DOI:
10.1039/C7RA06240K
(Paper)
RSC Adv., 2017,
7, 34315-34320
Preparation and characterization of novel phthalhydrazide-functionalized MCM-41 and its application in the one-pot synthesis of coumarin-fused triazolopyrimidines†
Received
4th June 2017
, Accepted 21st June 2017
First published on 10th July 2017
Abstract
Phthalhydrazide-functionalized MCM-41 (P-MCM-41) was prepared and characterized, and its catalytic activity was evaluated. MCM-41 and 3-chloropropyl trimethylsilyl-MCM-41 (CPS-MCM-41) were prepared according to methods previously reported in the literature. Then CPS-MCM-41 was reacted with phthalhydrazide to yield the desired catalyst. The obtained base catalyst was characterized using SEM, EDX, XRD and FT-IR. No leaching problem was observed after several runs, while the catalyst was recovered and reused without a loss of reactivity under the described reaction conditions. Phthalhydrazide-functionalized MCM-41 was employed as a reusable base catalyst for the synthesis of coumarin-fused triazolopyrimidines via a three-component condensation of 4-hydroxycoumarin, 3-amino-1H-1,2,4-triazole and aromatic aldehydes.
Introduction
Mesoporous triazolopyrimidine materials have drawn tremendous attention because they can be employed as reusable catalysts, nano-reactors, and adsorbents.1–4 These many applications result from the unique three-dimensional structures, high surface areas, tunable and uniform pore channels and large volumes of these materials.5 MCM-41 is a well-ordered mesoporous material with controllable pore sizes and forms close-packed silica-coated micellar surfactants.6 However, pure MCM-41 has a low intrinsic acidity and weak hydrogen-bonding sites.7 Modification and functionalization of the surface of MCM-41 with organic species such as imidazole, piperazine and mercaptopyrimidine have been shown to improve its catalytic activity by increasing its hydrophobicity and providing it with various properties.8–10 Accordingly, we set out to use phthalhydrazide as a donor ligand and mild base for these purposes.
4-Hydroxycoumarin has attracted significant interest as a structural subunit of many natural products, pharmaceuticals and biologically active compounds.11,12 Furthermore, 4-hydroxycoumarin and its derivatives have been used in many chemical and agricultural applications,13 as well as in the dye and liquid crystal industries,14,15 because of their conjugated structures. Also, they can be found in some drugs with potential anti-HIV,16 bacteriostatic, anticoagulant, spasmolytic, and antifungal activity with few side effects and little toxicity.17–19
Triazolopyrimidines are among the most important hybrid heterocycles of pyrimidine as they are structural elements of various bioactive natural products.20 Also, they are useful compounds for medicinal chemists.21,22 They have been used as important and useful starting materials for the synthesis of other fused heterocyclic systems.23 Triazolopyrimidines have been used in the synthesis of some herbicidal drugs, such as penoxsulam, diclosulam, flumetsulam, azafenidin, and floransulan.24,25
Due to these benefits, and as a continuation of our previous work on the applications of heterogeneous catalysts for the development of new synthetic methodologies,26–30 we here set out to accomplish the following two aims: the preparation of phthalhydrazide-functionalized MCM-41 (P-MCM-41) as a novel reusable base catalyst (Scheme 1), and study of the applicability of this catalyst for the synthesis of coumarin-fused triazolopyrimidines via a novel three-component reaction of 3-amino-1H-1,2,4-triazole 1, aromatic aldehydes 2, and 4-hydroxycoumarin 3 (Scheme 2).
