DOI:
10.1039/C7RA06933B
(Paper)
RSC Adv., 2017,
7, 40015-40019
Sumalactones A–D, four new curvularin-type macrolides from a marine deep sea fungus Penicillium Sumatrense†
Received
21st June 2017
, Accepted 24th July 2017
First published on 16th August 2017
Abstract
Sumalactones A–D (1–4), four new curvularin-type macrolides, together with two known analogues, curvularin (5) and dehydrocurvularin (6), were isolated from Penicillium Sumatrense, a marine fungus isolated from deep-sea sediments. Sumalactones C (3) and D (4) are unprecedented curvularin-type macrolides bearing a rare 11-membered macrolide skeleton. Their structures were elucidated on the basis of intensive spectroscopic analysis. The absolute configurations of compounds 1–4 were determined by CD spectra and modified Mosher's method. Compound 6 showed significant inhibition activity towards LPS-induced nitric oxide production in RAW 264.7 macrophages with IC50 value of 0.91 μM.
Introduction
Among fungal macrolides, resorcinylic acid lactones (RALs) and dihydroxyphenylacetic acid lactones (DALs) belong to a unique family of naturally occurring homologous macrolides, which are characterized by possessing a macrolide core structure fused to a resorcinol aromatic ring.1 Curvularins, featuring a substituted resorcinol fragment fused to the β, γ-positions of the macrocyclic lactone ring, are produced by a number of fungal species mainly from the genera Aspergillus,2 Alternaria,3 Astragalus,4 Curvularia,5 Cochliobolus,6 and Penicillium7 with diverse biological activities. They are biogenetically derived from the polyketide synthase pathways in bacterial and fungi, and have brought great interest and challenges for total synthesis and biosynthesis studies.8
In the course of our ongoing research on new bioactive secondary metabolites from marine fungi,9 Penicillium sumatrense was isolated from a deep-sea sediment sample (−2500 m depth) of the Indian Ocean, which resulted in the isolation of four new curvularin derivatives, sumalactones A–D (1–4) with 10- or 11- or 12-membered macrolide skeletons, as well as two known compounds curvularin (5)10 and dehydrocurvularin (6).11 Compounds 3 and 4 with 11-membered macrolides skeleton are considered rare in nature and haven't been reported before. All the compounds were evaluated for their inhibitory effect on the production of nitric oxide (NO) induced by lipopolysaccharide (LPS) in RAW 264.7 macrophages. Herein we report the isolation, structure elucidation, and biological activities of these compounds (Fig. 1).
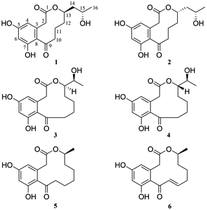 |
| Fig. 1 Chemical structures of 1–6. | |
Results and discussion
Sumalactone A (1) gave an HRESIMS ion peak at m/z 307.1197 [M − H]−, corresponding to the molecular formula C16H20O6, which required seven degrees of unsaturation. The UV spectrum showed absorption maxima at 204, 218, 269, and 297 nm. The 1H signals (Table 1) suggested a pair of meta-coupled aromatic protons at δH 6.12 (d, J = 1.8 Hz, H-4), and 6.24 (d, J = 2.0 Hz, H-6) and one methyl group at δH 1.12 (3H, d, J = 6.2 Hz, CH3-16). Analysis of 13C NMR and DEPT spectra data (Table 1) together with the HSQC data indicated the presence of six sp2 quaternary carbons including one ketone carbonyl carbon (δC 211.6) and one ester carbonyl carbon (δC 171.8), two sp2 methine carbons (δC 110.6 and 102.4), two sp3 oxygenated methine carbons (δC 76.3 and 65.5), five sp3 methylene carbons, and one methyl carbon (δC 23.9). The 1H and 13C NMR data were nearly identical to curvularin (5),10 except that C-13 methylene carbon in 5 was replaced by an oxygenated methine carbon (δC 76.3) in 1, which was confirmed by the 1H–1H COSY correlations between H-13 (δH 4.91) and H2-12 (δH 1.47 and 1.95). In addition, the key HMBC correlation from H-13 (δH 4.91) to ester carbonyl carbon C-1 (δC 171.8) indicated the location of the 10-membered lactone between C-1 and C-13. Thus, the planar structure of 1 was established (Fig. 2). In order to determine the absolute configuration of OH group at C-15, the modified Mosher's method was applied.12 When reacted with (R)- and (S)-MTPA chloride, 1 gave the corresponding (S)- and (R)-MTPA esters, respectively. The observed chemical shift differences ΔδH(S–R) (Fig. 3) clearly defined the S configuration at C-15. The absolute configuration of C-13 was determined by CD spectrum (Fig. 4). The positive Cotton effect at approximately 265 nm and negative Cotton effect at 316 nm of 1 suggested the R configuration at C-13, comparing to that of xestodecalactone A with opposite sign.7c,13 Therefore, the absolute configuration of 1 was established as 13R, 15S.
