DOI:
10.1039/C7RA04960A
(Paper)
RSC Adv., 2017,
7, 29321-29329
Metal triflate promoted synthesis of naphthalenes†
Received
3rd May 2017
, Accepted 30th May 2017
First published on 6th June 2017
Abstract
A synthetic route to derive the skeleton of naphthalenes starting with isovanillin is described with modest total yields via the key transformation of metal triflate-mediated intramolecular benzannulation of o-formyl or o-benzoyl allylbenzenes in MeNO2 at rt.
Introduction
A naphthalene ring system1 is a key core skeleton for bioactive molecules,2 natural products3 and functionalized materials.4 A considerable number of attempts have been developed to determine the synthetic routes of the bicyclic skeleton. Two major key transformations for the formation of a functionalized naphthalene skeleton include: (1) acids (or Lewis acids) promote intramolecular Friedel–Crafts type annulations,5 and (2) bases promote intramolecular anionic annulations.6 Transition metal (e.g., Cr, Mn, Pd, W, Rh, Co, Ru, Ni, Co, Zn, Ti and Au) catalyzed benzannulations provide another approach for preparing the core structure.7 Therefore, a new synthetic route for diverse naphthalenes via intramolecular annulation of the readily available starting materials is still a continuing need in the synthetic field.
As part of our efforts in the synthetic applications of dialkoxy o-formyl allylbenzenes 18 and the development of metal triflate-promoted robust and efficient reactions,9 we have now streamlined the facile synthesis of substituted naphthalenes via metal triflate-mediated intramolecular benzannulation of o-carbonyl synthon allylbenzenes, such as o-formyl or o-benzoyl allylbenzenes, in MeNO2 at rt. To the best of our knowledge, few reports on the metal triflate-mediated intramolecular annulation of o-carbonyl synthon allylbenzenes have been documented.10 In 2011, Kuninobu and Takai described that metal triflates-catalyzed the dehydrative cycloaromatization of o-benzylbenzaldehyde 2 provided tricyclic anthracene in a 97% yield.10a Among the metal triflates-mediated syntheses, In(OTf)3 and Sc(OTf)3 demonstrated excellent transformation. In 2014, Luo demonstrated that Cu(OTf)2 provided a tetralin skeleton via the conjugate addition of 2′-allylchalcone 3 in a higher (90%) yield than the other scanned catalysts.10b The adopted synthetic routes are described in Scheme 1. Our aim was to find an optimal metal triflate as the catalyst for synthesizing naphthalenes 5 via the intramolecular benzannulation of o-formyl allylbenzene 4 (a hybrid combining the formyl motif of 2 and the allyl group of 3).
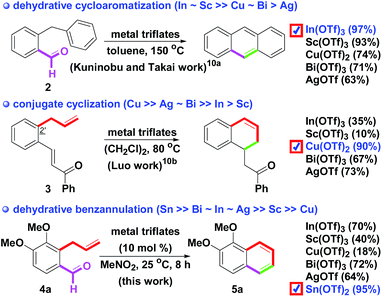 |
| Scheme 1 Metal triflates-catalyzed annulations. | |
Results and discussion
Initially, six metal triflates were chosen as the catalysts for examining the benzannulation of o-formyl allylbenzene 4a.11,12 In line with two preliminary studies, 10 mol% catalytic amounts of In(OTf)3, Sc(OTf)3, Cu(OTf)2, Bi(OTf)3, and AgOTf were first scanned for the formation of 1,2-dimethoxynaphthalene (5a) in MeNO2 at rt for 8 h, as shown in Table 1 and entries 1–5. However, 5a was isolated in a range of poor to moderate yields (18–72%). When Sn(OTf)2 or Fe(OTf)3 was applied to the reaction conditions, yields were increased to 95% or 88% (entries 6 and 7). Under the same conditions, treatment of 4a with Ga(OTf)3 provided 5a in a 44% yield along with a 38% yield of 6a (entry 8). In addition, 6a was generated via Ga(OTf)3-mediated by the addition of 4a with MeNO2. With these results in mind, a series of commercially available lanthanide triflates (Ln(OTf)3) were examined next, including: La(OTf)3, Ce(OTf)3, Pr(OTf)3, Nd(OTf)3, Sm(OTf)3, Eu(OTf)3, Gd(OTf)3, Tb(OTf)3, Dy(OTf)3, Ho(OTf)3, Er(OTf)3, Yb(OTf)3 and Tm(OTf)3. However, no isolation of the desired 5a was observed and only 4a was recovered under Ln(OTf)3-mediated reactions. With the results in hand, we found that Sn(OTf)2 was among the most reactive and used catalyst compared to other metal triflate derivatives. Along this line, we planned to take advantage of the intrinsically high catalytic ability of Sn(OTf)2 to construct the naphthalene system. After decreasing the catalytic amounts (10 → 5 mol%), a poor yield (72%) was observed (entry 9). Furthermore, we studied the factors of solvent and temperature. After changing the solvents (from MeNO2 to CH2Cl2 and toluene), different results were observed (entries 10 and 11). In entry 12, the complex mixture occurred (35%) when elevating the temperature (25 → 101).