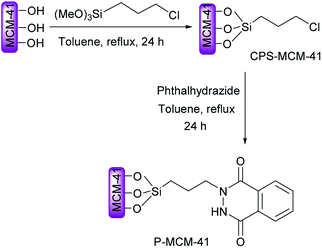 |
| Scheme 1 Preparation of phthalhydrazide-MCM-41 (P-MCM-41). | |
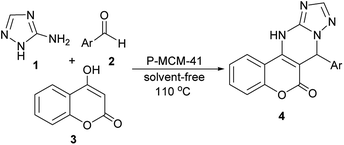 |
| Scheme 2 Synthesis of coumarin-fused triazolopyrimidines in the presence of P-MCM-41. | |
Experimental
The chemicals used were purchased from Merck and Aldrich chemical companies. The reactions were monitored by using thin layer chromatography (TLC) (silica-gel 60 F 254, hexane:EtOAc). Fourier transform infrared (FT-IR) spectra were recorded on a Shimadzu-470 spectrometer, using KBr pellets. Melting points were determined on a KRUSS model instrument. 1H NMR and 13C NMR spectra were recorded with a Bruker 400 Ultrashield1, with DMSO-d6 as the solvent and TMS as the internal standard. X-ray diffraction (XRD) patterns were obtained by using a Philips X Pert Pro X diffractometer operated with Ni-filtered Cu-Kα radiation. The particle size and morphology of the samples were studied by carrying out scanning electron microscopy (SEM: KYKY-EM3200) experiments under an acceleration voltage of 26 kV.
Preparation of MCM-41
Cetyltrimethylammonium bromide (CTAB) (5 g) was dissolved in an NH4OH solution (1250 mL, 1.1 M) at room temperature. Then, tetraethoxysilane (TEOS) (20 mL) was poured into the solution, stirred for 30 min, and then kept at room temperature for 72 hours. The solid product was filtered and washed with water and dried overnight at room temperature. The final step involved calcinating the sample at 550 °C for 48 h to remove the organic template.31
Preparation of 3-chloropropyl trimethylsilyl-MCM-41 (CPS-MCM-41)
MCM-41 (1 g), 3-chloropropyl trimethoxysilane (5 mmol), and dry toluene (10 mL) were added into a round-bottom flask under reflux with stirring in an argon atmosphere for 24 h. The result of this reaction was cooled to room temperature, filtered, and then washed with hot toluene. The subsequent product was dried overnight under vacuum at 80 °C.32,33
Immobilization of phthalhydrazide on CPS-MCM-41
CPS-MCM-41 (1 g) was added to a solution of phthalhydrazide (1.5 mmol) in dry toluene and refluxed for 24 h under an argon atmosphere. Then the mixture was filtered and washed with hot ethanol and dried overnight under vacuum to afford phthalhydrazide-MCM-41 (P-MCM-41) as a white powder.32,33
General procedure for the synthesis of coumarin-fused triazolopyrimidines 4
A mixture of 3-amino-1H-1,2,4-triazole (1 mmol), aryl aldehyde (1 mmol), 4-hydroxycoumarin (1 mmol), and P-MCM-41 (0.02 g) was stirred and heated at 110 °C in a preheated oil bath for 10–25 min. After completion of the reaction as indicated by TLC (EtOAc/hexane), the mixture was dissolved in hot ethanol, the catalyst was separated by filtration, and the solvent was removed under vacuum. The pure product was obtained after recrystallization from EtOH. The catalyst was washed with MeOH, dried at 100 °C for 45 min, and reused in another reaction.
Compound 4a. IR (KBr) νmax = 3320, 1701, 1600, 1521, 1078 cm−1; 1H NMR (400 MHz, DMSO-d6): δ = 9.50 (s, 1H), 8.43 (s, 1H), 7.88 (d, J = 7.2 Hz, 2H), 7.74 (t, J = 7.2 Hz, 1H), 7.65 (t, J = 7.2 Hz, 2H), 7.36–7.41 (m, 4H), 5.99 (s, 1H) ppm; 13C NMR (100 MHz, DMSO-d6): δ = 159.34, 156.10, 152.66, 149.07, 147.47, 137.01, 129.90, 128.78, 128.42, 125.87, 125.43, 116.89, 116.87, 113.43, 99.03, 58.44 ppm; anal. calcd for C18H12N4O2: C, 68.35; H, 3.82; N, 17.71. Found: C, 68.36; H, 3.84; N, 17.70.
