DOI:
10.1039/C7RA06004A
(Paper)
RSC Adv., 2017,
7, 32559-32563
Direct C-2 acylation of indoles with toluene derivatives via Pd(II)-catalyzed C–H activation†
Received
29th May 2017
, Accepted 16th June 2017
First published on 26th June 2017
Abstract
A simple and efficient Pd-catalyzed method for the C2-acylation of indoles is described. Less toxic, stable, and commercially available toluene derivatives were used as acyl sources, with tert-butylhydroperoxide (TBHP) as oxidant and pivalic acid as additive, providing moderate to good yields.
Introduction
Acylated indoles are important structural motifs present in a number of biologically active natural products and serve as valuable intermediates in the synthesis of various dyes and pharmaceuticals.1 Thus, the efficient construction of functionalized acyl indole analogues has been the focus of intense research efforts of synthetic chemists. With the advent of transition metal-catalyzed C–H bond functionalization,2 this methodology has become the most straightforward one leading to functionalized indoles.3 However, the regioselective C-2 functionalization of the indole nucleus in the presence of the electron rich C-3 position, still remains a challenging proposition for organic chemists.4 To override this inherent selectivity, the introduction of a suitable N-protecting group would change the regioselectivity from C-3 to the more electrophilic C-2 position of the indole nucleus.5 Towards this end, considerable progress has been achieved in recent years via metal-catalyzed oxidative cross-couplings (Scheme 1). These processes allow the use of less functionalized starting materials in a minimum number of operational steps.6 In 2012, Li and co-workers developed a Rh-catalyzed oxidative direct C2-acylation of indoles with alkyl and aryl aldehydes through Csp2–H activation.7 Following this work, the group of Zhu disclosed a palladium-catalyzed decarboxylative C2-acylation of indoles with α-oxocarboxylic acids.8 Subsequently, Liang and co-workers described a palladium-catalyzed C2-acylation of indoles with aryl and alkyl aldehydes via C–H functionalization.9 Very recently, a palladium-catalyzed C2-acylation of N-pyrimidyl indoles with α-diketones10a and ethyl glyoxylate10b was reported. Despite the significant progress made in the area of C2-acylation of indoles, there are no reports on the formation of a C–C bond directly from the more challenging and inert Csp3–H bond.11,12 In continuation of our efforts to develop facile methods for the C–H functionalization via radical addition,13 we were interested to use substrates without prefunctionalization for the creation of a new Csp2–Csp3 bond between the indole ring and an unactivated methyl substituent. Considering that readily available toluene derivatives are easy to handle, inexpensive, quite stable, with low toxicity,11 and could be used as ideal in situ generated acylating reagents, we sought to develop a complementary method for the preparation of 2-acylindoles (Scheme 1).
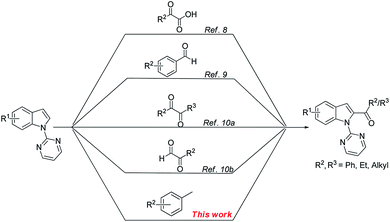 |
| Scheme 1 Previous reports towards C-2 acylation of N-pyrimidyl indoles. | |
We began to investigate the feasibility for oxidative cross-coupling between N-pyrimidyl indole and toluene by the identification of suitable reaction conditions. To this end, the model reaction between 1-(pyrimidin-2-yl)-1H-indole (1a) with toluene (2a) was systematically examined (Table 1). As the use of a transition-metal catalyst is generally needed both for activating the alkyl C–H bond and for the subsequent coupling to form the C–C bond, we screened various Pd-catalysts with tert-butyl hydroperoxide (TBHP) as oxidant in toluene (entries 1–4). The results revealed the superior catalytic activity of Pd catalysts having halide ions with PdBr2 providing a better yield (entry 4). Subsequently, we investigated the effect of various oxidants on this transformation and found that TBHP was the most suitable oxidant (45% yield), with other oxidants affording poorer reactivities (entries 5–7 vs. 4, also see ESI Table S1†). The above-obtained positive results encouraged us to explore co-solvents with toluene for achieving satisfying yields (entries 8–12). Using chlorobenzene as a co-solvent with toluene in a 3
:
1 ratio (v/v) led to a slightly higher yield (entry 12). Increasing the stoichiometry of TBHP to 5 equiv. resulted in higher yield (57%) of the desired product (entry 13). However a further increase led to a decrease in yield (entry 14). A significant influence of the additive on reaction was observed as the use of 0.5 equiv. of PivOH resulted in a relatively high yield of 73% (entry 15). However, either increasing the amount of PivOH or adding another acid additive (CF3COOH) was relatively ineffective (entries 16 and 17).
