process.34
Table 2 CV data of 10−3 M solutions of selected compounds in CH2Cl2 containing 0.1 M TBAPF6 as supporting electrolyte at 100 mV s−1 scan rate. Potentials are vs. Fc/Fc+
Compd. |
Epaa/mV |
Epcb/mV |
ΔEc/mV |
E1/2d/mV |
ipae/μA |
ipa/ipcf |
Anodic peak potential. Cathodic peak potential. ΔE = Epa − Epc. E1/2 = 1/2(Epa + Epc). Anodic peak current. ipc, cathodic peak current. |
Ia-8 |
223 |
74 |
149 |
148 |
27.4 |
0.96 |
Ia-12 |
217 |
74 |
143 |
146 |
25.0 |
0.89 |
Ib-12 |
151 |
6 |
145 |
79 |
29.4 |
0.99 |
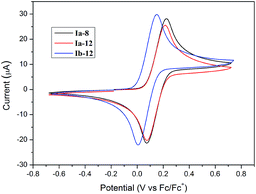 |
| Fig. 5 CV curves of selective compounds Ia–Ib in CH2Cl2, 0.1 M TBAPF6. Scan rate = 100 mV s−1. | |
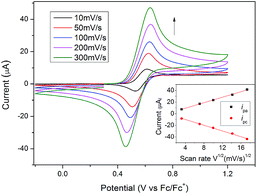 |
| Fig. 6 CV curves and peak currents of Ib-8 obtained at different scan rates in CH2Cl2. | |
2.3 Thermal behavior
Thermal stability of these compounds was measured by simultaneous thermogravimetric and differential scanning calorimetry (TG-DSC) measurement under N2. The TG curves of Ia–Id are shown in Fig. S1.† From the TG curves, we can see that the decomposition temperatures were somewhat higher for Ic-8 and Id-8 (about 300 °C) than for Ia-8 and Ib-8 (about 270 °C), which indicated the higher thermal stability of the former.
Mesomorphic properties of these compounds had been investigated. The textural studies of them have been performed using thermal polarizing microscopy (POM) and the thermal analyses have been carried out using DSC. The thermal analyses data of compounds Ia, Ib, II and III are presented in Table S2.† As shown in Table S2,† compounds Ia–Ib did not display LC behaviors, however, most of them gave rise to crystal to crystal or crystal polymorphic phase transitions upon heating and cooling cycles. For the series of compounds Ia, the phase transition temperature of crystal to isotropic liquid lowered gradually with increasing the length of the terminal alkoxy chain from eight to fourteen carbon atoms, but this temperature raised when further lengthening the terminal alkoxy chain to sixteen carbon atoms. In addition, this temperature for the series of compounds Ib with the same length of terminal alkoxy chain lowered obviously when replacing ferrocene by [3]ferrocenophane.
Compounds Ia–Ib were non-mesomorphic. It seems to like that there is unfavourable effect of bulky ferrocene in present system which is disadvantageous to molecule packing, so ferrocene was replaced by a benzene ring or thiophene ring in the following study. Compounds Ic–Id with one terminal alkoxy chain on the side of ester group were prepared, and we were pleased to find that they all displayed liquid crystal behaviors and showed similar phase transitions in the heating and cooling cycles. As examples, the DSC curves of Ic-8 and Id-8 are shown in Fig. 7. At the beginning of the first heating cycle, crystal to crystal phase transition was observed for Ic-8, then an endothermic peak appeared at 167 °C with large enthalpy change of 82.0 kJ mol−1 corresponding to the melting point followed by the formation of mesophase with the range of 61 °C. The mesophase exhibited marbled texture as presented in Fig. 8a which was identified by POM.37 On slow cooling from the isotropic liquid, the enantiotropic mesophase was observed. The droplet texture for Ic-8, fourbrush schlieren texture for Ic-12 and marbled texture for Ic-14 appeared on cooling as presented in Fig. 8b–d. X-ray diffraction (XRD) experiments were further performed to elucidate the mesomorphic properties. As shown in Fig. 9, the XRD patterns of Ic-8 presented one diffuse peak in wide-angle region during the heating and cooling cycle after entering into the mesophase, which demonstrated a disordered position of mesogen. Taking into account the DSC, POM and XRD results, this series compounds probably possessed enantiotropic smectic C (SmC) phase.38 As shown in Table 3, the mesophase ranges (ΔT) in the cooling cycles were 79.1 °C for Ic-8, 56.4 °C for Ic-12 and 51.5 °C for Ic-14 respectively, which narrowed gradually with increasing the length of the terminal alkoxy chain. The lower homologues Ic-8 had the widest mesogenic domain and the highest clearing temperature (∼229 °C). Similar thermal behaviors were observed in series Id. Moreover, for the compound with same length of terminal alkoxy chain, the mesophase range of the series Id containing thiophene (91.0 °C for Id-8, 66.0 °C for Id-12 and 58.7 °C for Id-14) was wider, and the clearing points of series Id were higher than those of the series Ic. The POM images of Id-8 at 232.6 °C and Id-12 at 213.0 °C on cooling were presented in Fig. 8e and f. Therefore, the presence of heteroatom S being more polarizable than carbon resulted in great changes in the corresponding liquid crystalline phases and physical properties of the observed phases.
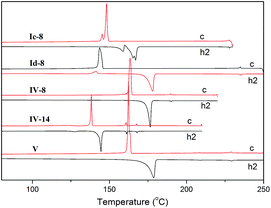 |
| Fig. 7 DSC traces of selected compounds. h2: the second heating, c: the first cooling. | |
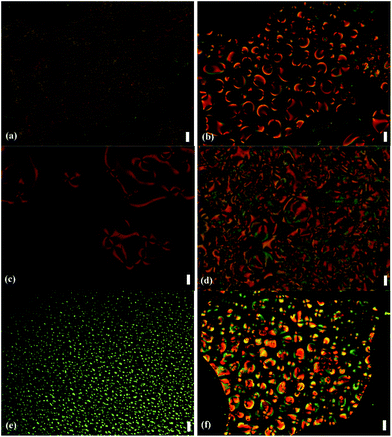 |
| Fig. 8 Polarizing optical microscopy images (20×) (a) Ic-8 at 195.0 °C on heating, (b) Ic-8 at 229.8 °C (c) Ic-12 at 207.4 °C (d) Ic-14 at 200.4 °C (e) Id-8 at 232.6 °C (f) Id-12 at 213.0 °C on cooling. Scale bar, 30 μm. | |
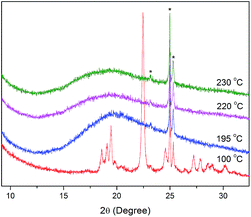 |
| Fig. 9 XRD patterns of compound Ic-8 on heating 195 °C and cooling 230 °C, 220 °C and 100 °C. Asterisks in the spectrum show the alumina from the sample cell holder. | |
Table 3 Phase transition temperatures and associated enthalpies of compounds 1–4
Compd. |
Phase transitionsa °C (ΔH kJ−1 mol−1) |
First heating |
Second heating |
First cooling |
ΔTb |
C: crystal, M: mesophase, I: isotropic liquid. Mesophase range. |
Ic-8 |
C1 71.7 (8.3) C2 158.2 (6.8) C3 167.6 (82.0) M 228.7 (0.9) I |
C2 158.8 (16.2) C3 166.8 (57.5) M 227.9 (1.2) I |
I 227.6 (−1.13) M 148.5 (−56.2) C3 145.3 (−9.5) C2 |
79.1 |
Ic-12 |
C1 102.2 (17.6) C2 128.2 (6.8) C3 161.1 (78.0) M 207.5 (1.3) I |
C 160.7 (72.3) M 205.9 (1.5) I |
I 205.2 (−1.31) M 148.8 (−71.2) C |
56.4 |
Ic-14 |
C1 113.0 (31.7) C2 164.4 (86.6) M 201.8 (1.6) I |
C1 61.2 (12.3) C2 164.2 (82.0) M 201.1 (1.6) I |
I 200.3 (−1.3) M 148.8 (−80.1) C2 66.0 (−11.3) C1 |
51.5 |
Id-8 |
C 178.8 (97.9) M 236.7 (2.21) I |
C1141.4 (−10.4) C2 177.8 (91.3) M 234.9 (2.04) I |
I 234.9 (−1.93) M 143.9 (−71.4) C |
91.0 |
Id-12 |
C1 103.6 (32.1) C2 159.4 (128.9) M 213.8 (2.4) I |
C1 51.4 (5.4) C2 158.9 (116.5) M 212.9 (2.7) I |
I 212.5 (−2.2) M 146.5 (−110.7) C2 45.4 (−6.3) C1 |
66.0 |
Id-14 |
C1 113.8 (31.7) C2 160.5 (84.5) M 205.3 (1.4) I |
C 159.7 (78.0) M 205.3 (1.5) I |
I 204.6 (−1.57) M 145.9 (−76.6) C |
58.7 |
IV-8 |
C1 123.2 (8.0) C2 176.0 (54.3) M 190.2 (0.6) I |
C1 176.1 (52.2) M 189.9 (0.6) I |
I 189.8 (−0.4) M 163.8 (−49.2) C |
26.0 |
IV-10 |
C1 76.5 (15.4) C2 151.2 (42.5) M 181.1 (0.6) I |
C 158.6 (43.9) M 180.8 (0.7) I |