Table 1 1H NMR (500 MHz) and 13C NMR (125 MHz) spectroscopic data of 1–4 in CD3OD (δ in ppm, J in Hz)
No. |
1 |
2 |
3 |
4 |
δC |
δH |
δC |
δH |
δC |
δH |
δC |
δH |
1 |
171.8 |
|
172.7 |
|
172.4 |
|
172.5 |
|
2 |
41.3 |
3.44, d (18.3) |
41.3 |
3.49, d (18.5) |
41.1 |
3.46, d (17.2) |
41.1 |
3.50, d (17.1) |
3.96, d (18.4) |
3.98, d (18.4) |
4.14, d (17.1) |
4.11, d (17.2) |
3 |
136.3 |
|
136.2 |
|
136.3 |
|
136.3 |
|
4 |
110.6 |
6.12, d (1.8) |
110.6 |
6.13, d (1.6) |
111.5 |
6.16, d (2.2) |
111.7 |
6.17, d (2.2) |
5 |
161.0 |
|
161.0 |
|
160.9 |
|
160.9 |
|
6 |
102.4 |
6.24, d (2.0) |
102.4 |
6.24, d (2.0) |
102.6 |
6.25, d (2.2) |
102.6 |
6.24, d (2.2) |
7 |
158.8 |
|
158.9 |
|
158.8 |
|
158.7 |
|
8 |
122.6 |
|
122.6 |
|
122.0 |
|
122.0 |
|
9 |
211.6 |
|
211.5 |
|
209.8 |
|
209.9 |
|
10 |
46.7 |
2.70, m |
46.6 |
2.70, ddd (2.1, 7.7, 15.9) |
41.7 |
2.87, ddd (3.4, 6.1, 17.5) |
41.8 |
2.86, ddd (3.3, 6.0, 17.3) |
3.06, m |
3.06, ddd (2.2, 10.5, 15.4) |
3.17, ddd (3.1, 11.6, 17.4) |
3.19, ddd (3.2, 11.6, 17.3) |
11 |
23.4 |
1.87, 2H, m |
23.4 |
1.87, 2H, overlapped |
23.9 |
1.47, overlapped |
23.9 |
1.47, overlapped |
1.89, m |
1.89, m |
12 |
35.4 |
1.47, overlapped |
36.3 |
1.47, overlapped |
22.0 |
1.23, m |
22.1 |
1.23, m |
1.95, m |
1.87, overlapped |
1.66, m |
1.67, overlapped |
13 |
76.3 |
4.91, m |
76.3 |
4.90, m |
26.1 |
1.47, overlapped |
26.3 |
1.47, overlapped |
1.75, m |
1.67, overlapped |
14 |
45.3 |
1.47, overlapped |
45.9 |
1.47, overlapped |
79.8 |
4.75, ddd (3.3, 5.1, 10.5) |
79.9 |
4.78, ddd (2.7, 6.0, 10.2) |
1.70, ddd (6.6, 7.5, 14.1) |
1.56, ddd (3.8, 7.9, 14.2) |
15 |
65.5 |
3.69, m |
65.1 |
3.65, m |
69.4 |
3.63, dq (5.3, 6.3) |
69.2 |
3.62, p (6.4) |
16 |
23.9 |
1.12, d (6.2) |
23.6 |
1.09, d (6.2) |
18.7 |
0.98, d (6.4) |
18.9 |
1.02, d (6.5) |
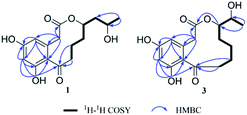 |
| Fig. 2 Key 1H–1H COSY and HMBC correlations of 1 and 3. | |
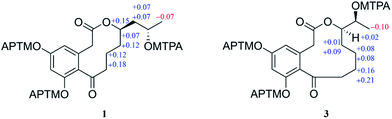 |
| Fig. 3 Δδ(δS − δR) values (in ppm) for the MTPA esters of 1 and 3. | |
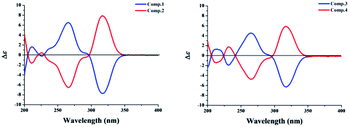 |
| Fig. 4 CD spectra of 1–4. | |
Sumalactone B (2) was isolated as a yellow oil and it was determined to be C16H20O6 on the basis of negative HRESIMS, indicating that 2 is isomeric to 1. Indeed, analysis of the NMR data indicated that compound 2 had the same planer structure as 1. Considering that the only difference observed between 2 and 1 was the completely opposite CD curves (Fig. 4), we suggest that 2 is epimeric at C-13 relative to compound 1. Consequently, the absolute configuration of 2 was assigned as 13S, 15S.