Table 1 Optimization of reaction conditionsa
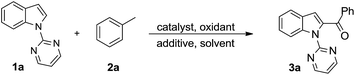
|
Entry |
Catalyst |
Oxidant |
Solvent |
Additive |
Yieldb (%) |
Reaction conditions: 1a (0.1 mmol), oxidant (3 equiv., 0.3 mmol), catalyst (10 mol%), solvent (1 mL), sealed tube, 120 °C, 24 h. NMR yields (isolated yields in parentheses). TBHP 5 equiv. TBHP 10 equiv. Additive 0.5 equiv. Additive 1 equiv. TBHP = tert-butyl hydroperoxide, DCP = dicumyl peroxide, DTBP = di-tert-butyl peroxide, TBPB = tert-butyl peroxybenzoate, PivOH = pivalic acid. nd = not detected. |
1 |
Pd(OAc)2 |
TBHP |
Toluene |
— |
7 |
2 |
PdCl2 |
TBHP |
Toluene |
— |
33 |
3 |
PdCl2(PPh3)2 |
TBHP |
Toluene |
— |
33 |
4 |
PdBr2 |
TBHP |
Toluene |
— |
45 |
5 |
PdBr2 |
DCP |
Toluene |
— |
27 |
6 |
PdBr2 |
DTBP |
Toluene |
— |
39 |
7 |
PdBr2 |
TBPB |
Toluene |
— |
Trace |
8 |
PdBr2 |
TBHP |
Toluene/EtOAc (1 : 1) |
— |
32 |
9 |
PdBr2 |
TBHP |
Toluene/DCE (1 : 1) |
— |
17 |
10 |
PdBr2 |
TBHP |
Toluene/PhCl (1 : 1) |
— |
47 |
11 |
PdBr2 |
TBHP |
Toluene/PhCl (2 : 1) |
— |
46 |
12 |
PdBr2 |
TBHP |
Toluene/PhCl (3 : 1) |
— |
50 |
13c |
PdBr2 |
TBHP |
Toluene/PhCl (3 : 1) |
— |
57 (50) |
14d |
PdBr2 |
TBHP |
Toluene/PhCl (3 : 1) |
— |
51 |
15c,e |
PdBr2 |
TBHP |
Toluene/PhCl (3 : 1) |
PivOH |
— (73) |
16c,e |
PdBr2 |
TBHP |
Toluene/PhCl (3 : 1) |
CF3COOH |
nd |
17c,f |
PdBr2 |
TBHP |
Toluene/PhCl (3 : 1) |
PivOH |
70 (63) |
A survey of other directing groups (Table 2) established that besides the N-2-pyrimidyl group (1a, entry 1) only the N-2-pyridyl group (1b, entry 2) is effective, albeit to a lower extent. The other reported directing groups for C-2 functionalization of indoles failed to yield any product under the optimized conditions (entries 3–6). Under the optimized reaction conditions (Table 1, entry 15), the substrate scope of this synthetic methodology was examined. As summarized in Table 3, a number of toluene derivatives were employed as acylation reagents to react with N-pyrimidyl indole (1a) to generate the desired acylation products (3a–o).
Table 2 Optimization of directing group
Table 3 Scope of toluene derivativesa,b
Reaction conditions: 1a (0.2 mmol), toluene derivatives (1.5 mL), TBHP (5 equiv.), PdBr2 (10 mol%), PhCl (0.5 mL), PivOH (0.5 equiv.), sealed tube, 120 °C, 24 h. Isolated yield. nd = not detected. |
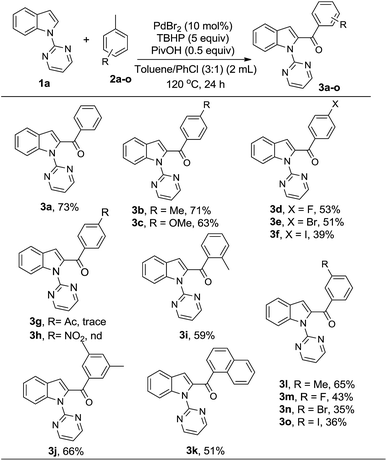 |
In general, reaction of 1a with toluene derivatives bearing electron-donating groups (such as CH3, OCH3) or weakly electron-withdrawing groups (such as Cl, Br, I) in para-position gave moderate to good yields (3b–f). However, the reaction was completely hampered in the presence of strongly electron-withdrawing groups such as acetyl (3g) and nitro (3h). In case of o-xylene (3i) and mesitylene (3j), the reaction took place on only one methyl group while the others remained untouched. This could be due to the fact that after oxidation of one of the methyl groups to the carbonyl group, its electron-withdrawing property renders the subsequent oxidations unfavorable.12a 1-Methylnaphthalene was also found to be a suitable coupling partner for the reaction (3k). Furthermore, the presence of a methyl group or a halo group in the meta-position of the aromatic ring was well tolerated (3l–3o).