Compound 4b. IR (KBr) νmax = 3164, 1700, 1608, 1527, 1106 cm−1; 1H NMR (400 MHz, DMSO-d6): δ = 9.75 (s, 1H), 8.32 (s, 1H), 7.76 (d, J = 8.4 Hz, 2H), 7.52–7.56 (m, 4H), 7.34–7.37 (m, 2H), 6.05 (s, 1H) ppm; 13C NMR (100 MHz, DMSO-d6): δ = 161.16, 151.26, 150.22, 149.90, 149.02, 148.01, 132.42, 130.55, 129.78, 128.77, 127.83, 125.52, 124.68, 116.82, 116.75, 112.87, 99.16, 53.09 ppm; anal. calcd for C18H11N5O4: C, 59.84; H, 3.07; N, 19.38. Found: C, 59.85; H, 3.09; N, 19.37.
Compound 4c. IR (KBr) νmax = 3245, 1701, 1598, 1529, 1187 cm−1; 1H NMR (400 MHz, DMSO-d6): δ = 9.46 (s, 1H), 8.66 (s, 1H), 8.10 (t, J = 7.6 Hz, 1H), 7.86 (s, 1H), 7.60–7.67 (m, 5H), 7.18 (d, J = 7.6 Hz, 1H), 5.98 (s, 1H) ppm; 13C NMR (100 MHz, DMSO-d6): δ = 160.23, 153.21, 152.14, 149.77, 148.23, 147.78, 138.33, 134.53, 130.66, 127.79, 122.33, 120.63, 116.33, 116.21, 112.17, 99.15, 56.74 ppm; anal. calcd for C18H11N5O4: C, 59.84; H, 3.07; N, 19.38. Found: C, 59.85; H, 3.08; N, 19.39.
Compound 4d. IR (KBr) νmax = 3369, 1702, 1602, 1562, 1108 cm−1; 1H NMR (400 MHz, DMSO-d6): δ = 9.82 (s, 1H), 8.61 (s, 1H), 8.48 (d, J = 6.4 Hz, 1H), 8.07 (d, J = 9.6 Hz, 1H), 7.60–7.67 (m, 2H), 7.35 (d, J = 8.4 Hz, 2H), 7.18 (d, J = 8.4 Hz, 2H), 6.08 (s, 1H) ppm; 13C NMR (100 MHz, DMSO-d6): δ = 160.01, 152.94, 150.95, 149.76, 147.95, 144.66, 143.46, 130.62, 129.44, 125.85, 123.41, 117.44, 117.35, 112.02, 99.08, 57.49 ppm; anal. calcd for C18H11N5O4: C, 59.84; H, 3.07; N, 19.38. Found: C, 59.83; H, 3.09; N, 19.40.
Compound 4e. IR (KBr) νmax = 3220, 1729, 1710 cm−1; 1H NMR (400 MHz, DMSO-d6): δ = 9.15 (s, 1H), 8.25 (s, 1H), 7.48–7.52 (m, 4H), 7.39 (d, J = 7.2 Hz, 1H), 7.23 (t, J = 7.2 Hz, 2H), 7.00 (d, J = 6.8 Hz, 1H), 6.07 (s, 1H), 2.62 (s, 3H) ppm; 13C NMR (100 MHz, DMSO-d6): δ = 159.89, 153.04, 151.86, 149.60, 148.30, 138.03, 132.38, 130.55, 129.68, 128.59, 128.08, 125.05, 116.65, 116.54, 111.94, 102.18, 98.87, 56.63, 19.01 ppm; anal. calcd for C19H14N4O2: C, 69.08; H, 4.27; N, 16.96. Found: C, 69.07; H, 4.28; N, 16.97.
Compound 4f. IR (KBr) νmax = 3214, 1732, 1702, 1643 cm−1; 1H NMR (400 MHz, DMSO-d6): δ = 9.24 (s, 1H), 8.27 (s, 1H), 7.40 (d, J = 8 Hz, 1H), 7.34 (s, 1H), 7.27 (t, J = 8 Hz, 1H), 7.17 (d, J = 8 Hz, 1H), 7.02–7.09 (m, 4H), 6.08 (s, 1H), 2.62 (s, 3H) ppm; 13C NMR (100 MHz, DMSO-d6): δ = 160.23, 154.14, 152.77, 148.23, 147.97, 138.34, 137.34, 130.12, 128.73, 128.66, 126.66, 125.33, 124.13, 116.73, 116.62, 112.17, 99.15, 56.74, 24.49 ppm; anal. calcd for C19H14N4O2: C, 69.08; H, 4.27; N, 16.96. Found: C, 69.08; H, 4.28; N, 16.95.