Table 1 Metal triflates mediated reaction conditionsa
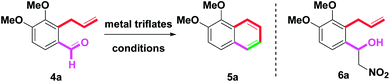
|
Entry |
Catalyst (mol%) |
Solvent |
Temp (°C) |
Time (h) |
5ab (%) |
Reactions were run on 4a (0.5 mmol), solvent (5 mL). Isolated yields. 4a was recovered (entry 5, 26%; entry 6, 54%; entry 14, 85%; entry 16, 80%). 6a (25%) was isolated. Unknown product (35%) was formed. Proton scavenger (2,6-di-tert-butylpyridine, 10 mol%) was added. No reaction. Water (10 mg) was added. |
1 |
Bi(OTf)3 (10) |
MeNO2 |
25 |
8 |
72 |
2 |
In(OTf)3 (10) |
MeNO2 |
25 |
8 |
70 |
3 |
AgOTf (10) |
MeNO2 |
25 |
8 |
64 |
4 |
Sc(OTf)3 (10) |
MeNO2 |
25 |
8 |
40c |
5 |
Cu(OTf)2 (10) |
MeNO2 |
25 |
8 |
18c |
6 |
Sn(OTf)2 (10) |
MeNO2 |
25 |
8 |
95 |
7 |
Fe(OTf)3 (10) |
MeNO2 |
25 |
8 |
88 |
8 |
Ga(OTf)3 (10) |
MeNO2 |
25 |
8 |
44 (38)d |
9 |
Sn(OTf)2 (5) |
MeNO2 |
25 |
8 |
72 |
10 |
Sn(OTf)2 (10) |
CH2Cl2 |
25 |
8 |
79 |
11 |
Sn(OTf)2 (10) |
Toluene |
25 |
8 |
69 |
12 |
Sn(OTf)2 (10) |
MeNO2 |
101 |
8 |
42e |
13 |
Sn(OTf)2 (10) |
MeNO2 |
25 |
20 |
83 |
14 |
SnCl2 (10) |
MeNO2 |
25 |
8 |
<5 |
15 |
TfOH (10) |
MeNO2 |
25 |
8 |
60 |
16 |
Sn(OTf)2 (10)f |
MeNO2 |
25 |
8 |
—c,g |
17 |
Sn(OTf)2 (10)h |
MeNO2 |
25 |
8 |
90 |
Next, Sn(OTf)2 exhibited a low yield (83%) under an elongated reaction time (8 → 20 h), as shown in entry 13. Another type of tin(II) salt (e.g. SnCl2) was examined. In entry 14, 5a was isolated in trace amounts only. By the involvement of 10 mol% TfOH (entry 15), 60% of 5a was generated. The control experiment revealed that the real catalyst was the trace amount of TfOH resulting from the hydrolysis of the metal triflates. In the other hand, proton scavenger (2,6-di-tert-butylpyridine, 10 mol%) was involved in the reaction system (entry 16). The resulting mixture failed to promote dehydrative benzannulation in the presence of 4a due to the proton scavenger could block in situ generated TfOH. To examine the stability and reactivity of Sn(OTf)2 in the presence of water, H2O (10 mg) was added to the reaction condition and a 90% yield of 5a was generated (entry 17). According to the results, a 10 mol% of Sn(OTf)2/MeNO2/rt condition would be an optimal combination for yielding 5a.
Aside from the present benzannulation of o-formyl allylbenzene 4a, Sn(OTf)2 has also been reported as a catalyst for aldol reactions,13a Mannish-type reactions,13b,c rearrangements,13d,e (3 + 2) annulations,13f–h and other routes.13i–k For branched, linear and cyclic alkyl substituents of o-formyl allylbenzenes 4b–d (R = Me, iPr, nBu, and c-C5H9), 5b–d provided good yields (90%, 91% or 88%) under the above-mentioned conditions (Scheme 2).
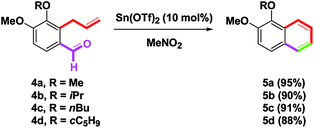 |
| Scheme 2 Synthesis of 5a–d. | |
On the basis of the results, a plausible reaction mechanism is shown in Scheme 3. Mechanistically, two general distinctive activation modes of the o-allylbenzaldehydes can be proposed for intramolecular dehydrative benzannulations. The first one is a purely metallic-based Lewis acid pathway. The sequence initiates the formation of intermediate A by complexation of a carbonyl motif of 3a with Sn(OTf)2. The in situ generated triflate anion deprotonates the Ha (blue) of A to give B as the result of a tandem intramolecular electrophilic annulation. Following the protonation of B with the TfOH, C is obtained. On the basis of the triflate anion-mediated deprotonation of C (for Hb, pink), 5a is provided via the removal of Sn(OH)OTf and TfOH. Subsequently, Sn(OTf)2 is regenerated for the next catalytic cycle by the complexation of Sn(OH)OTf and TfOH. The second possibility would involve a Brønsted acid catalysis. By Sn(OTf)2 assisted in situ formed TfOH, D is generated.17 Following the above similar route, 5a is also produced via (i) the triflate anion deprotonates the Ha of D, (ii) protonation of E with the TfOH, (iii) triflate anion-mediated deprotonation of F (for Hb), (iv) the removal of water and the regeneration of TfOH. Although metal triflate-mediated transformations have been described proceeding via proton transfer, we think that the nature of the catalytic species may be still under debate.18 For the overall benzannulation procedure, water is the only by-product. Therefore, it is important to use Sn(OTf)2 that are stable and show high reactivity even in the presence of water.9a,b,g,10a Metal cations (e.g. Bi3+, Al3+ or Sn2+) in water exhibit strong acidic properties because of the acidification through coordination of water molecules present in the “inner-sphere” of the cation.19 According to Duñach report,18d metal triflates such as Bi(OTf)3 are generally obtained and used as its hydrate form and therefore presents indubitably a strong induced Brønsted acidity.