I 180.7 (−0.8) M 144.9 (−34.7) C |
35.8 |
IV-12 |
C1 82.5 (5.6) C2 98.8 (8.3) C3 104.7 (1.8) C4 146.8 (44.9) M1 153.0 (0.8) M2 172.8 (0.9) I |
C1 113.9 (2.8) C2 147.4 (34.5) M1 152.84 (0.6) M2 172.6 (0.8) I |
I 172.3 (−0.9) M2 152.6 (−0.9) M1 142.6 (−29.9) C |
29.7 |
IV-14 |
C1 92.56 (5.5) C2 109.55 (27.0) C3 144.1 (43.4) M1 161.2 (1.9) M2 167.9 (0.4) I |
C1 127.5 (4.0) C2 144.3 (37.9) M1 161.1 (2.0) M2 167.8 (0.6) I |
I 167.6 (−0.8) M2 160.8 (−1.8) M1 138.5 (−26.9) C |
29.1 |
V |
C 180.4 (40.8) M 235.7 (0.8) I |
C 178.4 (38.3) M 229.1 (0.7) I |
I 229.1 (−0.5) M 163.3 (−34.8) C |
65.8 |
Compounds Ic–Id all exhibited enantiotropic mesomorphic behaviours, but their melting points were high. In order to obtain mesogenic compounds with low melting point, compounds IIc–IId with three terminal alkoxy chains were prepared. As shown in Table S2,† the melting points of compounds IIc-8, IIc-14 and IId-8 are 73.5, 72.7, and 94.1 °C respectively, lowering 85–94 °C compared with compounds Ic-8, Ic-14 and Id-8. However, compounds IIc–IId with three terminal alkoxy chains were non-mesomorphic, but showed either simple melting and freezing process or crystal polymorphic phase transitions in the heating and cooling cycles. From above observations, it seemed likely that increasing the number of long chain was unfavourable for inducing mesomorphism due to increasing the disorder of molecular.
To better understand the effect of the position of long chain on the formation of mesophases, compounds III-8 and III-14 with terminal alkoxy chain on the side of chalcone were prepared. We were surprised to find that compounds III-8 and III-14 showed no liquid crystal behaviours. As shown in Table S2,† compounds III-8 and III-14 also displayed crystal polymorphic phase transitions in the heating and cooling cycles, and their melting points (III-8, 184.8 °C; III-14, 173.6 °C) were slight higher than those of compounds Ic-8 (167.6 °C) and Ic-14 (164.4 °C).
Next, we studied the thermal behaviours of compounds IV–V with a terminal alkoxy chain at both ends of the molecular. From Table 3, we can see that the melting point of compound IV-8 (176.0 °C) increased by about 10 °C compared with 1c-8 (167.6 °C), whereas the increase of the length of the flexible chain (IV-12, 146.8 °C; IV-14, 144.1 °C) resulted in a decrease of the melting point by 15–20 °C compared with Ic-12 (161.1 °C) and Ic-14 (164.4 °C). According DSC and POM observation, the series IV all showed enantiotropic mesophases but with the narrow mesogenic domains (26–36 °C) and low clearing temperature compared with the series of Ic. Upon heating, compounds IV-8 and IV-10 having shorter chains exhibited schlieren texture after entering into the mesophase, and showed fourfold brush near the melting point (Fig. 10a). The inversing process was also observed on cooling. However, as shown in Table 3, IV-12 and IV-14 having longer chains displayed two mesogenic domains. As an example, on cooling from isotropic of IV-14, fourbrush schlieren texture was observed at 169.6 °C as displayed in Fig. 10b and further cooling, dendritic texture were exhibited at 162.7 °C as displayed in Fig. 10c.39 The XRD patterns of IV-14 (Fig. 11) presented one diffuse peak during the cooling cycle after entering into the mesophase indicating the possibility of SmC phase.
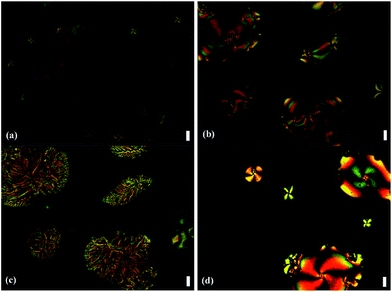 |
| Fig. 10 Polarizing optical microscopy images (20×) (a) IV-8 at 192.4 °C on heating, (b) IV-14 at 169.6 °C (c) IV-14 at 162.7 °C (d) V at 239.6 °C on cooling. Scale bar, 30 μm. | |
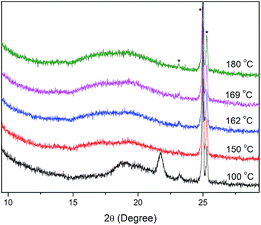 |
| Fig. 11 XRD patterns of compound IV-14 on cooling. Asterisks in the spectrum show the alumina from the sample cell holder. | |
The increase of the length of the mesogenic unit by replacement benzene ring with naphthalene ring (compound V) resulted in both an increase of the clearing point by about 46 °C compared with its analogue, compound IV-8, and in an increase of the SmC mesophase domain (by about 40 °C), but the melting point changed slightly. Fig. 10d showed the POM image of V at 239.6 °C on cooling. Thus, the lengthening the rigid core may favour a better packing of the molecules which was beneficial to exhibiting liquid crystal properties.
3 Conclusion
In summary, we have synthesized 23 azobenzene compounds involving chalcone and ester linkages. The compounds containing ferrocene exhibit good electrochemical properties. Investigations of liquid crystal properties indicated that the compounds containing ferrocene (Ia–Ib series) and the compounds with three (IIc–IId series) or no (III series) terminal alkoxy chains on the side of ester group all showed no liquid crystal behaviours. However, the compounds with a terminal alkoxy chain on the side of ester group (Ic–Id series) or a terminal alkoxy chain at both ends of the molecular (IV–V series) exhibited enantiotropic SmC phases. The replacement benzene ring with naphthalene ring results in both an increase of the clearing point and in an increase of the mesophase domain. Some of these azo molecules underwent photoisomerization under UV light. Very long thermal back relaxation has potential advantage in the creation of optical storage devices.
4 Experimental
4.1 Materials and measurements
Detailed procedures of synthesis and characterization of compounds VIa–VIe were described in ESI.† Acyl chloride VII was prepared according to reported procedures.25 IR spectra were recorded as KBr pellets on a Bruker-ALPHA spectrometer. NMR spectra were recorded on an Avance 500 Bruker (500 MHz) spectrometer using tetramethylsilane as internal standard. HRMS spectra were recorded on a Bruker ultrafleXtreme MALDI-TOF/TOF mass spectrometer. Elemental analysis was performed on a Perkins-Elmer 2400 elemental analyser. Electronic absorption spectra were recorded on Shimadzu UV2600 spectrophotometer. Thermal stability was measured by EXSTAR TG/DTA7300 simultaneous TG-DSC measurement under N2. DSC thermographs were obtained on a METTLER TOLEDO DSC3 at a heating rate of 10 °C min−1 under nitrogen flow. Powder XRD experiments were conducted using a D8 ADVANCE equipped with a LynxEye detector, CuKα radiation source, and graphite monochromator, λ = 1.54 Å. CV experiments were recorded on AUTOLAB PGSTAT302 voltammetric analyzer and performed at room temperature in dry CH2Cl2 solutions with the concentration of 10−3 M containing 0.1 M TBAPF6 as a supporting electrolyte. A three-electrode configuration consisting of a glassy carbon working electrode, a Pt wire counter electrode, and an Ag/AgCl (with a saturated KCl solution) couple reference electrode was used. The Fc/Fc+ couple was used as internal reference and showed a peak at +0.484 V vs. Ag/AgCl. Prior to experiments, the system was purged with purified nitrogen gas to exclude dissolved oxygen from the solution.