Sumalactone C (3) was obtained as a yellow oil, for which the molecular formula was assigned as C16H20O6 by HRESIMS, from a [M − H]− ion at 307.1191 (calc. 307.1182). The UV absorptions together with 1H and 13C NMR data indicated the curvularin-type macrolide skeleton similar to that of compound 1. The 1H–1H COSY correlations between H2-13/H-14/H-15/H3-16, and HMBC correlation from H-14 (δH 4.75) to C-1 (δC 172.4), confirmed the 11-membered macrolide in compound 3, which was rare in nature and was first example of oxygenation at C-14 in curvularin skeleton. The absolute configuration at C-14 was assigned to be R because of the almost identical CD spectrum to that of 1 (Fig. 4). The OH group at C-15 was determined as S by the modified Mosher's method (Fig. 3). Therefore, the absolute configuration of 3 was established as 14R, 15S.
Sumalactone D (4) was attributed the molecular formula C16H20O6 from its HRESIMS at m/z 307.1014 ([M − H]− 307.1014, calc. 307.1182). On analysis of its 1H and 13C NMR spectra, similar features to that of 3 were evident, but with an opposite CD curve (Fig. 4). Therefore, the absolute configuration at C-14 was assigned as S. Thus, the absolute configuration of 4 was assigned as 14S, 15S.
In addition to compounds 1–4, the known curvularin (5)10 and dehydrocurvularin (6)11 were also isolated and identified from the fungal strain P. sumatrense MCCC 3A00612. All isolated compounds were tested for inhibitory activities against LPS-induced NO production in RAW 264.7 macrophages. As the results, only compound 6 showed significant NO production inhibitory activity with IC50 of 0.91 ± 0.03 μM, which was comparable
to
that
of the
positive control L-NMMA (IC50 of 41.91 ± 1.27 μM). The cell viability measured by the MTS assay showed that compound 6 had no significant cytotoxicity to the RAW 264.7 cells at the effective concentration for the inhibition of NO production.
Experimental section
General experimental procedures
Optical rotations were measured on an MCP 300 polarimeter, and UV spectra were measured on a U-2910 spectrometer. IR spectra were measured on an Affinity-1 FT-IR spectrometer. The CD spectra were recorded in MeOH using a Chirascan spectropolarimeter at room temperature. HRESIMS spectra were obtained on Waters Synapt G2 TOF mass spectrometer. The NMR data were acquired with a Bruker AV 500 NMR spectrometer using solvent signals (CD3OD: δH 3.30/δC 49.0) as standards. Column chromatography (CC) was carried out on Sephadex LH-20 (Pharmacia, USA), and ODS (60–80 μm, YMC). TLC was performed on silica gel plate (SGF254, 0.2 mm, Merck, Germany). Analytical and semi-preparative HPLC were performed on an Agilent HPLC system equipped with a G1311B pump, a G1329B automated sample injector, a G1316A column compartment, and a G1315D diode array detector using a Phenomenex Kinetex C18 column (4.6 × 250 mm, 5 μm), a Waters T3 C18 column (4.6 × 250 mm, 5 μm) and a Phemomenex Kinetex C18 column (10.0 × 250 mm, 5 μm).