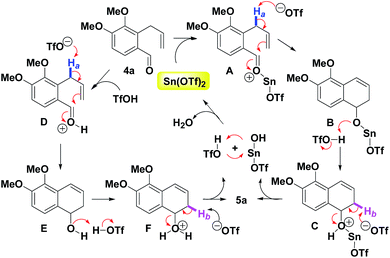 |
| Scheme 3 Proposed mechanism. | |
Furthermore, Sn(OTf)2-mediated conversion of o-aroyl allylbenzenes 4e–l into arylnaphthalenes 5e–l was examined, as shown in Scheme 4. The starting materials 4e–l (R = H, allyl; Ar = Ph, 4-FC6H4, 2-MeC6H4, 2-MeOC6H4, 3-MeOC6H4, 4-MeOC6H4, 3,4-CH2O2C6H3) were prepared by our previous reports.8p The substituents of R and Ar on 4e–l did not affect the yield outcome for the benzannulation procedure and no obvious yield changes were observed for the generation of 5e–l. The isolated yields were provided in the range of 88–95%. The structures of 5e and 5f were determined by single-crystal X-ray crystallography.14
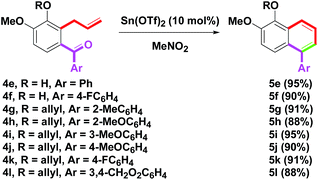 |
| Scheme 4 Synthesis of 5e–l. | |
As an extension of the benzannulation, the skeleton of aroylbenzene having two allyl arms (ortho and para positions) was studied (Scheme 5). Treatment of 4m–o (R = H, Me; Ar = 4-MeOC6H4, 3,4-CH2O2C6H3)8p with Sn(OTf)2 provided 5m–o in 95%, 90% and 91% yields, respectively. Notably, the p-allyl substituent was unaffected. With the results in hands, 10 mol% of Sn(OTf)2 was applied to the annulation of two substrates 4j (Ar = 4-MeOC6H4) or 4l (Ar = 3,4-CH2O2C6H3) having two allyl arms: one o-allyl group (blue) and one C-allyl group (red). Under a two-step procedure of Sn(OTf)2-mediated reaction and then O-methylation (K2CO3, MeI), we observed C-allyl isomers 7a or 7b were produced in only 21% and 15% yields, respectively via a one-pot tandem intramolecular dehydrative benzannulation and Claisen rearrangement (Scheme 6). By increasing the use of Sn(OTf)2 from catalytic amounts (10 mol%) to stoichiometric amounts (1.0 equivalent),15 the yields of 7a or 7b were enhanced to 85% and 80% yields, respectively via the two-step route. The non-catalytic amounts (1.0 equivalent) of Sn(OTf)2 which mediated the tandem intramolecular procedure included: (1) benzannulation of o-allyl and aroyl groups and (2) double Claisen rearrangement of the o-allyl group. In particular, the present double Claisen rearrangement occurred at room temperature. In comparison with the literature reports (150–200 °C),16 a few examples were described.
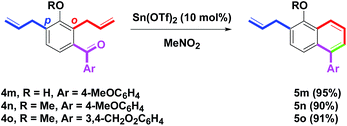 |
| Scheme 5 Synthesis of 5m–o. | |
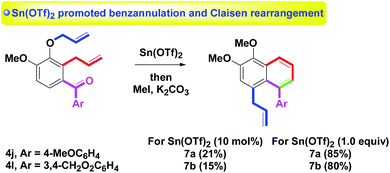 |
| Scheme 6 Synthesis of 7a–b. | |
To change the position of the aryl group on the naphthalene skeleton, eight 4p–v (Ar = Ph, 4-FC6H4, 4-MeC6H4, 4-MeOC6H4, 4-CF3C6H4, 2-MeOC6H4, 4-PhC6H4) were investigated next. By this synthetic protocol, 8a–g were synthesized in 74–81% yields, as shown in Scheme 7. By the removal of the m-oxygenated group, 4w–x having the o-allyl side arms (Y = H, MeO) were then examined. When benzannulation of 4w–x was treated with Sn(OTf)2, the yields of 9a (61%) and 9b (40%) provide good results (Scheme 8). The structures of 8d, 8g, 9a and 9b were determined by single-crystal X-ray crystallography.14 By a one-pot two-step route (Suzuki–Miyaura coupling and our method),8p a simple naphthalene 10a could be obtained from o-formyl phenyl boronic acids in a 70% yield via the formation of o-allylbenzaldehyde 4y (Scheme 9). Although the isolated yield of 10a was low, it still provided a novel and efficient transformation from o-formyl phenylboronic acid to naphthalene.
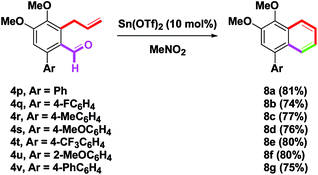 |
| Scheme 7 Synthesis of 8a–g. | |
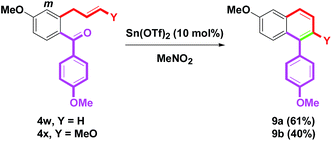 |
| Scheme 8 Synthesis of 9a–b. | |
 |
| Scheme 9 Synthesis of 10a. | |
Conclusion
In summary, we have successfully presented a synthetic route for the synthesis of substituted naphthalenes in good yields via Sn(OTf)2-mediated benzannulation of o-formyl or o-aroyl allylbenzenes. The use of various metal triflates was investigated for the one-pot facile approach and efficient transformation. Further investigations regarding the synthetic application of metal triflates will be conducted and published in due course.
Experimental
General
All reagents and solvents were obtained from commercial sources and used without further purification. Reactions were routinely carried out under an atmosphere of dry air with magnetic stirring. Products in organic solvents were dried with anhydrous magnesium sulfate before concentration in vacuo. Melting points were determined with a SMP3 melting apparatus. 1H and 13C NMR spectra were recorded on a Varian INOVA-400 spectrometer operating at 400 and at 100 MHz, respectively. Chemical shifts (δ) are reported in parts per million (ppm) and the coupling constants (J) are given in Hertz. High resolution mass spectra (HRMS) were measured with a mass spectrometer Finnigan/Thermo Quest MAT 95XL. X-ray crystal structures were obtained with an Enraf-Nonius FR-590 diffractometer (CAD4, Kappa CCD).
A representative procedure of skeleton 5 and compound 6a is as follows
Sn(OTf)2 (42 mg, 0.1 mmol) was added to a solution of 4a–o (1.0 mmol) in MeNO2 (5 mL) at 25 °C. The reaction mixture was stirred for 8 h at 25 °C and the solvent was concentrated. The residue was diluted with water (10 mL) and the mixture was extracted with CH2Cl2 (3 × 20 mL). The combined organic layers were washed with brine, dried, filtered and evaporated to afford crude product. Purification on silica gel (hexanes/EtOAc = 100/1–10/1) afforded 5 and 6a.