Next, the substituent effect on the indole ring was investigated using toluene as coupling partner (Table 4). The reaction worked well with a variety of functional groups at the 3-, 4-, 5-, 6- and 7-position of the indole, such as F, Br, CH3, and CH3O (3p–3w) with moderate yields. Interestingly, it was observed that indoles bearing a substitution at the 5- or 6-position provide the corresponding products with slightly higher yields (3q–s, 3v) while moderate yields were observed for 4- or 7-substituted indoles (3t, 3w). However, a sterically hindering 3-substitution (3p) or the presence of a strongly electron-withdrawing group such as cyano on the indole ring (3u) gave an inferior yield. No reaction was observed with benzimidazole derivatives even after prolonged reaction time.
Table 4 Scope of indolesa,b
Reaction conditions: 1 (0.2 mmol), toluene (1.5 mL), TBHP (5 equiv.), PdBr2 (10 mol%), PhCl (0.5 mL), PivOH (0.5 equiv.), sealed tube, 120 °C, 24 h. Isolated yield. |
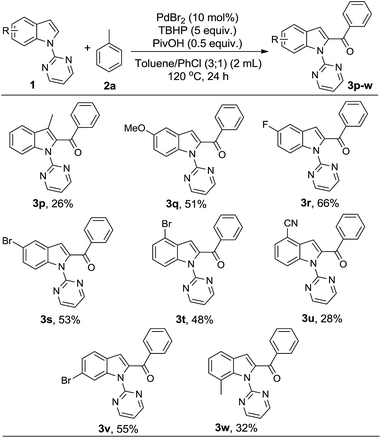 |
To enhance the synthetic utility of this process, facile removal of the N-pyrimidyl group, was carried out in a traceless fashion using sodium ethoxide in DMSO for 24 h at 100 °C, with an overall yield of 73% 3cc (Scheme 2).
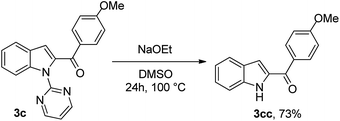 |
| Scheme 2 Traceless removal of the directing group. | |
Based on the previous research about C2-acylation of indoles with toluene as acylation reagent,11,12 we proposed a plausible reaction mechanism as shown in Scheme 3. First, Pd(II)Br2 reacted with the C2 position of the indole (1a) directed by the pyrimidin-2-yl to generate the five-membered cyclopalladated intermediate A. Subsequently, intermediate A would react with the benzoyl radical (formed in situ from the oxidation of toluene by TBHP), to produce the reactive Pd(IV)14 or dimeric Pd(III)15 species B. Finally, the cyclopalladated intermediate B underwent reductive elimination to give the desired product 3a. Meanwhile, the Pd(II) species was regenerated for the next catalytic cycle.
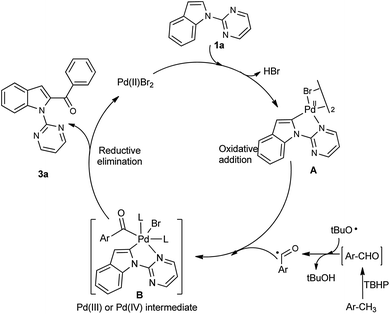 |
| Scheme 3 Plausible reaction mechanism. | |
Conclusions
We have developed an operationally simple and efficient Pd-catalyzed method for the synthesis of C2-acylated indoles. Low toxic, stable, and commercially available toluene derivatives were used as acyl source, with TBHP as oxidant and PivOH as additive. The reaction exhibited a satisfying functional group tolerance. The generality and operational simplicity of this method make it attractive for the alternative construction of 2-acylindoles.