4.2 General procedure for the synthesis of compounds I–V
Compound VI (0.45 mmol) was redissolved in dry pyridine (2 mL), to which acyl chloride VII (0.5 mmol) in dry pyridine (2 mL) was added dropwise at −5 °C. The reaction was stirred for 4 h at room temperature. The solvent was removed by distillation at reduced pressure and the residue was separated by silica gel column chromatography using CH2Cl2/petroleum ether as eluent. The second fraction was the desired product, which was recrystallized from CH2Cl2/methanol to afford the pure compounds I–V (Scheme 1).
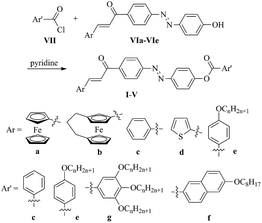 |
| Scheme 1 Synthesis of compounds I–V. | |
Ia-8. Yield 67%, mp 172–178 °C, Rf = 0.117 (CH2Cl2
:
petroleum ether = 2
:
1); IR (KBr, ν, cm−1): 3102, 2950, 2919, 2853, 1733, 1654, 1592, 1509, 1315, 1261, 1074, 979; 1H NMR (500 MHz, CDCl3) (δ ppm): 8.17–8.13 (m, 4H, C6H4), 8.06–8.00 (m, 4H, C6H4), 7.81 (d, J = 15.0 Hz, 1H, CH), 7.41 (d, J = 8.5 Hz, 2H, C6H4), 7.17 (d, J = 15.0 Hz, 1H, CH), 7.00 (d, J = 9.0 Hz, 2H, C6H4), 4.63 (d, J = 2.0 Hz, 2H, FcH), 4.52 (d, J = 2.0 Hz, 2H, FcH), 4.21 (s, 5H, FcH), 4.06 (t, J = 6.5 Hz, 2H, OCH2), 1.88–1.78 (m, 2H, CH2), 1.52–1.44 (m, 2H, CH2), 1.37–1.45 (m, 8H, CH2), 0.90 (t, J = 7.0 Hz, 3H, CH3); 13C NMR (125 MHz, CDCl3) (δ ppm): 188.97, 164.62, 163.82, 154.62, 153.76, 150.23, 147.55, 132.45, 129.49, 124.49, 122.98, 122.65, 121.17, 114.46, 72.74, 72.30, 70.58, 69.67, 68.44, 31.86, 29.38, 29.28, 26.04, 22.71, 14.16; HRMS, MALDI+, m/z: calcd for C40H40FeN2O4: [M+] 668.2337, found: 668.2333.
Ia-10. Yield 71%, mp 182–184 °C, Rf = 0.263 (CH2Cl2
:
petroleum ether = 3
:
1); IR (KBr, ν, cm−1): 3051, 2922, 2851, 1731, 1654, 1590, 1512, 1316, 1267, 1104, 978; 1H NMR (500 MHz, CDCl3) (δ ppm): 8.16 (d, J = 8.5 Hz, 2H, C6H4), 8.12 (d, J = 7.0 Hz, 2H, C6H4), 8.05 (d, J = 8.5 Hz, 2H, C6H4), 8.01 (d, J = 8.0 Hz, 2H, C6H4), 7.75 (d, J = 14.0 Hz, 1H, CH), 7.40 (d, J = 9.0 Hz, 2H, C6H4), 7.13 (d, J = 14 Hz, 1H, CH), 6.99 (d, J = 9.0 Hz, 2H, C6H4), 4.76 (s, 2H, FcH), 4.64 (s, 2H, FcH), 4.33 (s, 5H, FcH), 4.06 (t, J = 6.5 Hz, 2H, OCH2), 1.86–1.80 (m, 2H, CH2), 1.51–1.45 (m, 2H, CH2), 1.41–1.19 (m, 12H, CH2), 0.89 (t, J = 7.0 Hz, 3H, CH3); 13C NMR (125 MHz, CDCl3) (δ ppm): 189.09, 164.59, 163.78, 154.58, 153.72, 150.19, 147.54, 132.41, 129.39, 124.45, 122.93, 122.61, 121.13, 119.02, 114.42, 71.61, 69.90, 69.16, 68.40, 31.91, 29.57, 29.36, 29.11, 26.00, 22.70, 14.14; HRMS, MALDI+, m/z: calcd for C42H44FeN2O4: [M+] 696.2651, found: 696.2648.
Ia-12. Yield 66.5%, mp 164–168 °C, Rf = 0.27 (CH2Cl2
:
petroleum ether = 3
:
1); IR (KBr, ν, cm−1): 3088, 2920, 2850, 1728, 1653, 1602, 1510, 1314, 1256, 1070, 979; 1H NMR (500 MHz, CDCl3) (δ ppm): 8.16 (d, J = 8.5 Hz, 2H, C6H4), 8.12 (d, J = 8.0 Hz, 2H, C6H4), 8.05 (d, J = 8.5 Hz, 2H, C6H4), 8.01 (s, 2H, C6H4), 7.73 (b, 1H, CH), 7.40 (d, J = 8.5 Hz, 2H, C6H4), 7.14 (b, 1H, CH), 6.99 (d, J = 8.5 Hz, 2H, C6H4), 4.83 (s, 2H, FcH), 4.69 (s, 2H, FcH), 4.37 (s, 5H, FcH), 4.06 (t, J = 6.5 Hz, 2H, OCH2), 1.84–1.80 (m, 2H, CH2), 1.49–1.46 (m, 2H, CH2), 1.37–1.20 (m, 16H, CH2), 0.88 (t, J = 6.5 Hz, 3H, CH3); 13C NMR (125 MHz, CDCl3) (δ ppm): 188.98, 164.59, 163.78, 154.57, 153.72, 150.19, 146.41, 132.41, 129.55, 124.45, 122.99, 122.61, 121.13, 114.41, 71.17, 69.97, 69.63, 68.40, 31.94, 29.63, 29.37, 22.71, 14.14; HRMS, MALDI+, m/z: calcd for C44H48FeN2O4: [M+] 724.2964, found: 724.2966.
Ia-14. Yield 72.3%, mp 172–176 °C, Rf = 0.27 (CH2Cl2
:
petroleum ether = 3
:
1); IR (KBr, ν, cm−1): 3094, 2921, 2849, 1732, 1654, 1590, 1512, 1316, 1269, 1077, 977; 1H NMR (500 MHz, CDCl3) (δ ppm): 8.16 (d, J = 8.5 Hz, 2H, C6H4), 8.13 (d, J = 8.0 Hz, 2H, C6H4), 8.05 (d, J = 8.5 Hz, 2H, C6H4), 8.01 (d, J = 8.0 Hz, 2H, C6H4), 7.79 (d, J = 15.0 Hz, 1H, CH), 7.40 (d, J = 8.5 Hz, 2H, C6H4), 7.17 (d, J = 15.0 Hz, 1H, CH), 6.98 (d, J = 8.5 Hz, 2H, C6H4), 4.66 (s, 2H, FcH), 4.55 (s, 2H, FcH), 4.23 (s, 5H, FcH), 4.06 (t, J = 6.5 Hz, 2H, OCH2), 1.86–1.80 (m, 2H, CH2), 1.49–1.45 (m, 2H, CH2), 1.37–1.26 (m, 20H, CH2), 0.88 (t, J = 6.5 Hz, 3H, CH3); 13C NMR (125 MHz, CDCl3) (δ ppm): 189.00, 164.59, 163.78, 154.58, 153.71, 150.18, 147.55, 132.41, 129.45, 124.45, 122.93, 122.61, 119.12, 114.41, 70.51, 69.63, 69.34, 68.40, 31.94, 29.64, 29.38, 29.11, 26.00, 22.71, 14.15; HRMS, MALDI+, m/z: calcd for C46H52FeN2O4: [M+] 752.3277, found: 752.3278.