1,2-Dimethoxynaphthalene (5a). Yield = 95% (179 mg); colorless oil; HRMS (ESI, M+ + 1) calcd for C12H13O2 189.0916, found 189.0919; 1H NMR (400 MHz, CDCl3): δ 8.15 (d, J = 8.4 Hz, 1H), 7.80 (d, J = 8.4 Hz, 1H), 7.62 (d, J = 8.8 Hz, 1H), 7.52–7.48 (m, 1H), 7.40–7.36 (m, 1H), 7.31 (d, J = 8.8 Hz, 1H), 4.03 (s, 3H), 4.01 (s, 3H); 13C NMR (100 MHz, CDCl3): δ 148.28, 142.84, 129.63, 128.98, 127.61, 126.05, 124.17, 124.01, 121.23, 115.15, 61.06, 56.83.
1-(2-Allyl-3,4-dimethoxyphenyl)-2-nitroethanol (6a). For Ga(OTf)3 promoted reaction, 6a was generated in 38% (101 mg); colorless solid; mp = 78–80 °C (recrystallized from hexanes and EtOAc); HRMS (ESI, M+ + 1) calcd for C13H18NO5 268.1185, found 268.1188; 1H NMR (400 MHz, CDCl3): δ 7.24 (d, J = 8.4 Hz, 1H), 6.87 (d, J = 8.4 Hz, 1H), 6.02–5.92 (m, 1H), 5.60 (dd, J = 2.8, 10.0 Hz, 1H), 5.60 (dq, J = 1.6, 10.0 Hz, 1H), 4.93 (dq, J = 1.6, 17.2 Hz, 1H), 4.49 (q, J = 13.2 Hz, 1H), 4.48 (q, J = 13.2 Hz, 1H), 3.87 (s, 3H), 3.80 (s, 3H), 3.53 (t, J = 5.6 Hz, 2H), 2.41 (br s, 1H); 13C NMR (100 MHz, CDCl3): δ 152.87, 147.13, 136.98, 130.59, 129.66, 121.95, 115.74, 110.93, 80.88, 67.28, 60.89, 55.66, 29.86.
1-Isopropoxy-2-methoxynaphthalene (5b). Yield = 90% (194 mg); colorless oil; HRMS (ESI, M+ + 1) calcd for C14H17O2 217.1229, found 217.1230; 1H NMR (400 MHz, CDCl3): δ 8.19 (d, J = 8.4 Hz, 1H), 7.78 (d, J = 8.4 Hz, 1H), 7.59 (d, J = 9.2 Hz, 1H), 7.49–7.45 (m, 1H), 7.38–7.34 (m, 1H), 7.30 (d, J = 8.8 Hz, 1H), 4.72–4.65 (m, 1H), 3.98 (s, 3H), 1.40 (d, J = 6.0 Hz, 6H); 13C NMR (100 MHz, CDCl3): δ 148.53, 140.93, 130.40, 129.72, 127.43, 125.67, 123.88, 123.67, 122.17, 115.36, 75.30, 56.84, 22.71 (2x).
1-Butoxy-2-methoxynaphthalene (5c). Yield = 91% (209 mg); colorless oil; HRMS (ESI, M+ + 1) calcd for C15H19O2 231.1385, found 231.1388; 1H NMR (400 MHz, CDCl3): δ 8.17 (d, J = 8.4 Hz, 1H), 7.79 (d, J = 8.4 Hz, 1H), 7.60 (d, J = 8.8 Hz, 1H), 7.50–7.46 (m, 1H), 7.39–7.35 (m, 1H), 7.30 (d, J = 8.8 Hz, 1H), 4.16 (t, J = 6.4 Hz, 2H), 3.99 (s, 3H), 1.94–1.87 (m, 2H), 1.67–1.58 (m, 2H), 1.04 (t, J = 7.2 Hz, 3H); 13C NMR (100 MHz, CDCl3): δ 148.36, 142.34, 129.77, 129.45, 127.55, 125.88, 123.98, 123.86, 121.52, 115.53, 73.43, 57.00, 32.50, 19.34, 13.94.
1-Cyclopentyloxy-2-methoxynaphthalene (5d). Yield = 88% (213 mg); colorless oil; HRMS (ESI, M+ + 1) calcd for C16H19O2 243.1385, found 243.1382; 1H NMR (400 MHz, CDCl3): δ 8.13 (d, J = 8.0 Hz, 1H), 7.76 (d, J = 8.0 Hz, 1H), 7.57 (d, J = 8.8 Hz, 1H), 7.47–7.43 (m, 1H), 7.37–7.33 (m, 1H), 7.29 (d, J = 8.8 Hz, 1H), 5.09–5.05 (m, 1H), 3.97 (s, 3H), 2.04–1.92 (m, 4H), 1.80–1.72 (m, 2H), 1.70–1.61 (m, 2H); 13C NMR (100 MHz, CDCl3): δ 148.33, 141.15, 130.34, 129.81, 127.48, 125.70, 123.95, 123.55, 121.98, 115.71, 84.79, 57.00, 32.91 (2x), 23.78 (2x).
2-Methoxy-5-phenylnaphthalen-1-ol (5e). Yield = 95% (238 mg); colorless solid; HRMS (ESI, M+ + 1) calcd for C17H15O2 251.1072, found 251.1078; 1H NMR (400 MHz, CDCl3): δ 8.22 (d, J = 8.4 Hz, 1H), 7.56–7.41 (m, 7H), 7.32 (d, J = 6.8 Hz, 1H), 7.19 (d, J = 9.2 Hz, 1H), 6.13 (br s, 1H), 3.98 (s, 3H); 13C NMR (100 MHz, CDCl3): δ 141.02, 139.83, 139.69, 129.98 (2x), 128.90, 128.12 (2x), 127.70, 127.11, 125.33, 124.92, 124.34, 120.72, 117.93, 112.93, 57.00. Single-crystal X-ray diagram: crystal of compound 5e was grown by slow diffusion of EtOAc into a solution of compound 5e in CH2Cl2 to yield colorless prisms. The compound crystallizes in the orthorhombic crystal system, space group Fdd2, a = 13.3885(3) Å, b = 66.3135(18) Å, c = 5.5816(2) Å, V = 4955.6(2) Å3, Z = 16, dcalcd = 1.342 g cm−3, F(000) = 2112, 2θ range 1.23–26.39°, R indices (all data) R1 = 0.0428, wR2 = 0.1154.