Acknowledgements
YZ is grateful to the China Scholarship Council (CSC) for providing a visiting doctoral fellowship. UKS and NS are thankful to University of Leuven (KU Leuven) for a postdoctoral fellowship. FS is grateful to Flanders Innovation & Entrepreneurship (VLAIO) for providing a PhD scholarship. We acknowledge the support by COST (European Cooperation in Science and Technology) Action (CA15106) CH-Activation in Organic Synthesis. The research was financially support by The Ministry of Education and Science of the Russian Federation (the Agreement number 02.a03.0008).
Notes and references
-
(a) R. J. Sundberg, Indoles, Academic, New York, 1996 Search PubMed;
(b) M. Somei and F. Yamada, Nat. Prod. Rep., 2005, 22, 73–103 RSC;
(c) A. J. Kochanowska-Karamyan and M. T. Hamann, Chem. Rev., 2010, 110, 4489–4497 CrossRef CAS PubMed;
(d) M. Shiri, Chem. Rev., 2012, 112, 3508–3549 CrossRef CAS PubMed.
- Selected reviews, see:
(a) X. Chen, K. M. Engle, D.-H. Wang and J.-Q. Yu, Angew. Chem., Int. Ed., 2009, 48, 5094–5115 CrossRef CAS PubMed;
(b) O. Daugulis, H.-Q. Do and D. Shabashov, Acc. Chem. Res., 2009, 42, 1074–1086 CrossRef CAS PubMed;
(c) T. W. Lyons and M. S. Sanford, Chem. Rev., 2010, 110, 1147–1169 CrossRef CAS PubMed;
(d) L. Ackermann, Chem. Rev., 2011, 111, 1315–1345 CrossRef CAS PubMed;
(e) C. Liu, H. Zhang, W. Shi and A. Lei, Chem. Rev., 2011, 111, 1780–1824 CrossRef CAS PubMed;
(f) J. Wencel-Delord, T. Dr€oge, F. Liu and F. Glorius, Chem. Soc. Rev., 2011, 40, 4740–4761 RSC;
(g) C. S. Yeung and V. M. Dong, Chem. Rev., 2011, 111, 1215–1292 CrossRef CAS PubMed;
(h) D. A. Colby, A. S. Tsai, R. G. Bergman and J. A. Ellman, Acc. Chem. Res., 2012, 45, 814–825 CrossRef CAS PubMed;
(i) G. Song, F. Wang and X. Li, Chem. Soc. Rev., 2012, 41, 3651–3678 RSC;
(j) F. W. Patureau, J. Wencel-Delord and F. Glorius, Aldrichimica Acta, 2012, 45, 31–41 CAS;
(k) C. Pan, X. Jia and J. Cheng, Synthesis, 2012, 44, 677–685 CrossRef CAS;
(l) T.-S. Mei, L. Kou, S. Ma, K. M. Engle and J.-Q. Yu, Synthesis, 2012, 44, 1778–1791 CrossRef CAS PubMed.
-
(a) D. M. Ketcha and G. W. Gribble, J. Org. Chem., 1985, 50, 5451–5457 CrossRef CAS;
(b) T. Okauchi, M. Itonaga, T. Minami, T. Owa, K. Kitoh and H. Yoshino, Org. Lett., 2000, 2, 1485–1487 CrossRef CAS PubMed;
(c) O. Ottoni, A. V. Neder, A. K. Dias, R. P. Cruz and L. B. Aquino, Org. Lett., 2001, 3, 1005–1007 CAS;
(d) A. R. Katritzky, K. Suzuki, S. K. Singh and H. Y. He, J. Org. Chem., 2003, 68, 5720–5723 CrossRef CAS PubMed;
(e) W. Wu and W. Su, J. Am. Chem. Soc., 2011, 133, 11924–11927 CrossRef CAS PubMed;
(f) J. P. Brand, C. Chevalley, R. Scopelliti and J. Waser, Chem.–Eur. J., 2012, 18, 5655–5666 CrossRef CAS PubMed;
(g) J. Chen, B. Liu, D. Liu, S. Liu and J. Cheng, Adv. Synth. Catal., 2012, 354, 2438–2442 CrossRef CAS;
(h) J. L. Ruano, J. Aleman, L. Marzo, C. Alvarado, M. Tortosa, S. Diaz-Tendero and A. Fraile, Angew. Chem., Int. Ed., 2012, 51, 2712–2716 (Angew. Chem., 2012, 124, 2766–2770) CrossRef PubMed;
(i) E. Kianmehr, S. Kazemi and A. Foroumadi, Tetrahedron, 2014, 70, 349–354 CrossRef CAS;
(j) Y. Ma, J. You and F. Song, Chem.–Eur. J., 2013, 19, 1189–1193 CrossRef CAS PubMed;
(k) C. Wang, S. Wang, H. Li, J. Yan, H. Chi, X. Chen and Z. Zhang, Org. Biomol. Chem., 2014, 12, 1721–1724 RSC;
(l) Q. Xing, P. Li, H. Lv, R. Lang, C. Xia and F. Li, Chem. Commun., 2014, 50, 12181–12184 RSC.