Ia-16. Yield 73%, mp 168–170 °C, Rf = 0.275 (CH2Cl2
:
petroleum ether = 3
:
1); IR (KBr, ν, cm−1): 3094, 2950, 2920, 2854, 1732, 1653, 1592, 1509, 1316, 1260, 1074, 979; 1H NMR (500 MHz, CDCl3) (δ ppm): 8.16 (d, J = 8.5 Hz, 2H, C6H4), 8.11 (b, 2H, C6H4), 7.05 (d, J = 8.5 Hz, 2H, C6H4), 8.01 (b, 2H, C6H4), 7.71 (b, 1H, CH), 7.40 (d, J = 8.5 Hz, 2H, C6H4), 7.14 (s, 1H, CH), 6.98 (d, J = 8.5 Hz, 2H, C6H4), 4.84 (s, 2H, FcH), 4.71 (s, 2H, FcH), 4.39 (s, 5H, FcH), 4.05 (t, J = 6.5 Hz, 2H, OCH2), 1.85–1.80 (m, 2H, CH2), 1.49–1.46 (m, 2H, CH2), 1.37–1.20 (m, 24H, CH2), 0.88 (t, J = 6.7 Hz, 3H, CH3); 13C NMR (125 MHz, CDCl3) (δ ppm): 188.94, 164.58, 163.78, 154.57, 153.72, 151.74–151.56, 150.18–149.98, 132.41, 124.45, 122.98, 122.61, 121.13, 114.41, 68.41, 31.95, 29.71, 29.64, 29.38, 26.00, 22.71, 14.15; HRMS, MALDI+, m/z: calcd for C48H56FeN2O4: [M+] 780.3590, found: 780.3585.
Ib-8. Yield 77.2%, mp 142–145 °C, Rf = 0.23 (CH2Cl2
:
petroleum ether = 3
:
1); IR (KBr, ν, cm−1): 3078.37, 2926.80, 2852.78, 1731.00, 1650.52, 1603.11, 1509.38, 1260.60, 1070.15, 976.68; 1H NMR (500 MHz, CDCl3) (δ ppm): 8.17 (d, J = 8.5 Hz, 2H, C6H4), 8.11 (d, J = 8.5 Hz, 2H, C6H4), 8.05 (d, J = 8.5 Hz, 2H, C6H4), 8.00 (s, 2H, C6H4), 7.82 (b, 1H, CH), 7.40 (d, J = 8.5 Hz, 2H, C6H4), 7.05 (b, 1H, CH), 6.99 (d, J = 8.5 Hz, 2H, C6H4), 4.65 (b, 2H, FcH), 4.51–4.29 (m, 3H, FcH), 4.17–4.04 (m, 3H, FcH, OCH2), 3.93 (s, 1H, FcH), 2.02–1.62 (m, 8H, CH2), 1.52–1.45 (m, 2H, CH2), 1.42–1.24 (m, 8H, CH2), 0.90 (t, J = 6.5 Hz, 3H, CH3); 13C NMR (125 MHz, CDCl3) (δ ppm): 188.66, 164.58, 163.77, 162.62, 154.38, 153.66, 150.20, 149.61, 147.76, 147.73, 147.70, 132.41, 132.41, 131.16, 129.31, 125.80, 124.48, 124.41, 123.11, 123.03, 122.60, 121.13, 114.41, 89.67, 86.60, 73.49, 73.47, 70.78, 69.99, 68.40, 34.85, 31.82, 29.71, 29.34, 29.24, 29.01, 26.00, 24.54, 24.09, 22.67, 14.13; HRMS, ESI m/z: calcd for C43H44FeN2O4: [M]+ 708.2646, found: 708.2631.
Ib-12. Yield 78.5%, mp 140–144 °C, Rf = 0.215 (CH2Cl2
:
petroleum ether = 3
:
1); IR (KBr, ν, cm−1): 3124.18, 2923.63, 2853.37, 1727.16, 1648.34, 1602.12, 1510.01, 1258.84, 1075.98, 975.69. 1H NMR (500 MHz, CDCl3) (δ ppm): 8.16 (d, J = 8.5 Hz, 2H, C6H4), 8.11 (d, J = 6.5 Hz, 2H, C6H4), 8.06 (d, J = 8.5 Hz, 2H, C6H4), 8.00 (d, J = 7.0 Hz, 2H, C6H4), 7.76 (d, J = 14.0 Hz, 1H, CH), 7.41 (d, J = 8.5 Hz, 2H, C6H4), 7.05 (d, J = 14.0 Hz, 1H, CH), 7.00 (d, J = 9.0 Hz, 2H, C6H4), 4.54 (s, 2H, FcH), 4.36 (s, 1H, FcH), 4.33 (s, 1H, FcH), 4.28 (s, 1H, FcH), 4.07 (t, J = 6.5 Hz, 3H, OCH2), 3.90 (s, 1H, FcH), 2.06–1.89 (m, 6H, CH2), 1.86–1.80 (m, 2H, CH2), 1.51–1.45 (m, 2H, CH2), 1.37–1.25 (m, 16H, CH2), 0.89 (t, J = 6.5 Hz, 3H, CH3); 13C NMR (125 MHz, CDCl3) (δ ppm): 189.04, 164.53, 163.79, 154.46, 153.70, 150.24, 147.64, 147.64, 140.56, 132.39, 129.30, 124.39, 122.89, 122.55, 114.43, 89.63, 86.58, 73.44, 73.43, 70.80, 70.24, 70.22, 70.20, 70.06, 68.41, 34.83, 31.91, 29.61, 29.35, 29.10, 25.99, 24.55, 24.11, 22.67, 14.08; HRMS, ESI m/z: calcd for C47H52FeN2O4: [M]+ 764.3272, found: 764.3263.
Ic-8. Yield 72.5%, mp 158–165 °C, Rf = 0.25 (CH2Cl2
:
petroleum ether = 2
:
1); IR (KBr, ν, cm−1): 3052, 2953, 2922, 2853, 1729, 1656, 1605, 1573, 1466, 1306, 1259, 1066, 842; 1H NMR (500 MHz, CDCl3) (δ ppm): 8.19 (d, J = 8.5 Hz, 2H, C6H4), 8.17 (d, J = 9.0 Hz, 2H, C6H4), 8.07 (d, J = 8.5 Hz, 2H, C6H4), 8.03 (d, J = 8.5 Hz, 2H, C6H4), 7.86 (d, J = 15.5 Hz, 1H, CH), 7.69–7.68 (m, 2H, C6H5), 7.58 (d, J = 15.5 Hz, 1H, CH), 7.45–7.44 (m, 3H, C6H5), 7.42 (d, J = 9.0 Hz, 2H, C6H4), 6.99 (d, J = 8.5 Hz, 2H, C6H4), 4.06 (t, J = 6.5 Hz, 2H, OCH2), 1.86–1.81 (m, 2H, CH2), 1.51–1.47 (m, 2H, CH2), 1.42–1.23 (m, 8H, CH2), 0.90 (t, J = 7.0 Hz, 3H, CH3); 13C NMR (125 MHz, CDCl3) (δ ppm): 189.85, 164.58, 163.79, 154.83, 153.79, 150.17, 145.34, 139.69, 134.81, 132.41, 130.75, 129.59, 129.04, 128.57, 124.49, 123.01, 122.62, 121.99, 121.12, 114.42, 68.40, 31.82, 29.34, 29.23, 29.11, 26.00, 22.67, 14.12; HRMS, MALDI+ m/z: calcd for C36H37N2NaO4: [M + Na]+ 583.2567, found: 583.2560.
Ic-12. Yield 75.3%, mp 150–156 °C, Rf = 0.277 (CH2Cl2
:
petroleum ether = 2
:
1); IR (KBr, ν, cm−1): 3052, 2953, 2821, 2853, 1729, 1656, 1605, 1578, 1495, 1306, 1259, 1066, 842; 1H NMR (500 MHz, CDCl3) (δ ppm): 8.18 (d, J = 8.5 Hz, 2H, C6H4), 8.16 (d, J = 9.0 Hz, 2H, C6H4), 8.05 (d, J = 9.0 Hz, 2H, C6H4), 8.02 (d, J = 8.5 Hz, 2H, C6H4), 7.85 (d, J = 15.5 Hz, 1H, CH), 7.69–7.68 (m, 2H, C6H5), 7.58 (d, J = 15.5 Hz, 1H, CH), 7.45–7.44 (m, 3H, C6H5), 7.42 (d, J = 9.0 Hz, 2H, C6H4), 7.00 (d, J = 9.0 Hz, 2H, C6H4), 4.06 (t, J = 6.5 Hz, 2H, OCH2), 1.86–1.80 (m, 2H, CH2), 1.48–1.45 (m, 2H, CH2), 1.37–1.26 (m, 16H, CH2), 0.89 (t, J = 7.0 Hz, 3H, CH3); 13C NMR (125 MHz, CDCl3) (δ ppm): 189.84, 164.57, 163.79, 154.82, 153.79, 150.16, 145.34, 139.68, 134.81, 132.41, 130.75, 129.59, 129.04, 128.58, 124.49, 123.01, 122.62, 121.98, 121.12, 114.42, 68.40, 31.94, 29.67, 29.65, 29.60, 29.57, 29.37, 29.11, 26.00, 22.71, 14.15; HRMS, MALDI+ m/z: calcd for C40H45N2O4: [M + H]+ 617.3379, found: 617.3350. Elemental analysis: calcd for C40H44N2O4: C 77.89, H 7.19, N 4.54%; found: C 77.73, H 7.55, N 4.33%.