Fungus material
The fungus P. sumatrense MCCC 3A00612 was isolated from deep-sea sediments collected from the Indian Ocean. The strain was identified by Dr Zongze Shao, and a voucher specimen (P. sumatrense MCCC 3A00612) has been deposited in the Marine Culture Collection of China.
Extraction and isolation
The fresh mycelia of P. sumatrense were grown on PDA medium at 28 °C for 4 days. Agar plugs were cut into small pieces and were selected to inoculate 10 Erlenmeyer flasks (500 mL) each containing 200 mL of PDB. The seed cultures were incubated at 28 °C on a rotary shaker (150 rpm) for 5 days and were then inoculated into 60 × 500 mL conical flasks on rice solid medium (80 g rice, 0.36 g sea salt, and 120 mL filtered water) for 30 days at 28 °C. The fermented cultures were extracted with 70% acetone/water, and evaporated under reduced pressure to afford an aqueous solution, which was then extracted three times with EtOAc and afforded the EtOAc extract (10.4 g). The EtOAc extract (10.4 g) was fractionated by silica gel column chromatography (CC) eluting with CHCl3–MeOH (100
:
0, 95
:
5, 98
:
2, 9
:
1, 8
:
2, 1
:
1, and 0
:
100, v/v) to afford seven fractions (J1–J7). Fraction J5 (877.8 mg) was further subjected to Sephadex LH-20 CC using CHCl3–MeOH (1
:
1, v/v) to afford four subfractions (J5-1 to J5-4). Subfraction J5-3 (374.0 mg) was separated on a ODS column with a gradient of MeOH–H2O (10
:
90, 30
:
70, 50
:
50, 70
:
30, and 100
:
0, v/v) to give three portions (J5-3-1 to J5-3-3). J5-3-1 (167.2 mg) was separated on semi-preparative HPLC using CH3CN–H2O (15
:
85, v/v) to give five portions (J5-3-1-1 to J5-3-1-5). J5-3-1-1 (9.4 mg) was purified on semi-preparative HPLC by using MeOH–H2O (30
:
70, v/v) to yield 1 (7.3 mg). J5-3-1-2 (9.6 mg) was purified on semi-preparative HPLC by using CH3CN–H2O (22
:
78, v/v) to yield 2 (4.3 mg). J5-3-1-4 (7.4 mg) was purified on semi-preparative HPLC by using MeOH–H2O (30
:
70, v/v) to yield 3 (2.9 mg). J5-3-1-5 (10.6 mg) was purified on semi-preparative HPLC by using MeOH–H2O (30
:
70, v/v) to yield 4 (2.3 mg). J5-3-2 (112.0 mg) was purified on semi-preparative HPLC by using MeOH–H2O (55
:
45, v/v) to yield 5 (8.5 mg). Fraction J3 (4.0 g) was subjected to a ODS column with a gradient of MeOH–H2O (30
:
70, 50
:
50, 70
:
30, and 100
:
0, v/v) to give four subfractions (J3-1 to J3-4). Subfraction J3-2 (519.6 mg) was further separated by ODS CC eluting with MeOH–H2O (50
:
50, v/v) to afford four portions (J3-2-1 to J3-2-4). J3-2-2 (21.0 mg) was purified on semi-preparative HPLC by using MeOH–H2O (50
:
50, v/v) to yield 6 (6.8 mg).