-
(a) N. P. Grimster, C. Gauntlett, C. R. A. Godfrey and M. J. Gaunt, Angew. Chem., Int. Ed., 2005, 44, 3125–3129 CrossRef CAS PubMed;
(b) S. Lakhdar, M. Westermaier, F. Terrier, R. Goumont, T. Boubaker, A. R. Ofial and H. Mayr, J. Org. Chem., 2006, 71, 9088–9095 CrossRef CAS PubMed;
(c) J. P. Brand, J. Charpentier and J. Waser, Angew. Chem., Int. Ed., 2009, 48, 9346–9349 CrossRef CAS PubMed;
(d) Y. Gu and X. Wang, Tetrahedron Lett., 2009, 50, 763–766 CrossRef CAS;
(e) H. Yu and Z. Yu, Angew. Chem., Int. Ed., 2009, 48, 2929–2933 CrossRef CAS PubMed;
(f) S.-K. Xiang, B. Zhang, L.-H. Zhang, Y. Cui and N. Jiao, Chem. Commun., 2011, 47, 8097–8099 RSC;
(g) W.-L. Chen, Y.-R. Gao, S. Mao, Y.-L. Zhang, Y.-F. Wang and Y.-Q. Wang, Org. Lett., 2012, 14, 5920–5923 CrossRef CAS PubMed;
(h) Q. Yang, L. Wang, T. Guo and Z. Yu, J. Org. Chem., 2012, 77, 8355–8361 CrossRef CAS PubMed;
(i) Q. Huang, Q. Song, J. Cai, X. Zhang and S. Lin, Adv. Synth. Catal., 2013, 355, 1512–1516 CrossRef CAS;
(j) Y. P. Shang, X. M. Jie, J. Zhou, P. Hu, S. J. Huang and W. P. Su, Angew. Chem., Int. Ed., 2013, 52, 1299–1303 (Angew. Chem., 2013, 125, 1337–1341) CrossRef CAS PubMed.
-
(a) E. Capito, J. M. Brown and A. Ricci, Chem. Commun., 2005, 1854–1856 RSC;
(b) A. Garcia-Rubia, R. Gomez Arrayas and J. C. Carretero, Angew. Chem., Int. Ed., 2009, 48, 6511–6515 (Angew. Chem., 2009, 121, 6633–6637) CrossRef CAS PubMed;
(c) B. Li, J. Ma, W. Xie, H. Song, S. Xu and B. Wang, Chem.–Eur. J., 2013, 19, 11863–11868 CrossRef CAS PubMed;
(d) S. Sharma, S. Han, M. Kim, N. K. Mishra, J. Park, Y. Shin, J. Ha, J. H. Kwak, Y. H. Jung and I. S. Kim, Org. Biomol. Chem., 2014, 12, 1703–1706 RSC;
(e) S. Yu and X. Li, Org. Lett., 2014, 16, 1200–1203 CrossRef CAS PubMed.
-
(a) P. T. Anastas and J. C. Warner, Green Chemistry: Theory and Practice, Oxford University Press, New York, 1998 Search PubMed;
(b) J. C. Lewis, R. G. Bergman and J. A. Ellman, Acc. Chem. Res., 2008, 41, 1013–1025 CrossRef CAS PubMed;
(c) T. Newhouse, P. S. Baran and R. W. Hoffmann, Chem. Soc. Rev., 2009, 38, 3010–3021 RSC.