Ic-14. Yield 76.9%, mp 162–164 °C, Rf = 0.28 (ethyl acetate
:
petroleum ether = 1
:
20); IR (KBr, ν, cm−1): 3125.77, 2954.43, 2849.44, 1736.54, 1657.86, 1608.91, 1575.47, 1497.17, 1307.46, 1259.58, 1082.75, 842.04; 1H NMR (500 MHz, CDCl3) (δ ppm): 8.18 (d, J = 9.0 Hz, 2H, C6H4), 8.16 (d, J = 9.0 Hz, 2H, C6H4), 8.06 (t, J = 9.0 Hz, 2H, C6H4), 8.04 (t, J = 8.5 Hz, 2H, C6H4), 7.88 (d, J = 15.5 Hz, 1H, CH), 7.69–7.68 (m, 2H, C6H5), 7.60 (d, J = 15.5 Hz, 1H, ArH), 7.42–7.44 (m, 3H, C6H5), 7.40 (d, J = 9.0 Hz, C6H4), 7.00 (d, J = 9.0 Hz, 2H, C6H4), 4.06 (t, J = 6.5 Hz, 2H, OCH2), 1.86–1.80 (m, 2H, CH2), 1.51–1.45 (m, 2H, CH2), 1.37–1.26 (m, 20H, CH2), 0.88 (t, J = 7.0 Hz, 3H, CH3); 13C NMR (125 MHz, CDCl3) (δ ppm): 189.86, 164.58, 163.79, 153.79, 145.35, 139.69, 134.80, 132.42, 130.75, 129.59, 129.04, 128.58, 124.49, 123.01, 122.63, 121.98, 114.42, 68.40, 31.94, 29.71, 29.69, 29.61, 29.38, 29.11, 26.00, 22.71, 14.15; HRMS, MALDI+ m/z: calcd for C42H49N2O4: [M + H]+ 645.3692, found: 645.3668.
Id-8. Yield 75.7%, mp 176–179 °C, Rf = 0.27 (CH2Cl2
:
petroleum ether = 2
:
1); IR (KBr, ν, cm−1): 3105, 2925, 2853, 1731, 1647, 1602, 1567, 1494, 1315, 1257, 1074, 844; 1H NMR (500 MHz, CDCl3) (δ ppm): 8.17 (d, J = 8.5 Hz, 4H, C6H4), 8.05 (d, J = 8.5 Hz, 2H, C6H4), 8.01 (d, J = 8.5 Hz, 2H, C6H4), 7.98 (d, J = 15.5 Hz, 1H, CH), 7.46 (d, J = 5.0 Hz, 1H, TpH), 7.41–7.37 (m, 4H, C6H4), 7.12 (m, 1H, TpH), 6.99 (d, J = 8.5 Hz, 2H, C6H4), 4.06 (t, J = 6.5 Hz, 2H, CH2), 1.87–1.80 (m, 2H, CH2), 1.50–1.46 (m, 2H, CH2), 1.41–1.24 (m, 8H, CH2), 0.90 (t, J = 7.0 Hz, 3H, CH3); 13C NMR (125 MHz, CDCl3) (δ ppm): 189.17, 164.56, 163.79, 154.81, 153.78, 152.56, 140.34, 137.68, 132.41, 129.48, 129.12, 128.45, 124.49, 123.01, 122.62, 114.42, 68.40, 31.82, 29.34, 29.24, 29.11, 26.00, 22.67, 14.12; HRMS, MALDI+ m/z: calcd for C34H35N2O4S: [M + H]+ 567.2318, found: 567.2299.
Id-12. Yield 76%, mp 149–158 °C, Rf = 0.29 (CH2Cl2
:
petroleum ether = 2
:
1); IR (KBr, ν, cm−1): 3128, 2954, 2919, 2849, 1736, 1652, 1596, 1510, 1469, 1289, 1260, 1081, 844; 1H NMR (500 MHz, CDCl3) (δ ppm): 8.17 (d, J = 8.5 Hz, 4H, C6H4), 8.05 (d, J = 8.5 Hz, 2H, C6H4), 8.02 (d, J = 8.5 Hz, 2H, C6H4), 7.98 (d, J = 15.5 Hz, 1H, CH), 7.45 (d, J = 5 Hz, 1H, TpH), 7.41–7.37 (m, 4H, CH, TpH, C6H4), 7.12 (t, J = 4.5 Hz, 1H, TpH), 7.00 (d, J = 9.0 Hz, 2H, C6H4), 4.05 (t, J = 6.5 Hz, 2H, OCH2), 1.85–1.80 (m, 2H, CH2), 1.51–1.45 (m, 2H, CH2), 1.37–1.27 (m, 16H, CH2), 0.89 (t, J = 7.0 Hz, 3H, CH3); 13C NMR (125 MHz, CDCl3) (δ ppm): 189.15, 164.57, 163.79, 154.81, 153.78, 150.16, 140.34, 139.61, 137.66, 132.41, 129.48, 129.12, 128.46, 124.48, 123.01, 122.61, 121.12, 120.65, 114.41, 68.40, 31.94, 29.67, 29.61, 29.58, 29.37, 29.11, 26.00, 22.71, 14.14; HRMS, MALDI+ m/z: calcd for C38H43N2O4S: [M + H]+ 623.2944, found 623.2926; elemental analysis: calcd for C38H42N2O4S: C 73.28, H 6.80, N 4.50%; found: C 72.89, H 6.76, N 4.43%.
Id-14. Yield 73%, mp 158–160 °C, Rf = 0.305 (CH2Cl2
:
petroleum ether = 2
:
1); IR (KBr, ν, cm−1): 3078, 2954, 2918, 2849, 1735, 1652, 1596, 1510, 1469, 1290, 1259, 1081, 852; 1H NMR (500 MHz, CDCl3) (δ ppm): 8.17 (d, J = 8.5 Hz, 4H, C6H4), 8.05 (d, J = 8.5 Hz, 2H, C6H4), 8.02 (d, J = 8.5 Hz, 2H, C6H4), 7.98 (d, J = 15.5 Hz, 1H, CH), 7.45 (d, J = 5 Hz, 1H, TpH), 7.41–7.37 (m, 4H, CH, TpH, C6H4), 7.12 (t, J = 4.5 Hz, 1H, TpH), 7.00 (d, J = 9.0 Hz, 2H, C6H4), 4.06 (t, J = 6.5 Hz, 2H, ArH), 1.86–1.80 (m, 2H, CH2), 1.49–1.47 (m, 2H, CH2), 1.37–1.27 (m, 20H, CH2), 0.88 (t, J = 7.0 Hz, 3H, CH3); 13C NMR (125 MHz, CDCl3) (δ ppm): 189.16, 164.58, 163.78, 154.80, 153.78, 150.16, 140.34, 137.68, 132.41, 129.49, 129.13, 128.46, 124.49, 123.01, 122.62, 120.64, 114.41, 68.40, 31.95, 29.69, 29.67, 29.61, 29.57, 29.38, 29.11, 26.00, 22.72, 14.15; HRMS, MALDI+ m/z: calcd for C40H47N2O4S: [M + H]+ 651.3257, found: 651.3243; elemental analysis: calcd for C40H46N2O4S: C 73.81%, H 7.12, N 4.30%; found: C 73.65, H 7.11, N 4.24%.