Sumalactone A (1). Yellow oil (MeOH); [α]25D −124.0 (c 0.1, MeOH); UV (MeOH) λmax (log
ε) 204 (4.13), 218 (4.08), 269 (3.76), 297 (3.68) nm; CD (0.81 mM, MeOH) λmax (Δε) 211 (1.61), 226 (−0.22), 266 (6.52), 317 (−7.72) nm; IR (MeOH) νmax 3194, 2968, 2938, 1715, 1663, 1607, 1589, 1472, 1339, 1267, 1161, 1136, 1024, 1007, 845, 669 cm−1; HRESIMS m/z 307.1197 [M − H]− (calcd for C16H19O6, 307.1182); the 1H and 13C NMR data, see Table 1.
Sumalactone B (2). Yellow oil (MeOH); [α]25D +86.1 (c 0.1, MeOH); UV (MeOH) λmax (log
ε) 203 (4.02), 218 (3.94), 268 (3.62), 298 (3.55) nm; CD (0.81 mM, MeOH) λmax (Δε) 210 (−1.61), 226 (0.45), 266 (−6.54), 317 (7.89) nm; IR (MeOH) νmax 3167, 2967, 2932, 1705, 1661, 1605, 1589, 1404, 1339, 1252, 1161, 1089, 1024, 989, 845 cm−1; HRESIMS m/z 307.1193 [M − H]− (calcd for C16H19O6, 307.1182); the 1H and 13C NMR data, see Table 1.
Sumalactone C (3). Yellow oil (MeOH); [α]25D −41.6 (c 0.1, MeOH); UV (MeOH) λmax (log
ε) 203 (4.04), 219 (3.95), 268 (3.61), 297 (3.54) nm; CD (0.81 mM, MeOH) λmax (Δε) 214 (1.30), 231 (−1.90), 265 (4.53), 318 (−6.30) nm; IR (MeOH) νmax 3256, 2934, 1703, 1651, 1607, 1591, 1462, 1335, 1261, 1161, 1088, 1042, 1013, 845, 665 cm−1; HRESIMS m/z 307.1191 [M − H]− (calcd for C16H19O6, 307.1182); the 1H and 13C NMR data, see Table 1.
Sumalactone D (4). Yellow oil (MeOH); [α]25D +35.8 (c 0.1, MeOH); UV (MeOH) λmax (log
ε) 203 (4.04), 219 (3.96), 268 (3.63), 298 (3.54) nm; CD (0.81 mM, MeOH) λmax (Δε) 213 (−1.45), 231 (1.76), 265 (−4.75), 317 (5.87) nm; IR (MeOH) νmax 3304, 2943, 1703, 1651, 1607, 1462, 1337, 1265, 1161, 1040, 993, 847 cm−1; HRESIMS m/z 307.1014 [M − H]− (calcd for C16H19O6, 307.1182); the 1H and 13C NMR data, see Table 1.
Curvularin (5). [α]25D −22.5 (c 0.1, MeOH); literature value [α]25D −28.6 (c 0.4, EtOH).
Dehydrocurvularin (6). [α]25D −45.6 (c 0.1, MeOH); literature value [α]25D −65.9 (c 1.8, EtOH).
NO production bioassay
The murine macrophage cell line RAW 264.7 was obtained from Cell Bank of Chinese Academy of Sciences. RAW 264.7 cells were seeded in 96-well cell culture plates (1.5 × 105 cells per well) and treated with serial dilutions of the compounds with a maximum concentration of 25 μM in triplicate, followed by stimulation with 1 μg mL−1 LPS (Sigma, St. Louis, MO, USA) for 18 h. NO production in the supernatant was assessed by Griess reagents (Reagent A & Reagent B, respectively, Sigma). The absorbance at 570 nm was measured with a microplate reader (Thermo, Waltham, MA, USA). NG-methyl-L-arginine acetate salt (L-NMMA, Sigma), a well-known nitric oxide synthase (NOS) inhibitor, was used as a positive control.14 The viability of RAW 264.7 cells was evaluated by the MTS assay simultaneously to exclude the interference of the cytotoxicity of the test compounds.