Compound 4g. IR (KBr) νmax = 3214, 1731, 1693 cm−1; 1H NMR (400 MHz, DMSO-d6): δ = 9.49 (s, 1H), 7.85 (s, 1H), 7.65–7.69 (m, 4H), 7.09 (d, J = 8 Hz, 2H), 7.03 (d, J = 8 Hz, 2H), 6.09 (s, 1H), 2.62 (s, 3H) ppm; 13C NMR (100 MHz, DMSO-d6): δ = 160.02, 153.76, 153.10, 147.99, 146.72, 135.83, 134.62, 128.93, 128.82, 124.29, 123.08, 116.24, 116.17, 112.12, 99.12, 56.66, 24.49 ppm; anal. calcd for C19H14N4O2: C, 69.08; H, 4.27; N, 16.96. Found: C, 69.07; H, 4.27; N, 16.97.
Compound 4h. IR (KBr) νmax = 3106, 1728, 1708 cm−1; 1H NMR (400 MHz, DMSO-d6): δ = 10.91 (s, 1H), 8.25 (s, 1H), 7.86 (s, 1H), 7.61–7.63 (m, 4H), 7.32 (d, J = 8.8 Hz, 1H), 7.04 (d, J = 8.8 Hz, 1H), 5.34 (s, 1H) ppm; 13C NMR (100 MHz, DMSO-d6): δ = 161.78, 153.05, 151.14, 149.86, 148.22, 136.16, 133.30, 132.19, 131.98, 129.00, 128.32, 126.07, 125.32, 116.14, 116.03, 112.02, 98.91, 56.02 ppm; anal. calcd for C18H10Cl2N4O2: C, 56.13; H, 2.62; N, 14.55. Found: C, 56.12; H, 2.63; N, 14.56.
Results and discussion
P-MCM-41 was prepared and several characterization techniques were utilized to determine the structure of the synthesized catalyst. The XRD patterns of the MCM-41 and functionalized MCM-41 are shown in Fig. 1. The powder X-ray diffractograms exhibited three peaks indicative of an ordered hexagonal MCM-41 structure (Fig. 1a).34 Compared to the main peak from MCM-41, that of CPS-MCM-41 was slightly less intense (Fig. 1b) and that of P-MCM-41 was much less intense (Fig. 1c), and there was also a shift towards a larger diffraction angle. These results were due to the decreased order of the mesoporous sample resulting from the incorporation of 3-chloropropyl trimethoxysilane and phthalhydrazide into the framework of the MCM-41 and a decrease in the pore size.34 Nevertheless, the XRD results showed that the mesoporous hexagonal channels were still present after modification.
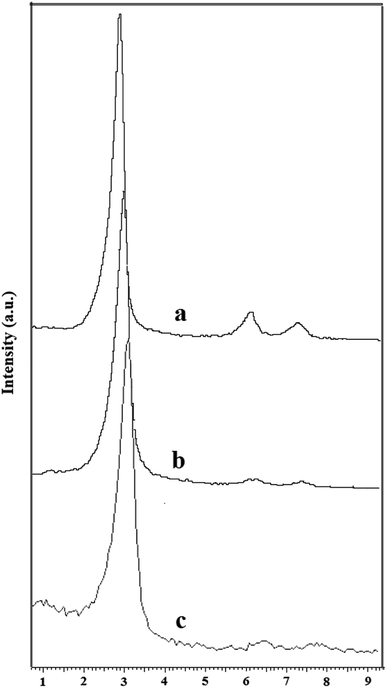 |
| Fig. 1 XRD patterns of (a) MCM-41, (b) CPS-MCM-41, and (c) P-MCM-41. | |
The EDX spectra of CPS-MCM-41 (Fig. 2a) and P-MCM-41 (Fig. 2b) confirmed them to include chlorine and nitrogen, and hence indicated that 3-chloropropyl trimethoxysilane and phthalhydrazide grafted successfully on the mesoporous silica.