IIc-8. Yield 53.1%, mp 71–73 °C, Rf = 0.2 (CH2Cl2
:
petroleum ether
:
ethyl acetate = 2 mL
:
1 mL
:
20 μL); IR (KBr, ν, cm−1): 3066, 2923, 2853, 2360, 1729, 1656, 1595, 1569, 1496, 1193, 1033, 983; 1H NMR (500 MHz, CDCl3) (δ ppm): 8.19 (d, J = 8.5 Hz, 2H), 8.06 (d, J = 8.5 Hz, 2H), 8.04 (d, J = 8.5 Hz, 2H), 7.87 (d, J = 15.5 Hz, 1H), 7.70–7.67 (m, 2H), 7.59 (d, J = 15.5 Hz, 1H), 7.47–7.38 (m, 7H), 4.11–4.03 (m, 6H), 1.87–1.76 (m, 6H), 1.53–1.47 (m, 6H), 1.38–1.26 (m, 24H), 0.91–0.87 (m, 9H); 13C NMR (125 MHz, CDCl3) (δ ppm): 189.82, 164.71, 163.66, 154.76, 153.70, 153.34, 153.04, 151.57, 150.58, 150.34, 150.24, 147.02, 145.37, 143.27, 139.75, 134.79, 130.76, 129.59, 129.03, 128.57, 124.52, 123.42, 123.04, 122.54, 122.19, 121.95, 111.07, 108.64, 74.17, 73.63, 69.31, 31.92, 31.68, 31.88, 31.87, 31.84, 31.82, 30.31, 30.20, 29.52, 29.47, 29.43, 29.36, 29.34, 29.31, 29.30, 29.27, 26.09, 26.02, 26.01, 22.69, 14.12. HRMS, MALDI+ m/z: calcd for C52H68N2NaO6: [M + Na]+ 839.4975, found: 839.4939.
IIc-14. Yield 53.6%, mp 87–90 °C, Rf = 0.29 (CH2Cl2
:
petroleum ether
:
ethyl acetate = 3 mL
:
1 mL
:
20 μL); IR (KBr, ν, cm−1): 3063, 2920, 2850, 1744, 1658, 1576, 1495, 1261, 1011, 879.80; 1H NMR (500 MHz, CDCl3) (δ ppm): 8.19 (d, J = 8.5 Hz, 2H), 8.06 (d, J = 8.5 Hz, 2H), 8.03 (d, J = 8.5 Hz, 2H), 7.87 (d, J = 15.5 Hz, 1H), 7.70–7.68 (m, 2H), 7.59 (d, J = 15.5 Hz, 1H), 7.46–7.43 (m, 5H), 7.38 (s, 1H), 4.12–4.02 (m, 6H), 1.87–1.75 (m, 6H), 1.53–1.45 (m, 6H), 1.40–1.26 (m, 60H), 0.91–0.86 (m, 9H); 13C NMR (125 MHz, CDCl3) (δ ppm): 189.83, 163.67, 154.77, 153.35, 153.04, 151.58, 150.59, 150.35, 147.03, 145.38, 139.76, 134.80, 130.76, 129.59, 129.04, 128.58, 124.52, 123.43, 123.04, 122.60, 122.53, 122.24, 122.02, 111.10, 108.61, 74.31, 74.22, 73.61, 69.32, 69.31, 69.28, 31.96, 31.95, 31.92, 31.84, 31.82, 30.48, 30.35, 30.26, 30.25, 29.58, 29.57, 29.48, 29.46, 29.45, 29.36, 29.35, 29.34, 29.33, 29.26, 26.16, 26.10, 26.08, 26.05, 22.69, 14.12. HRMS, MALDI+ m/z: calcd for C70H104N2NaO6: [M + Na]+ 1091.7792, found: 1901.7763.
IId-8. Yield 53.1%, mp 90–91 °C, Rf = 0.2 (CH2Cl2
:
petroleum ether
:
ethyl acetate = 3 mL
:
1 mL
:
20 μL); IR (KBr, ν, cm−1): 3072, 2922, 2854, 1727, 1651, 1586, 1498, 1283, 1028, 942; 1H NMR (500 MHz, CDCl3) (δ ppm): 8.17 (d, J = 8.5 Hz, 2H), 8.06 (d, J = 8.5 Hz, 2H), 8.02 (d, J = 8.5 Hz, 2H), 8.00 (d, J = 15.5 Hz, 1H), 7.46 (d, J = 5.0 Hz, 1H, TpH), 7.44–7.37 (m, 6H), 7.13–7.11 (m, 1H), 4.11–4.04 (m, 6H), 1.87–1.75 (m, 6H), 1.53–1.47 (m, 6H), 1.40–1.26 (m, 24H), 0.91–0.87 (m, 9H); 13C NMR (125 MHz, CDCl3) (δ ppm): 189.11, 164.71, 163.66, 154.77, 153.70, 153.34, 153.04, 151.58, 150.59, 147.02, 143.27, 140.33, 139.65, 137.69, 132.41, 129.49, 129.13, 128.46, 124.52, 123.48, 123.04, 122.64, 122.53, 120.62, 111.08, 108.65, 74.31, 73.63, 69.32, 31.92, 31.83, 30.37, 30.32, 30.21, 29.53, 29.47, 29.44, 29.39, 29.37, 29.35, 29.32, 29.30, 29.27, 26.10, 26.07, 26.03, 26.02, 22.72, 22.69, 14.12. HRMS, MALDI+ m/z: calcd for C50H66N2NaO6S: [M + Na]+ 845.4538, found: 845.4539.
III-8. Yield 71%, mp 186–187 °C, Rf = 0.2 (CH2Cl2
:
petroleum ether
:
ethyl acetate = 3 mL
:
1 mL
:
20 μL); IR (KBr, ν, cm−1): 3060, 2954, 2922, 2852, 1744, 1658, 1629, 1572, 1511, 1266, 1084, 979; 1H NMR (500 MHz, CDCl3) (δ ppm): 8.24 (d, J = 7.5 Hz, 2H), 8.17 (d, J = 8.5 Hz, 2H), 8.07 (d, J = 8.5 Hz, 2H), 8.03 (d, J = 8.5 Hz, 2H), 7.83 (d, J = 15.5 Hz, 1H), 7.68 (t, J = 7.5 Hz, 1H), 7.63 (d, J = 8.5 Hz, 2H), 7.55 (t, J = 7.5 Hz, 2H), 7.45 (d, J = 15.5 Hz, 1H), 7.42 (d, J = 8.5 Hz, 2H), 6.95 (d, J = 8.5 Hz, 2H), 4.02 (t, J = 6.5 Hz, 2H), 1.85–1.78 (m, 2H), 1.50–1.44 (m, 2H), 1.38–1.30 (m, 8H), 0.89 (t, J = 6.8 Hz, 3H); 13C NMR (125 MHz, CDCl3) (δ ppm): 189.87, 164.83, 161.53, 154.64, 153.52, 150.31, 145.37, 140.15, 133.90, 130.40, 130.29, 129.48, 129.21, 128.70, 127.28, 124.51, 122.97, 122.54, 119.45, 114.99, 68.25, 31.82, 29.35, 29.25, 29.17, 26.03, 22.68, 14.12. HRMS, MALDI+ m/z: calcd for C36H37N2O4: [M + H]+ 561.2753, found: 561.2728.
III-14. Yield 70.6%, mp 165–166 °C, Rf = 0.2 (CH2Cl2
:
petroleum ether
:
ethyl acetate = 3 mL
:
1 mL
:
20 μL), IR (KBr, ν, cm−1): 3042, 2954, 2922, 2852, 1744, 1658, 1629, 1572, 1511, 1266, 1084, 979.70; 1H NMR (500 MHz, CDCl3) (δ ppm): 8.24 (d, J = 7.0 Hz, 2H), 8.17 (d, J = 8.5 Hz, 2H), 8.07 (d, J = 8.5 Hz, 2H), 8.03 (d, J = 8.5 Hz, 2H), 7.83 (d, J = 15.5 Hz, 1H), 7.68 (t, J = 7.5 Hz, 1H), 7.62 (d, J = 8.5 Hz, 2H), 7.55 (t, J = 7.5 Hz, 2H), 7.46 (d, J = 15.5 Hz, 1H), 7.42 (d, J = 8.5 Hz, 2H), 6.94 (d, J = 8.5 Hz, 2H), 4.01 (t, J = 6.5 Hz, 2H), 1.84–1.78 (m, 2H), 1.51–1.43 (m, 2H), 1.36–1.26 (m, 20H), 0.88 (t, J = 6.9 Hz, 3H); 13C NMR (125 MHz, CDCl3) (δ ppm): 189.87, 164.83, 161.53, 154.64, 150.31, 145.37, 133.90, 130.35, 129.48, 128.70, 127.28, 124.51, 122.97, 122.54, 119.45, 114.99, 77.28, 77.03, 76.77, 68.25, 31.82, 29.45–29.03, 26.03, 22.68, 14.12. HRMS, MALDI+ m/z: calcd for C42H49N2NaO4: [M + Na]+ 667.3506, found: 667.3519.