5-(4-Fluorophenyl)-2-methoxynaphthalen-1-ol (5f). Yield = 90% (241 mg); colorless solid; HRMS (ESI, M+ + 1) calcd for C17H14FO2 269.0978, found 269.0983; 1H NMR (400 MHz, CDCl3): δ 8.26 (d, J = 8.4 Hz, 1H), 7.53–7.39 (m, 4H), 7.7.29 (dd, J = 1.2, 6.8 Hz, 1H), 7.22–7.17 (m, 3H), 6.18 (br s, 1H), 3.99 (s, 3H); 13C NMR (100 MHz, CDCl3): δ 162.16 (d, J = 244.1 Hz), 141.08, 139.74, 138.71, 136.87 (d, J = 3.0 Hz), 131.46 (d, J = 17.5 Hz, 2x), 127.71, 125.41, 124.87, 124.35, 120.92, 117.65, 115.02 (d, J = 21.2 Hz, 2x), 113.01, 56.94. Single-crystal X-ray diagram: crystal of compound 5f was grown by slow diffusion of EtOAc into a solution of compound 5f in CH2Cl2 to yield colorless prisms. The compound crystallizes in the triclinic crystal system, space group P
, a = 6.7021(11) Å, b = 7.9371(12) Å, c = 12.170(2) Å, V = 631.92(18) Å3, Z = 2, dcalcd = 1.410 g cm−3, F(000) = 280, 2θ range 1.70–26.38°, R indices (all data) R1 = 0.0431, wR2 = 0.1097.
1-Allyloxy-2-methoxy-5-o-tolylnaphthalene (5g). Yield = 91% (277 mg); colorless gum; HRMS (ESI, M+ + 1) calcd for C21H21O2 305.1542, found 305.1549; 1H NMR (400 MHz, CDCl3): δ 8.19 (dt, J = 0.4, 8.4 Hz, 1H), 7.51 (dd, J = 6.8, 8.4 Hz, 1H), 7.38–7.16 (m, 7H), 6.30–6.20 (m, 1H), 5.49 (dq, J = 1.6, 17.2 Hz, 1H), 5.30 (dq, J = 1.6, 10.4 Hz, 1H), 4.71 (dt, J = 1.6, 6.0 Hz, 2H), 3.97 (s, 3H), 2.03 (s, 3H); 13C NMR (100 MHz, CDCl3): δ 148.20, 141.77, 140.33, 139.58, 136.76, 134.49, 130.30, 129.81, 129.61, 128.23, 127.50, 125.68, 125.48, 124.89, 122.60, 121.00, 117.39, 114.90, 74.33, 56.80, 20.01.
1-Allyloxy-2-methoxy-5-(2-methoxyphenyl)naphthalene (5h). Yield = 88% (282 mg); colorless gum; HRMS (ESI, M+ + 1) calcd for C21H21O3 321.1491, found 321.1496; 1H NMR (400 MHz, CDCl3): δ 8.19 (dt, J = 1.2, 8.4 Hz, 1H), 7.52 (dd, J = 7.2, 8.8 Hz, 1H), 7.43 (ddd, J = 1.6, 7.2, 9.2 Hz, 1H), 7.34 (dd, J = 0.8, 9.2 Hz, 1H), 7.30–7.27 (m, 2H), 7.18 (d, J = 9.2 Hz, 1H), 7.09 (dd, J = 0.8, 7.2 Hz, 1H), 7.05 (d, J = 7.2 Hz, 1H), 6.30–6.20 (m, 1H), 5.49 (dq, J = 1.6, 17.2 Hz, 1H), 5.29 (dq, J = 1.6, 10.4 Hz, 1H), 4.70 (dt, J = 1.6, 6.0 Hz, 2H), 3.97 (s, 3H), 3.71 (s, 3H); 13C NMR (100 MHz, CDCl3): δ 157.19, 148.08, 141.68, 136.74, 134.56, 131.91, 129.64, 129.57, 128.92, 128.36, 125.67, 125.60, 122.94, 121.22, 120.50, 117.34, 114.76, 111.01, 74.33, 56.82, 55.59.
1-Allyloxy-2-methoxy-5-(3-methoxyphenyl)naphthalene (5i). Yield = 95% (304 mg); colorless gum; HRMS (ESI, M+ + 1) calcd for C21H21O3 321.1491, found 321.1499; 1H NMR (400 MHz, CDCl3): δ 8.18 (d, J = 8.4 Hz, 1H), 7.68 (d, J = 9.2 Hz, 1H), 7.51 (dd, J = 7.2, 8.4 Hz, 1H), 7.40 (t, J = 8.0 Hz, 1H), 7.31 (dd, J = 0.8, 6.8 Hz, 1H), 7.23 (d, J = 9.2 Hz, 1H), 7.09–7.06 (m, 1H), 7.04 (t, J = 2.4 Hz, 1H), 6.98 (ddd, J = 0.8, 2.8, 8.4 Hz, 1H), 6.29–6.19 (m, 1H), 5.48 (dq, J = 1.6, 17.2 Hz, 1H), 5.29 (dq, J = 1.6, 10.0 Hz, 1H), 4.70 (dt, J = 1.6, 6.0 Hz, 2H), 3.98 (s, 3H), 3.86 (s, 3H); 13C NMR (100 MHz, CDCl3): δ 159.41, 148.23, 142.27, 141.63, 139.95, 134.41, 129.87, 129.15, 127.76, 125.65, 125.03, 122.65, 122.51, 121.21, 117.52, 115.52, 114.87, 112.86, 74.33, 56.78, 55.28.