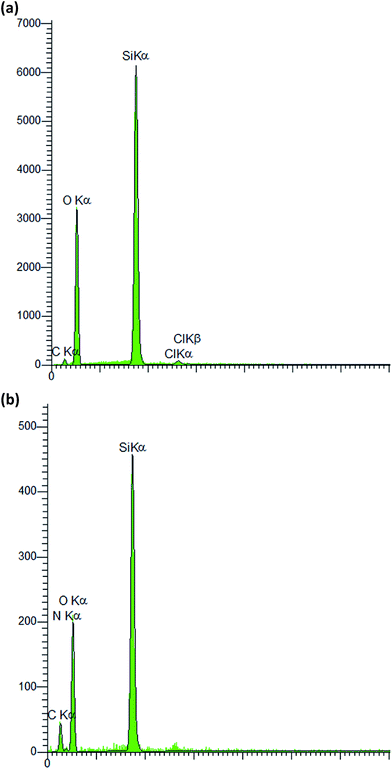 |
| Fig. 2 (a) EDX spectrum of CPS-MCM-41. (b) EDX spectrum of P-MCM-41. | |
The morphology of the P-MCM-41 nanocatalyst was investigated by acquiring a scanning electron microscopy (SEM) image of it, as shown in Fig. 3. Spherical nanoparticles with a narrow distribution of diameters averaging approximately 49 nm were observed in this image.
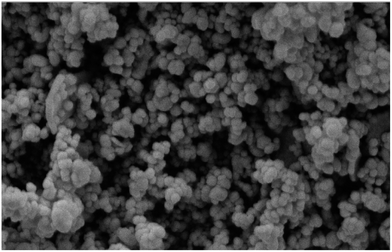 |
| Fig. 3 SEM image of phthalhydrazide-functionalized MCM-41. | |
Fig. 4 shows FT-IR spectra of MCM-41, CPT-MCM-41, P-MCM-41, and phthalhydrazide. Characteristic asymmetric and symmetric Si–O–Si stretching vibrations at 1083 cm−1 and 806 cm−1 along with a Si–OH vibration at 958 cm−1 and bending Si–O–Si band at 460 cm−1 were observed for MCM-41. The bands at about 3450 cm−1 and 1642 cm−1 were related to H-bonded hydroxyl stretching and adsorbed water bending vibrations. After modification of MCM-41 with 3-chloropropyl trimethoxysilane, new bands appeared at 2934 and 2840 cm−1, which corresponded to aliphatic C–H vibrations. In addition, bands at 3164 cm−1, 1660 cm−1 and 3016 cm−1 appeared, corresponding to N–H, carbonyl, and aromatic C–H groups, respectively, and these bands hence provided evidence for the presence of phthalhydrazide on the surface of the grafted materials.
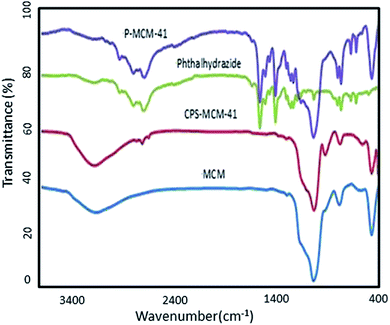 |
| Fig. 4 FT-IR spectra of MCM-41, CPS-MCM-41, phthalhydrazide, and P-MCM-41. | |
After characterizing the MCM-41 catalyst and its derivatives, we investigated the use of phthalhydrazide-functionalized MCM-41 in the synthesis of coumarin-fused triazolopyrimidines for the ultimate purpose of developing novel applications of the catalyst. To determine suitable conditions, a reaction between 3-amino-1H-1,2,4-triazole, benzaldehyde, and 4-hydroxycoumarin was selected as a model reaction. By screening loading of the catalyst and considering the effect of the solvent and temperature on the reaction rate, it was found that as little as 0.02 g of P-MCM-41 was sufficient to produce the desired product at 110 °C under solvent-free conditions (Table 1).