IV-8. Yield 42.5%, mp 172–177 °C, Rf = 0.17 (CH2Cl2
:
petroleum ether
:
ethyl acetate = 2 mL
:
1 mL
:
20 μL); IR (KBr, ν, cm−1): 3072, 2955, 2922, 2851, 1729, 1656, 1597, 1511, 1262, 1070, 985; 1H NMR (500 MHz, CDCl3) (δ ppm): 8.17 (d, J = 8.5 Hz, 4H), 8.06 (d, J = 9.0 Hz, 2H), 8.02 (d, J = 8.5 Hz, 2H), 7.83 (d, J = 15.5 Hz, 1H), 7.63 (d, J = 8.5 Hz, 2H), 7.46 (d, J = 15.5 Hz, 1H), 7.40 (d, J = 9.0 Hz, 2H), 6.99 (d, J = 9.0 Hz, 2H), 6.95 (d, J = 8.5 Hz, 2H), 4.06 (t, J = 6.5 Hz, 2H), 4.02 (t, J = 6.5 Hz, 2H), 1.86–1.78 (m, 4H), 1.50–1.44 (m, 4H), 1.39–1.26 (m, 16H), 0.91–0.88 (m, 6H). 13C NMR (125 MHz, CDCl3) (δ ppm): 189.85, 163.78, 161.52, 154.67, 153.74, 150.17, 145.34, 140.09, 132.41, 130.40, 129.47, 127.28, 124.46, 122.95, 122.61, 121.13, 119.45, 114.99, 114.41, 77.29, 77.03, 76.78, 68.32, 31.82, 29.22, 26.02, 22.68, 14.13. HRMS, MALDI+ m/z: calcd for C44H53N2O5: [M + H]+ 689.3954, found: 689.3922.
IV-10. Yield 42.7%, mp 149–150 °C, Rf = 0.18 (CH2Cl2
:
petroleum ether
:
ethyl acetate = 2 mL
:
1 mL
:
20 μL); IR (KBr, ν, cm−1): 3064, 2955, 2920, 2851, 1737, 1658, 1628, 1573, 1510, 1258, 1080, 989; 1H NMR (500 MHz, CDCl3) (δ ppm): 8.17 (d, J = 8.0 Hz, 4H), 8.06 (d, J = 9.0 Hz, 2H), 8.02 (d, J = 8.5 Hz, 2H), 7.83 (d, J = 15.5 Hz, 1H), 7.63 (d, J = 8.5 Hz, 2H), 7.46 (d, J = 15.5 Hz, 1H), 7.40 (d, J = 8.5 Hz, 2H), 6.99 (d, J = 9.0 Hz, 2H), 6.95 (d, J = 8.5 Hz, 2H), 4.06 (t, J = 6.5 Hz, 2H), 4.01 (t, J = 6.5 Hz, 2H), 1.86–1.78 (m, 4H), 1.51–1.44 (m, 4H), 1.36–1.28 (m, 24H), 0.90–0.87 (m, 6H); 13C NMR (125 MHz, CDCl3) (δ ppm): 189.86, 163.78, 161.52, 154.67, 153.74, 145.34, 132.41, 130.40, 129.47, 127.28, 124.46, 122.95, 122.61, 121.12, 119.46, 114.99, 114.41, 77.28, 77.03, 76.78, 68.32, 31.91, 29.57, 29.36, 29.14, 26.01, 22.70, 14.14. HRMS, MALDI+ m/z: calcd for C48H61N2O5: [M + H]+ 745.4580, found: 745.4545.
IV-12. Yield 43%, mp 147–148 °C, Rf = 0.20 (CH2Cl2
:
petroleum ether
:
ethyl acetate = 2 mL
:
1 mL
:
20 μL); IR (KBr, ν, cm−1): 3068, 2955, 2919, 2850, 1737, 1659, 1603, 1573, 1510, 1260, 1081, 989. 1H NMR (500 MHz, CDCl3) (δ ppm): 8.17 (d, J = 8.0 Hz, 4H), 8.05 (d, J = 9.0 Hz, 2H), 8.02 (d, J = 8.5 Hz, 2H), 7.83 (d, J = 15.5 Hz, 1H), 7.63 (d, J = 8.5 Hz, 2H), 7.46 (d, J = 15.5 Hz, 1H), 7.40 (d, J = 8.5 Hz, 2H), 6.99 (d, J = 9.0 Hz, 2H), 6.94 (d, J = 8.5 Hz, 2H), 4.06 (t, J = 6.5 Hz, 2H), 4.01 (t, J = 6.5 Hz, 2H), 1.86–1.78 (m, 4H), 1.51–1.43 (m, 4H), 1.37–1.27 (m, 32H), 0.89 (t, J = 6.5 Hz, 6H); 13C NMR (125 MHz, CDCl3) (δ ppm): 189.85, 164.57, 163.78, 161.52, 154.67, 153.74, 150.17, 145.34, 140.09, 132.41, 130.40, 129.47, 127.28, 124.46, 122.95, 122.61, 121.12, 119.45, 114.98, 114.41, 77.29, 77.03, 76.78, 68.32, 31.94, 29.63, 29.37, 29.14, 26.01, 22.71, 14.15. HRMS, MALDI+ m/z: calcd for C52H69N2O5: [M + H]+ 801.5206, found: 801.5189.
IV-14. Yield 42.6%, mp 140–144 °C, Rf = 0.23 (CH2Cl2
:
petroleum ether
:
ethyl acetate = 2 mL
:
1 mL
:
20 μL); IR (KBr, ν, cm−1): 3062, 2955, 2918, 2849, 1736, 1659, 1603, 1568, 1258, 1082.04, 978. 1H NMR (500 MHz, CDCl3) trans isomer >85% (δ ppm): 8.17 (d, J = 8.0 Hz, 4H), 8.05 (d, J = 8.5 Hz, 2H), 8.01 (d, J = 8.5 Hz, 2H), 7.82 (d, J = 16.0 Hz, 1H), 7.63 (d, J = 8.5 Hz, 2H), 7.46 (d, J = 15.5 Hz, 1H), 7.40 (d, J = 9.0 Hz, 2H), 6.99 (d, J = 9.0 Hz, 2H), 6.94 (d, J = 8.5 Hz, 2H), 4.06 (t, J = 6.5 Hz, 2H), 4.01 (t, J = 6.5 Hz, 2H), 1.86–1.78 (m, 4H), 1.51–1.44 (m, 4H), 1.37–1.24 (m, 40H), 0.88 (t, J = 6.5 Hz, 6H). 13C NMR (125 MHz, CDCl3) (δ ppm): 189.84, 164.52 (s), 163.79, 161.57, 154.57, 153.74, 150.17, 145.32, 140.08, 132.38, 130.42, 129.48, 127.31, 124.42, 122.93, 122.59, 121.12, 119.44, 114.95, 114.46, 77.30, 77.04, 76.77, 68.31, 31.96, 29.64, 29.36, 29.14, 26.03, 22.71, 14.14. HRMS, MALDI+ m/z: calcd for C56H77N2O5: [M + H]+ 857.5832, found: 857.5812.