- B. Zhou, Y. Yang and Y. Li, Chem. Commun., 2012, 48, 5163–5165 RSC.
- C. Pan, H. Jin, X. Liu, Y. Cheng and C. Zhu, Chem. Commun., 2013, 49, 2933–2935 RSC.
- X.-B. Yan, Y.-W. Shen, D.-Q. Chen, P. Gao, Y.-X. Li, X.-R. Song, X.-Y. Liu and Y.-M. Liang, Tetrahedron, 2014, 70, 7490–7495 CrossRef CAS.
-
(a) C. Li, W. Zhu, S. Shu, X. Wu and H. Liu, Eur. J. Org. Chem., 2015, 3743–3750 CrossRef CAS;
(b) W. Wang, J. Liu, Q. Gui and Z. Tan, Synlett, 2015, 26, 771–778 CrossRef.
- Z. Xu, B. Xiang and P. Sun, RSC Adv., 2013, 3, 1679–1682 RSC.
-
(a) C.-W. Chan, Z. Zhou, A. S. C. Chan and W.-Y. Yu, Org. Lett., 2010, 12, 3926–3929 CrossRef CAS PubMed;
(b) Z. Yin and P. Sun, J. Org. Chem., 2012, 77, 11339–11344 CrossRef CAS PubMed;
(c) F. Xiong, C. Qian, D. Lin, W. Zeng and X. Lu, Org. Lett., 2013, 15, 5444–5447 CrossRef CAS PubMed;
(d) Y. Wu, P. Y. Choy, F. Mao and F. Y. Kwong, Chem. Commun., 2013, 49, 689–691 RSC;
(e) J. Weng, Z. Yu, X. Liu and G. Zhang, Tetrahedron Lett., 2013, 54, 1205–1207 CrossRef CAS;
(f) Y. Wu, L.-J. Feng, X. Lu, F. Y. Kwong and H.-B. Luo, Chem. Commun., 2014, 50, 15352–15354 RSC;
(g) S. K. Santra, A. Banerjee and B. K. Patel, Tetrahedron, 2014, 70, 2422–2430 CrossRef CAS;
(h) H. Song, D. Chen, C. Pi, X. Cui and Y. Wu, J. Org. Chem., 2014, 79, 2955–2962 CrossRef CAS PubMed;
(i) Y. Zheng, W.-B. Song, S.-W. Zhang and L.-J. Xuan, Tetrahedron, 2015, 71, 1574–1580 CrossRef CAS;
(j) Y.-F. Liang, X. Li, X. Wang, Y. Yan, P. Feng and N. Jiao, ACS Catal., 2015, 5, 1956–1963 CrossRef CAS;
(k) P. He, Q. Tian and C. Kuang, Synthesis, 2015, 47, 1309–1316 CrossRef CAS.
-
(a) Y. Zhao, L. Sun, T. Zeng, J. Wang, Y. Peng and G. Song, Org. Biomol. Chem., 2014, 12, 3493–3498 RSC;
(b) Y. Zhao, N. Sharma, U. K. Sharma, Z. Li, G. Song and E. V. Van der Eycken, Chem.–Eur. J., 2016, 32, 5878–5882 CrossRef PubMed;
(c) U. K. Sharma, H. Gamoets, F. Schrӧder, T. Noël and E. V. Van der Eycken, ACS Catal., 2017, 7, 3818–3823 CrossRef CAS.
-
(a) N. R. Deprez and M. S. Sanford, J. Am. Chem. Soc., 2009, 131, 11234 CrossRef CAS PubMed;
(b) J. M. Racowski, A. R. Dick and M. S. Sanford, J. Am. Chem. Soc., 2009, 131, 10974 CrossRef CAS PubMed.
-
(a) D. C. Powers and T. Ritter, Acc. Chem. Res., 2012, 45, 840 CrossRef CAS PubMed;
(b) D. C. Powers and T. Ritter, Nat. Chem., 2009, 1, 302 CrossRef CAS PubMed;
(c) D. C. Powers, M. A. L. Geibel, J. E. M. N. Klein and T. Ritter, J. Am. Chem. Soc., 2009, 131, 17050 CrossRef CAS PubMed.
Footnote |
† Electronic supplementary information (ESI) available. See DOI: 10.1039/c7ra06004a |
|
This journal is © The Royal Society of Chemistry 2017 |
Click here to see how this site uses Cookies. View our privacy policy here.