Conclusions
In summary, six curvularin-type macrolides belonging to DALs group15 were isolated from P. sumatrense MCCC 3A00612, including four new ones. The curvularins are octaketides composed of a 12-membered macrolide skeleton attached to a 3,5-dihydroxyphenylacetic acid. Compounds 1–4 are the new curvularin-type macrolides with 10- or 11-membered macrolide skeleton, among them, compounds 3 and 4 are the first macrolides with 11-membered macrolide skeleton. Interestingly, compounds 1–4 were isolated as epimers at the lactone carbon. Although the configuration at the lactone carbon is obviously variable among the DALs including the (−)-(15S)-curvularin (compound 5) and (+)-(15R)-curvularin series5 but they were isolated from different sources. However, epimers at the non-lactone carbon including (+)-(11S,15R)-11-hydroxycurvularin and (+)-(11R,15R)-11-hydroxycurvularin,5 as well as (−)-(11R,15S)-11-hydroxycurvularin and (−)-(11S,15S)-11-hydroxycurvularin16 were isolated from same sources. Therefore, the lactone formation does not change the absolute configuration of the lactone carbon, which is established upon generating the secondary hydroxyl group. Different positions of lactone cyclization would produce different ring sizes, which was certainly observed. In addition, compound 6 exhibited potent inhibitory effect on NO production when tested in vitro. High levels of NO are markers at the treatment of inflammatory disorders. Our results suggested that dehydrocurvularin (6) may be a potential candidate for further evaluation on the molecular mechanism of action on specific inflammatory disorders.
Conflicts of interest
There are no conflicts to declare.
Acknowledgements
This work was financially supported by the National Basic Research Program of China (2015CB150600), and the National Natural Science Foundation of China (31470236, 41206130), and the Pearl River Nova Program of Guangzhou (201506010067). We thank Chuan-Rong Li and Ai-Jun Sun for assistance with CD and NMR measurement and Dr Zong-Ze Shao for fungal strain isolation and identification.
References
- J. W. Blunt, B. R. Copp, R. A. Keyzers, M. H. G. Munro and M. R. Prinsep, Nat. Prod. Rep., 2012, 29, 144–222 RSC.
- O. Caputo and F. Viola, Planta Med., 1977, 31, 31–32 CrossRef CAS PubMed.
- D. J. Robeson, G. A. Strobel and R. N. Strange, J. Nat. Prod., 1985, 48, 139–141 CrossRef CAS.
- B. P. Bashyal, W. M. K. Wijeratne, J. Tillotson, A. E. Arnold, E. Chapman and A. A. L. Gunatilaka, J. Nat. Prod., 2017, 80, 427–433 CrossRef CAS PubMed.
- H. Greve, P. J. Schupp, E. Eguereva, S. Kehraus, G. Kelter, A. Maier, H. H. Fiebig and G. M. König, Eur. J. Org. Chem., 2008, 2008, 5085–5092 CrossRef PubMed.
- E. L. Ghisalberti and C. Y. Rowland, J. Nat. Prod., 1993, 56, 2175–2177 CrossRef CAS.
-
(a) C. J. Barrow, J. Nat. Prod., 1997, 60, 1023–1025 CrossRef CAS;
(b) L. H. Meng, X. M. Li, C. T. Lv, C. S. Li, G. M. Xu, C. G. Huang and B. G. Wang, J. Nat. Prod., 2013, 76, 2145–2149 CrossRef CAS PubMed;
(c) R. A. Edrada, M. Heubes, G. Brauers, V. Wray, A. Berg, U. Gräfe, M. Wohlfarth, J. Mühlbacher, K. Schaumann, G. Bringmann and P. Proksch, J. Nat. Prod., 2002, 65, 1598–1604 CrossRef CAS PubMed.
-
(a) H. Choe, T. T. Pham, J. Y. Lee, M. Latif, H. Park, Y. K. Kang and J. Lee, J. Org. Chem., 2016, 81, 2612–2617 CrossRef CAS PubMed;
(b) P. M. Tadross, S. C. Virgil and B. M. Stoltz, Org. Lett., 2010, 12, 1612–1614 CrossRef CAS PubMed;
(c) Y. Xu, T. Zhou, P. Espinosa-Artiles, Y. Tang, J. Zhan and I. Molnár, ACS Chem. Biol., 2014, 9, 1119–1127 CrossRef CAS PubMed;
(d) Q. R. Liang, Y. Q. Sun, B. X. Yu, X. G. She and X. F. Pan, J. Org. Chem., 2007, 72, 9846–9849 CrossRef CAS PubMed.