Table 1 Optimization of the reaction conditionsa
Entry |
Catalyst (g) |
Solvent |
T (°C) |
Yieldb (%) |
Reaction conditions: 3-amino-1H-1,2,4-triazole (1 mmol), benzaldehyde (1 mmol), 4-hydroxycoumarin (1 mmol), 2 h. Isolated yields. |
1 |
— |
— |
25 |
— |
2 |
— |
— |
80 |
10 |
3 |
Phthalhydrazide (5 mol%) |
— |
80 |
5 |
4 |
Phthalhydrazide (10 mol%) |
— |
80 |
5 |
5 |
P-MCM-41 (0.003) |
|
80 |
50 |
6 |
P-MCM-41 (0.005) |
— |
80 |
55 |
7 |
P-MCM-41 (0.01) |
— |
80 |
65 |
8 |
P-MCM-41 (0.02) |
— |
80 |
75 |
9 |
P-MCM-41 (0.03) |
— |
80 |
70 |
10 |
P-MCM-41 (0.02) |
MeOH (5 mL) |
65 |
75 |
11 |
P-MCM-41 (0.02) |
H2O (5 mL) |
100 |
65 |
12 |
P-MCM-41 (0.02) |
CHCl3 (5 mL) |
60 |
63 |
13 |
P-MCM-41 (0.02) |
CH3CN (5 mL) |
80 |
72 |
14 |
P-MCM-41 (0.02) |
— |
90 |
80 |
15 |
P-MCM-41 (0.02) |
— |
100 |
80 |
16 |
P-MCM-41 (0.02) |
— |
110 |
95 |
17 |
P-MCM-41 (0.02) |
— |
120 |
95 |
With the optimized reaction conditions in hand, we investigated the scope of this reaction with a wide range of aromatic aldehydes (Table 2).
Table 2 P-MCM-41-catalyzed preparation of coumarin-fused triazolopyrimidinesa
Entry |
Productb |
Mp (°C) |
Yieldc (%) |
Reaction conditions: 3-amino-1H-1,2,4-triazole (1 mmol), arylaldehydes (1 mmol), 4-hydroxycoumarin (1 mmol), and P-MCM-41 (0.02 g), 110 °C. All products are novel and fully characterized by elemental analysis, FT-IR, 1H NMR and 13C NMR spectroscopy. Isolated yield. |
1 |
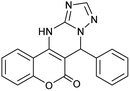 |
221–222 |
95 |
2 |
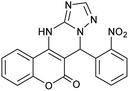 |
177–179 |
90 |
3 |
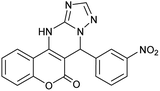 |
205–207 |
91 |
4 |
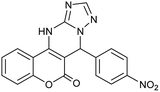 |
211–212 |
94 |
5 |
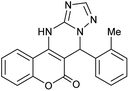 |
263–265 |
87 |
6 |
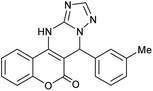 |
199–201 |
98 |
7 |
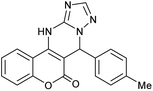 |
235–237 |
89 |
8 |
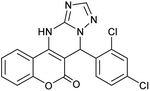 |
281–283 |
85 |
Although the detailed mechanism of the reaction was not very clear, a possible mechanism was proposed, as shown in Scheme 3. According to this proposal, the reaction proceeds via the Knoevenagel condensation of an aromatic aldehyde and 4-hydroxycoumarin to produce 5 in the presence of P-MCM-41 as a mild base catalyst. Compound 5 can then be attacked by 3-amino-1H-1,2,4-triazole to form intermediate 6 through a Michael addition. Finally, according to the proposed mechanism, the product is obtained after intramolecular cyclization and then dehydration of 7.