1-Allyloxy-2-methoxy-5-(4-methoxyphenyl)naphthalene (5j). Yield = 90% (288 mg); colorless gum; HRMS (ESI, M+ + 1) calcd for C21H21O3 321.1491, found 321.1493; 1H NMR (400 MHz, CDCl3): δ 8.15 (dt, J = 0.8, 8.4 Hz, 1H), 7.66 (dd, J = 0.8, 9.2 Hz, 1H), 7.48 (dd, J = 7.2, 8.8 Hz, 1H), 7.40 (d, J = 8.8 Hz, 2H), 7.27 (dd, J = 0.8, 7.2 Hz, 1H), 7.21 (d, J = 9.2 Hz, 1H), 7.01 (d, J = 8.8 Hz, 2H), 6.27–6.17 (m, 1H), 5.46 (dq, J = 1.6, 17.2 Hz, 1H), 5.27 (dq, J = 1.6, 10.4 Hz, 1H), 4.68 (dt, J = 1.6, 6.0 Hz, 2H), 3.96 (s, 3H), 3.88 (s, 3H); 13C NMR (100 MHz, CDCl3): δ 158.90, 148.19, 141.66, 139.76, 134.43, 133.24, 131.03 (2x), 129.93, 128.05, 125.73, 125.18, 122.67, 120.84, 117.49, 114.76, 113.64 (2x), 74.32, 56.79, 55.32.
1-Allyloxy-5-(4-fluorophenyl)-2-methoxynaphthalene (5k). Yield = 91% (280 mg); colorless gum; HRMS (ESI, M+ + 1) calcd for C20H18FO2 309.1291, found 309.1293; 1H NMR (400 MHz, CDCl3): δ 8.22 (dt, J = 0.8, 8.4 Hz, 1H), 7.60 (dd, J = 0.8, 9.2 Hz, 1H), 7.51 (dd, J = 6.8, 8.4 Hz, 1H), 7.47–7.42 (m, 2H), 7.27 (dd, J = 1.2, 7.2 Hz, 1H), 7.24 (d, J = 9.2 Hz, 1H), 7.21–7.15 (m, 2H), 6.30–6.20 (m, 1H), 5.49 (dq, J = 1.6, 17.2 Hz, 1H), 5.30 (dq, J = 1.6, 10.4 Hz, 1H), 4.72 (dt, J = 1.6, 6.0 Hz, 2H), 3.99 (s, 3H); 13C NMR (100 MHz, CDCl3): δ 162.21 (d, J = 244.1 Hz), 148.26, 141.69, 138.97, 136.76 (d, J = 3.0 Hz), 134.36, 131.46 (d, J = 7.5 Hz, 2x), 129.90, 127.82, 125.64, 125.25, 122.31, 121.32, 117.52, 115.09 (d, J = 21.2 Hz, 2x), 114.99, 74.31, 56.75.
5-(5-Allyloxy-6-methoxynaphthalen-1-yl)benzo[1,3]dioxole (5l). Yield = 88% (294 mg); colorless gum; HRMS (ESI, M+ + 1) calcd for C21H19O4 335.1283, found 335.1288; 1H NMR (400 MHz, CDCl3): δ 8.16 (dt, J = 1.2, 8.4 Hz, 1H), 7.68 (dd, J = 1.2, 9.2 Hz, 1H), 7.48 (dd, J = 6.8, 8.4 Hz, 1H), 7.26 (dd, J = 1.2, 7.2 Hz, 1H), 7.23 (d, J = 9.2 Hz, 1H), 6.96 (br s, 1H), 6.93 (br s, 2H), 6.27–6.17 (m, 1H), 6.04 (s, 2H), 5.47 (dq, J = 1.6, 16.8 Hz, 1H), 5.28 (dq, J = 1.6, 10.4 Hz, 1H), 4.68 (dt, J = 1.6, 6.0 Hz, 2H), 3.98 (s, 3H); 13C NMR (100 MHz, CDCl3): δ 148.23, 147.42, 141.66, 139.66, 134.74, 134.41, 129.92, 127.96, 126.01, 125.67, 125.16, 123.35, 122.58, 121.06, 117.52, 114.85, 110.57, 108.17, 101.09, 74.33, 56.81.
2-Allyl-5-(4-methoxyphenyl)naphthalen-1-ol (5m). Yield = 95% (276 mg); colorless gum; HRMS (ESI, M+ + 1) calcd for C20H19O2 291.1385, found 291.1386; 1H NMR (400 MHz, CDCl3): δ 8.20 (dt, J = 1.2, 8.4 Hz, 1H), 7.51 (dd, J = 6.8, 8.4 Hz, 1H), 7.46 (dd, J = 0.8, 8.4 Hz, 1H), 7.42 (d, J = 8.8 Hz, 2H), 7.38 (dd, J = 1.2, 6.8 Hz, 1H), 7.17 (d, J = 8.4 Hz, 1H), 7.03 (d, J = 8.8 Hz, 2H), 6.14–6.04 (m, 1H), 5.60 (br s, 1H), 5.30–5.23 (m, 2H), 3.90 (s, 3H), 3.58 (dt, J = 1.6, 6.4 Hz, 2H); 13C NMR (100 MHz, CDCl3): δ 158.88, 149.77, 139.59, 136.05, 133.35, 132.19, 131.07 (2x), 128.27, 126.89, 125.24, 124.86, 120.60, 118.65, 117.57, 117.05, 113.63 (2x), 55.33, 35.73.