-
(a) F. He, J. Bao, X. Y. Zhang, Z. T. Tu, Y. M. Shi and S. H. Qi, J. Nat. Prod., 2013, 76, 1182–1186 CrossRef CAS PubMed;
(b) F. He, Z. Liu, J. Yang, P. Fu, J. Peng, W. M. Zhu and S. H. Qi, Tetrahedron Lett., 2012, 53, 2280–2283 CrossRef CAS;
(c) F. He, Z. Han, J. Peng, P. Y. Qian and S. H. Qi, Nat. Prod. Commun., 2013, 8, 329–332 CAS;
(d) F. He, Y. L. Sun, K. S. Liu, X. Y. Zhang, P. Y. Qian, Y. F. Wang and S. H. Qi, J. Antibiot., 2012, 65, 109–111 CrossRef CAS PubMed;
(e) Z. Zhang, X. Min, J. Huang, Y. Zhong, Y. Wu, X. Li, Y. Deng, Z. Jiang, L. Zhang and F. He, Mar. Drugs, 2016, 14, 233 CrossRef PubMed.
- E. L. Ghisalberti, D. C. R. Hockless, C. Y. Rowland and A. H. White, Aust. J. Chem., 1993, 46, 571–575 CrossRef CAS.
- A. Kobayashi, T. Hino, S. Yata, T. J. Itoh, H. Sato and K. Kawazu, Agric. Biol. Chem., 1988, 52, 3119–3123 CAS.
-
(a) T. Kusumi, Y. Fujita, I. Ohtani and H. Kakisawa, Tetrahedron Lett., 1991, 32, 2923–2926 CrossRef CAS;
(b) B. N. Su, E. J. Park, Z. H. Mbwambo, B. D. Santarsiero, A. D. Mesecar, H. H. S. Fong, J. M. Pezzuto and A. D. Kinghorn, J. Nat. Prod., 2002, 65, 1278–1282 CrossRef CAS PubMed.
-
(a) G. Bringmann, G. Lang, M. Michel and M. Heubes, Tetrahedron Lett., 2004, 45, 2829–2831 CrossRef CAS;
(b) T. Yoshino, F. Ng and S. J. Danishefsky, J. Am. Chem. Soc., 2006, 128, 14185–14191 CrossRef CAS PubMed;
(c) Q. Liang, J. Zhang, W. Quan, Y. Sun, X. She and X. Pan, J. Org. Chem., 2007, 72, 2694–2697 CrossRef CAS PubMed.
- D. W. Reif and S. A. Mccreedy, Arch. Biochem. Biophys., 1995, 320, 170–176 CrossRef CAS PubMed.
-
(a) J. Xu, C. S. Jiang, Z. L. Zhang, W. Q. Ma and Y. W. Guo, Acta Pharmacol. Sin., 2014, 35, 316–330 CrossRef CAS PubMed;
(b) Y. Xu, T. Zhou, Z. Zhou, S. Su, S. A. Roberts, W. R. Montfort, J. Zhang, M. Chen, W. Zhang, M. Lin, J. Zhan and I. Molnár, Proc. Natl. Acad. Sci. U. S. A., 2013, 110, 5398–5498 CrossRef CAS PubMed.
- S. Lai, Y. Shizuri, S. Yamamura, K. Kawai, Y. Terada and H. Furukawa, Tetrahedron Lett., 1989, 30, 2241–2244 CrossRef CAS.
Footnotes |
† Electronic supplementary information (ESI) available: Spectroscopic data of 1–4, preparation of (S)- and (R)-MTPA esters of 1 and 3, and the 1D and 2D NMR spectra of 1–4. See DOI: 10.1039/c7ra06933b |
‡ These authors have contributed equally to this work. |
|
This journal is © The Royal Society of Chemistry 2017 |