V. Yield 42.7%, mp 220–224 °C, Rf = 0.18 (CH2Cl2
:
petroleum ether
:
ethyl acetate = 2 mL
:
1 mL
:
20 μL); IR (KBr, ν, cm−1): 3070, 2923, 2852, 1731, 1655, 1598, 1570, 1510, 1246, 1064, 979; 1H NMR (500 MHz, CDCl3) trans isomer >70% (δ ppm): 8.76 (s, 1H), 8.33 (d, J = 9.0 Hz, 1H), 8.27 (d, J = 9.0 Hz, 1H), 8.18 (d, J = 8.5 Hz, 3H), 8.09 (d, J = 8.5 Hz, 2H), 8.03 (d, J = 8.5 Hz, 2H), 7.94 (d, J = 9.0 Hz, 1H), 7.84 (d, J = 15.5 Hz, 1H), 7.63 (d, J = 8.5 Hz, 2H), 7.49–7.44 (m, 3H), 7.39 (d, J = 9.0 Hz, 1H), 7.26 (s, 1H), 6.95 (d, J = 8.5 Hz, 2H), 4.24 (t, J = 6.5 Hz, 2H), 4.02 (t, J = 6.5 Hz, 2H), 1.95–1.87 (m, 2H), 1.84–1.78 (m, 2H), 1.58–1.53 (m, 2H), 1.49–1.45 (m, 2H), 1.42–1.27 (m, 16H), 0.90 (t, J = 6.5 Hz, 6H); 13C NMR (125 MHz, CDCl3) (δ ppm): 189.84, 164.79, 161.53, 154.60, 145.36, 140.15, 132.32, 130.40, 129.56, 127.28, 126.84, 124.62, 124.21, 122.98, 122.58, 119.43, 115.58, 114.99, 77.29, 77.03, 76.78, 70.03, 68.25, 31.83, 29.28, 25.99, 22.68, 14.13. HRMS, MALDI+ m/z: calcd for C48H55N2O5: [M + H]+ 739.4111, found: 739.4108.
Conflicts of interest
There are no conflicts to declare.
Acknowledgements
We thank the National Natural Science Foundation of China (NSFC, 21562032), Natural Science Foundation of Inner Mongolia (2017MS0205) and Research Program of Science and Technology at Universities of Inner Mongolia (NJZZ001) for their generous financial support.
References
- S. Laschat, A. Baro, N. Steinke, F. Giesselmann, C. Hägele, G. Scalia, R. Judele, E. Kapatsina, S. Sauer, A. Schreivogel and M. Tosoni, Angew. Chem., 2007, 119, 4916 CrossRef.
- G. Lee, R. J. Carlton, F. Araoka, N. L. Abbott and H. Takezoe, Adv. Mater., 2013, 25, 245 CrossRef CAS PubMed.
- A. M. Lowe and N. L. Abbott, Chem. Mater., 2012, 24, 746 CrossRef CAS PubMed.
- A. D. Price and D. K. Schwartz, J. Am. Chem. Soc., 2008, 130, 8188 CrossRef CAS PubMed.
- S. Dixit and R. A. Vora, Mol. Cryst. Liq. Cryst., 2015, 623, 56 CrossRef CAS.
- Z. Jiang, M. Xu, F. Li and Y. Yu, J. Am. Chem. Soc., 2013, 135, 16446 CrossRef CAS PubMed.
- G. Hegde, G. Shanker, S. M. Gan, A. R. Yuvaraj, S. Mahmood and U. K. Mandal, Liq. Cryst., 2016, 43, 1578 CrossRef CAS.
- J. Wang, Y. Shi, K. Yang, J. Wei and J. Guo, RSC Adv., 2015, 5, 67357 RSC.
- M. L. Rahman, T. K. Biswas, S. M. Sarkar, M. M. Yusoff, M. N. F. A. Malek and C. Tschierske, J. Mol. Liq., 2015, 202, 125 CrossRef CAS.
- I. Niezgoda, J. Jaworska and Z. Galewski, J. Mol. Liq., 2016, 222, 571 CrossRef CAS.
- N. G. Nagaveni, M. Gupta, A. Royb and V. Prasad, J. Mater. Chem., 2010, 20, 9089 RSC.
- N. Trišović, J. Antanasijević, T. Tóth-Katona, M. Kohout, M. Salamonczyk, S. Sprunt, A. Jákli and K. Fodor-Csorba, RSC Adv., 2015, 5, 64886 RSC.
- N. G. Nagaveni, A. Royb and V. Prasad, J. Mater. Chem., 2012, 22, 8948 RSC.
- S. H. Ryu, M. Gim, W. Lee, S. W. Choi and D. K. Yoon, ACS Appl. Mater. Interfaces, 2017, 9, 3186 CAS.
- D. Y. Kim, S. A. Lee, H. Kim, S. M. Kim, N. Kimc and K. U. Jeong, Chem. Commun., 2015, 51, 11080 RSC.
- A. Zep, K. Sitkowska, D. Pociecha and E. Gorecka, J. Mater. Chem. C, 2014, 2, 2323 RSC.
- H. Y. Zhong, L. Chen, R. Yang, Z. Y. Meng, X. M. Ding, X. F. Liu and Y. Z. Wang, J. Mater. Chem. C, 2017, 5, 3306 RSC.
- E. Verploegen, J. Soulages, M. Kozberg, T. Zhang, G. McKinley and P. Hammond, Angew. Chem., Int. Ed., 2009, 48, 3494 CrossRef CAS PubMed.
- J. Wang, C. Lin, J. Zhang, J. Wei, Y. Song and J. Guo, J. Mater. Chem. C, 2015, 3, 4179 RSC.
- R. Prasath, P. Bhavana, S. W. Ng and E. R. T. Tiekink, J. Organomet. Chem., 2013, 726, 62 CrossRef CAS.
- S. Attar, Z. O'Brien, H. Alhaddad, M. L. Golden and A. Calderón-Urrea, Bioorg. Med. Chem., 2011, 19, 2055 CrossRef CAS PubMed.
- C. A. Calliste, J. C. Le Bail, P. Trouilas, C. Poug, A. J. Chulia and L. J. Doroux, Anticancer Res., 2001, 21, 3949 CAS.
- X. Wu, E. R. T. Tiekink, I. Kostetski, N. Kocherginsky, A. L. C. Tan, S. B. Khoo, P. Wilairat and M. L. Go, Eur. J. Pharm. Sci., 2006, 27, 175 CrossRef CAS PubMed.
- P. S. Patil, S. M. Dharmaprakash, K. Ramakrishna and H. K. Fun, J. Cryst. Growth, 2007, 303, 520 CrossRef CAS.
- H. Zhao, X. Zhu, D. Wang, S. Chen and Z. Bian, Aust. J. Chem., 2015, 68, 1035 CrossRef CAS.
- R. L. Coelho, E. Westphal, D. Z. Mezalira and H. Gallardo, Liq. Cryst., 2017, 44, 405 CAS.
- H. T. Srinivasa and S. Kumar, Liq. Cryst., 2017, 44, 1506 CrossRef CAS.
- A. Karuppusamy, V. Ramkumar, P. Kannan, S. Balamurugan and S. M. Said, Soft Mater., 2017, 15, 132 CrossRef CAS.
- B. B. Jain and R. B. Patel, Mol. Cryst. Liq. Cryst., 2016, 638, 27 CrossRef CAS.
- N. R. Muniya and V. R. Patel, Mol. Cryst. Liq. Cryst., 2016, 638, 95 CrossRef CAS.
- A. S. Pandey, R. Dhar, A. S. Achalkumar and C. V. Yelamaggad, Liq. Cryst., 2011, 38, 775 CrossRef CAS.
- J. Li, W. Zhang, Y. Zhao and J. Pu, Adv. Mater. Res., 2012, 380, 326 CrossRef CAS.
- D. Jayalatha, R. Balamurugan and P. Kannan, High Perform. Polym., 2009, 21, 139 CrossRef CAS.
- H. Zhao, X. Zhu, Y. Shang, S. Chen, B. Li and Z. Bian, RSC Adv., 2016, 6, 34476 RSC.
- C. Selvarasu and P. Kannan, J. Mol. Struct., 2016, 1125, 234 CrossRef CAS.
- T. Ogata, K. Oikawa, T. Fujisawa, S. Motoyama, T. Izumi, A. Kasahara and N. Tanaka, Bull. Chem. Soc. Jpn., 1981, 54, 3723 CrossRef CAS.
- S. Y. Kim, O. N. Kadkin, E. H. Kim and M. G. Choi, J. Organomet. Chem., 2011, 696, 2429 CrossRef CAS.
- G. Casella, V. Causin, F. Rastrelli and G. Saielli, Liq. Cryst., 2016, 43, 1161 CrossRef CAS.
- I. Cârlescu, A. M. Scutar, D. Apreutesei, V. Alupei and D. Scutaru, Liq. Cryst., 2007, 34, 775 CrossRef.
Footnote |
† Electronic supplementary information (ESI) available: Detailed procedures of synthesis and characterization of compounds VIa–VIe, Tables SI–SII, Fig. S1–S30. See DOI: 10.1039/c7ra06958h |
|
This journal is © The Royal Society of Chemistry 2017 |
Click here to see how this site uses Cookies. View our privacy policy here.