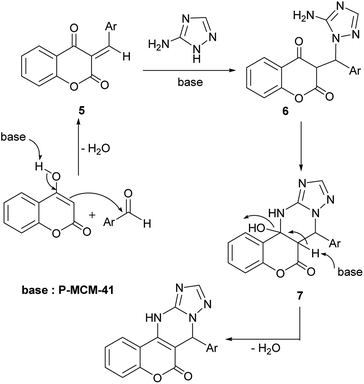 |
| Scheme 3 Proposed mechanism for the synthesis of 4 in the presence of P-MCM-41 as a catalyst. | |
Because the recoverability and recycling of the catalyst are important issues from both practical and economical viewpoints, we next investigated the reusability of P-MCM-41 in the reaction of 3-amino-1H-1,2,4-triazole, benzaldehyde and 4-hydroxycoumarin under the optimized reaction conditions. P-MCM-41 was successfully regenerated from the model reaction by washing it with MeOH and drying it at 120 °C. The result showed that the catalyst can be recovered and reused in six reaction cycles with only a gradual decrease of the reaction yield (Fig. 5).
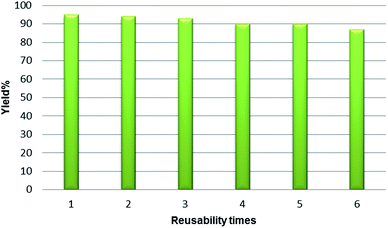 |
| Fig. 5 Reusability of the P-MCM-41 catalyst in the reaction of 3-amino-1H-1,2,4-triazole, benzaldehyde and 4-hydroxycoumarin. | |
Conclusions
In summary, we prepared and characterized P-MCM-41, and applied this catalyst to the reaction between 4-hydroxycoumarin, various aromatic aldehydes and 3-amino-1H-1,2,4-triazole to synthesize coumarin-fused triazolopyrimidine derivatives. The development of a new practical approach to novel useful heterocycles, the high yields of the products, the solvent-free reaction conditions and the short reaction times are the main advantages of this method. In addition, the catalyst could be recovered and reused at least six times with no decrease in its activity.
Acknowledgements
The authors gratefully acknowledge partial support of this work by Yasouj University, Yasouj, Iran.
Notes and references
- I. Muylaert, A. Verberckmoes, J. De Decker and P. Van Der Voort, Adv. Colloid Interface Sci., 2012, 175, 39 CrossRef CAS PubMed.
- A. Taguchi and F. Schuth, Microporous Mesoporous Mater., 2005, 77, 1 CrossRef CAS.
- B. Karimi and D. Zareyee, Org. Lett., 2008, 10, 3989 CrossRef CAS PubMed.
- Z. Wu and D. Zhao, Chem. Commun., 2011, 47, 3332 RSC.
- E. P. Ng and S. Mintova, Microporous Mesoporous Mater., 2008, 114, 1 CrossRef CAS.
- C. Gunathilake, M. S. Kadanapitiye, O. Dudarko, S. D. Huang and M. Jaroniec, ACS Appl. Mater. Interfaces, 2015, 7, 23144 CAS.
- J. Połtowicz, K. Pamin, L. Matachowski, E. M. Serwicka, R. Mokaya, Y. Xia and Z. Olejniczak, Catal. Today, 2006, 114, 287 CrossRef.
- P. Kamala and A. Pandurangan, Catal. Commun., 2008, 9, 605 CrossRef CAS.
- X. S. Zhaoand and G. Q. Lu, J. Phys. Chem., 1998, 102, 1556 Search PubMed.
- R. S. Robert, A. Rafael, A. D. James and W. R. Thatcher, Microporous Mesoporous Mater., 2003, 66, 53 CrossRef.
- M. E. Riveiro, N. De Kimpe, A. Vázquez, R. Moglioni, F. Monczor and C. Davio, Curr. Med. Chem., 2010, 17, 1325 CrossRef CAS PubMed.
- M. Joao Matos, S. Vazquez-Rodriguez, L. Santana, E. Uriarte, C. Fuentes-Edfuf, Y. Santos and A. Munoz-Crego, Med. Chem., 2012, 8, 1140 Search PubMed.