2-Allyl-1-methoxy-5-(4-methoxyphenyl)naphthalene (5n). Yield = 90% (274 mg); colorless gum; HRMS (ESI, M+ + 1) calcd for C21H21O2 305.1542, found 305.1548; 1H NMR (400 MHz, CDCl3): δ 8.16 (dt, J = 1.2, 8.4 Hz, 1H), 7.69 (dd, J = 0.8, 8.4 Hz, 1H), 7.56 (dd, J = 7.2, 8.4 Hz, 1H), 7.43 (d, J = 8.8 Hz, 2H), 7.40 (dd, J = 1.6, 6.8 Hz, 1H), 7.29 (d, J = 8.8 Hz, 1H), 7.05 (d, J = 8.8 Hz, 2H), 6.12–6.02 (m, 1H), 5.16–5.11 (m, 2H), 3.99 (s, 3H), 3.91 (s, 3H), 3.64 (dt, J = 1.6, 6.4 Hz, 2H); 13C NMR (100 MHz, CDCl3): δ 158.89, 153.47, 140.10, 137.11, 133.18, 132.25, 131.03 (2x), 128.55, 128.18, 127.76, 126.64, 125.45, 122.25, 121.25, 115.85, 113.64 (2x), 62.14, 55.29, 33.82.
5-(6-Allyl-5-methoxynaphthalen-1-yl)benzo[1,3]dioxole (5o). Yield = 91% (289 mg); colorless gum; HRMS (ESI, M+ + 1) calcd for C21H19O3 319.1334, found 319.1336; 1H NMR (400 MHz, CDCl3): δ 8.13 (dt, J = 1.2, 8.4 Hz, 1H), 7.67 (d, J = 8.8 Hz, 1H), 7.52 (dd, J = 7.2, 8.4 Hz, 1H), 7.37 (dd, J = 1.2, 6.8 Hz, 1H), 7.27 (d, J = 8.8 Hz, 1H), 6.97 (t, J = 1.6 Hz, 1H), 6.93 (br s, 2H), 6.04 (s, 2H), 6.10–6.00 (m, 1H), 5.14–5.08 (m, 2H), 3.96 (s, 3H), 3.61 (dt, J = 1.6, 6.4 Hz, 2H); 13C NMR (100 MHz, CDCl3): δ 153.50, 147.44, 146.86, 140.04, 137.10, 134.70, 132.21, 128.56, 128.30, 127.86, 126.64, 125.41, 123.37, 122.16, 121.48, 115.90, 110.58, 108.17, 101.10, 62.18, 33.82.
A representative procedure of skeleton 7 is as follows
Sn(OTf)2 (420 mg, 1.0 mmol) was added to a solution of 4j or 4l (1.0 mmol) in MeNO2 (5 mL) at 25 °C. The reaction mixture was stirred for 8 h at 25 °C and the solvent was concentrated. The residue was diluted with water (10 mL) and the mixture was extracted with CH2Cl2 (3 × 20 mL). The combined organic layers were washed with brine, dried, filtered and evaporated to afford crude product. Without further purification, K2CO3 (276 mg, 2.0 mmol) was added to a solution of the resulting products in acetone (10 mL) at 25 °C for 10 min. MeI (426 mg, 3.0 mmol) was added to the reaction mixture. The reaction mixture was stirred for 8 h at reflux, cooled to 25 °C and the solvent was concentrated. The residue was diluted with water (10 mL) and the mixture was extracted with CH2Cl2 (3 × 20 mL). The combined organic layers were washed with brine, dried, filtered and evaporated to afford crude product. Purification on silica gel (hexanes/EtOAc = 100/1–10/1) afforded 7.
4-Allyl-1,2-dimethoxy-5-(4-methoxyphenyl)naphthalene (7a). Yield = 85% (284 mg); colorless gum; HRMS (ESI, M+ + 1) calcd for C22H23O3 335.1647, found 335.1652; 1H NMR (400 MHz, CDCl3): δ 8.21 (dt, J = 1.2, 8.4 Hz, 1H), 7.44 (dd, J = 6.8, 8.4 Hz, 1H), 7.24 (d, J = 8.8 Hz, 2H), 7.17 (dd, J = 1.2, 6.8 Hz, 1H), 7.13 (s, 1H), 6.93 (d, J = 8.8 Hz, 2H), 5.70–5.60 (m, 1H), 4.92 (dq, J = 1.6, 10.0 Hz, 1H), 4.73 (dq, J = 1.6, 17.2 Hz, 1H), 4.01 (s, 3H), 3.98 (s, 3H), 3.88 (s, 3H), 3.13 (dt, J = 1.6, 6.4 Hz, 2H); 13C NMR (100 MHz, CDCl3): δ 158.64, 147.29, 141.43, 139.61, 138.20, 137.16, 134.02, 131.03, 130.21 (2x), 128.53, 124.70, 121.42, 117.95, 115.56, 113.64, 113.17 (2x), 61.08, 56.69, 55.28, 39.75.
5-(8-Allyl-5,6-dimethoxynaphthalen-1-yl)benzo[1,3]dioxole (7b). Yield = 80% (278 mg); colorless gum; HRMS (ESI, M+ + 1) calcd for C22H21O4 349.1440, found 349.1450; 1H NMR (400 MHz, CDCl3): δ 8.21 (dd, J = 1.2, 8.4 Hz, 1H), 7.43 (dd, J = 7.2, 8.4 Hz, 1H), 7.17 (dd, J = 1.6, 7.2 Hz, 1H), 7.14 (s, 1H), 6.84 (d, J = 8.0 Hz, 1H), 6.81 (d, J = 1.6 Hz, 1H), 6.77 (dd, J = 1.6, 8.0 Hz, 1H), 6.05 (d, J = 1.2 Hz, 1H), 6.01 (d, J = 1.2 Hz, 1H), 5.74–5.64 (m, 1H), 4.96 (dq, J = 1.6, 10.4 Hz, 1H), 4.78 (dq, J = 1.6, 17.2 Hz, 1H), 4.00 (s, 3H), 3.98 (s, 3H), 3.19 (d, J = 6.0 Hz, 2H); 13C NMR (100 MHz, CDCl3): δ 147.35, 147.02, 146.57, 141.44, 139.42, 138.54, 138.18, 133.88, 130.75, 128.40, 126.69, 124.66, 122.54, 121.63, 118.03, 115.65, 110.02, 107.77, 101.03, 61.09, 56.69, 39.61.