- G. L. Xi and Z. Q. Liu, J. Agric. Food Chem., 2015, 63, 3516 CrossRef CAS PubMed.
- V. F. Traven, A. V. Manaev, A. Y. Bochkov, T. A. Chibisova and I. V. Ivanov, Russ. Chem. Bull., 2012, 61, 1342 CrossRef CAS.
- X. M. Peng, G. L. Damu and C. Zhou, Curr. Pharm. Des., 2013, 19, 3884 CrossRef CAS PubMed.
- M. Rajabi, Z. Hossaini, M. A. Khalilzadeh, S. Datta, M. Halder and S. A. Mousa, J. Photochem. Photobiol., A, 2015, 148, 66 CrossRef CAS PubMed.
- I. Kostova, S. Bhatia, P. Grigorov, S. Balkansky, V. S. Parmar, A. K. Prasad and L. Saso, Curr. Med. Chem., 2011, 18, 3929 CrossRef CAS PubMed.
- Y. Bansal, P. Sethi and G. Bansal, Med. Chem. Res., 2013, 22, 3049 CrossRef CAS.
- A. Sánchez-Recillas, G. Navarrete-Vázquez, S. Hidalgo-Figueroa, M. Y. Rios, M. Ibarra-Barajas and S. Estrada-Soto, Eur. J. Med. Chem., 2014, 77, 400 CrossRef PubMed.
- M. A. Phillips, R. Gujjar, N. A. Malmquist, J. White, F. El Mazouni, J. Baldwin and P. K. Rathod, J. Med. Chem., 2008, 51, 3649 CrossRef CAS PubMed.
- R. Gujjar, F. El Mazouni, K. L. White, J. White, S. Creason, D. M. Shackleford, X. Deng, W. N. Charman, I. Bathurst and J. Burrows, J. Med. Chem., 2011, 54, 3935 CrossRef CAS PubMed.
- M. A. Phillips and P. K. Rathod, Infect. Disord.: Drug Targets, 2010, 10, 226 CrossRef CAS.
- N. Zhang, S. Ayral-Kaloustian, T. Nguyen, J. Afragola, R. Hernandez, J. Lucas, J. Gibbons and C. Beyer, J. Med. Chem., 2007, 50, 319 CrossRef CAS PubMed.
- Y. C. Liu, R. Y. Qu, Q. Chen, J. F. Yang, C. Niu, Z. Xi and G. F. Yang, J. Agric. Food Chem., 2016, 64, 4845 CrossRef CAS PubMed.
- G. F. Yang, R. J. Lu, X. N. Fei and H. Z. Yang, Chin. J. Chem., 2000, 18, 435 CAS.
- B. Karami, M. Kiani, S. J Hosseini and M. Bahrami, New J. Chem., 2015, 39, 8576 RSC.
- B. Karami, K. H. Eskandari, S. Khodabakhshi, S. J Hosseini and F. Hashemian, RSC Adv., 2013, 3, 23335 RSC.
- B. Karami, M. Farahi, N. Farmani and H. Mohamadi Tanuraghaj, New J. Chem., 2016, 40, 1715 RSC.
- B. Karami, S. Khodabakhshi and S. Karami, Croat. Chem. Acta, 2014, 87, 23 CrossRef CAS.
- B. Karami, V. Ghashghaee and S. Khodabakhshi, Catal. Commun., 2012, 20, 71 CrossRef CAS.
- Y. Wang, Y. Yu, C. Deng, J. Wang and B. T. Zhang, RSC Adv., 2015, 5, 103989 RSC.
- V. Antochshuk and M. Jaroniec, Chem. Commun., 2002, 258 RSC.
- A. Ahmad, H. Md Rasid and K. Kassim, Int. J. Chem. Eng. Appl., 2013, 4, 35 Search PubMed.
- M. Occelli and L. S. Auroux, Appl. Catal., A, 1999, 183, 231 CrossRef CAS.
Footnote |
† Electronic supplementary information (ESI) available. See DOI: 10.1039/c7ra06240k |
|
This journal is © The Royal Society of Chemistry 2017 |