A representative procedure of skeleton 8 is as follows
Sn(OTf)2 (42 mg, 0.1 mmol) was added to a solution of 4p–v (1.0 mmol) in MeNO2 (5 mL) at 25 °C. The reaction mixture was stirred for 8 h at 25 °C and the solvent was concentrated. The residue was diluted with water (10 mL) and the mixture was extracted with CH2Cl2 (3 × 20 mL). The combined organic layers were washed with brine, dried, filtered and evaporated to afford crude product. Purification on silica gel (hexanes/EtOAc = 100/1–10/1) afforded 8.
1,2-Dimethoxy-4-phenylnaphthalene (8a). Yield = 81% (214 mg); colorless gum; HRMS (ESI, M+ + 1) calcd for C18H17O2 265.1229, found 265.1230; 1H NMR (400 MHz, CDCl3): δ 8.22 (d, J = 8.8 Hz, 1H), 7.81 (d, J = 8.8 Hz, 1H), 7.53–7.43 (m, 6H), 7.34–7.30 (m, 1H), 7.27 (s, 1H), 4.06 (s, 3H), 4.02 (s, 3H); 13C NMR (100 MHz, CDCl3): δ 147.55, 142.32, 140.49, 136.89, 130.07 (2x), 129.32, 128.26 (2x), 127.74, 127.28, 126.05 (2x), 124.14, 121.54, 116.40, 61.14, 56.91.
4-(4-Fluorophenyl)-1,2-dimethoxynaphthalene (8b). Yield = 74% (209 mg); colorless gum; HRMS (ESI, M+ + 1) calcd for C18H16FO2 283.1134, found 283.1139; 1H NMR (400 MHz, CDCl3): δ 8.20 (d, J = 8.8 Hz, 1H), 7.74 (d, J = 8.8 Hz, 1H), 7.52–7.48 (m, 1H), 7.47–7.42 (m, 2H), 7.34–7.30 (m, 1H), 7.21 (s, 1H), 7.20–7.16 (m, 2H), 4.05 (s, 3H), 4.01 (s, 3H); 13C NMR (100 MHz, CDCl3): δ 162.28 (d, J = 244.9 Hz), 147.54, 142.51, 136.39 (d, J = 3.1 Hz), 135.75, 131.59 (d, J = 7.6 Hz, 2x), 129.35, 127.83, 126.12, 125.81, 124.31, 121.63, 116.57, 115.21 (d, J = 21.2 Hz, 2x), 61.16, 56.99.
1,2-Dimethoxy-4-p-tolylnaphthalene (8c). Yield = 77% (214 mg); colorless gum; HRMS (ESI, M+ + 1) calcd for C19H19O2 279.1385, found 279.1388; 1H NMR (400 MHz, CDCl3): δ 8.23–8.20 (m, 1H), 7.85–7.82 (m, 1H), 7.50 (ddd, J = 1.2, 6.8, 8.4 Hz, 1H), 7.40 (d, J = 8.0 Hz, 2H), 7.34–7.29 (m, 3H), 7.25 (s, 1H), 4.06 (s, 3H), 4.02 (s, 3H), 2.48 (s, 3H); 13C NMR (100 MHz, CDCl3): δ 147.57, 142.17, 137.55, 137.01, 136.91, 129.94 (2x), 129.32, 128.98 (2x), 127.83, 126.13, 125.99, 124.05, 121.51, 116.33, 61.13, 56.88, 21.19.
1,2-Dimethoxy-4-(4-methoxyphenyl)naphthalene (8d). Yield = 76% (223 mg); colorless solid; mp = 118–120 °C (recrystallized from hexanes and EtOAc); HRMS (ESI, M+ + 1) calcd for C19H19O3 295.1334, found 295.1338; 1H NMR (400 MHz, CDCl3): δ 8.20 (d, J = 8.4 Hz, 1H), 7.82 (d, J = 8.4 Hz, 1H), 7.51–7.47 (m, 1H), 7.35 (d, J = 8.4 Hz, 2H), 7.33–7.29 (m, 1H), 7.23 (s, 1H), 7.41 (d, J = 8.8 Hz, 2H), 4.05 (s, 3H), 4.01 (s, 3H), 3.90 (s, 3H); 13C NMR (100 MHz, CDCl3): δ 159.00, 147.57, 142.10, 136.60, 132.85, 131.11 (2x), 129.34, 127.96, 126.12, 125.99, 124.05, 121.52, 116.36, 113.74 (2x), 61.14, 56.90, 55.36. Single-crystal X-ray diagram: crystal of compound 8d was grown by slow diffusion of EtOAc into a solution of compound 8d in CH2Cl2 to yield colorless prisms. The compound crystallizes in the monoclinic crystal system, space group P21/c, a = 13.3450(12) Å, b = 15.2538(14) Å, c = 7.5723(7) Å, V = 1518.9(2) Å3, Z = 4, dcalcd = 1.287 g cm−3, F(000) = 624, 2θ range 2.045–26.421°, R indices (all data) R1 = 0.1172, wR2 = 0.1285.
1,2-Dimethoxy-4-(4-trifluoromethylphenyl)naphthalene (8e). Yield = 80% (266 mg); colorless gum; HRMS (ESI, M+ + 1) calcd for C19H16F3O2 333.1102, found 333.1108; 1H NMR (400 MHz, CDCl3): δ 8.26 (d, J = 8.4 Hz, 1H), 7.78 (d, J = 8.0 Hz, 2H), 7.74 (d, J = 8.4 Hz, 1H), 7.63 (d, J = 8.0 Hz, 2H), 7.55–7.51 (m, 1H), 7.37–7.33 (m, 1H), 4.09 (s, 3H), 4.04 (s, 3H), 7.27 (s, 1H); 13C NMR (100 MHz, CDCl3): δ 147.54, 144.19, 142.97, 135.19, 130.41 (2x), 129.51 (d, J = 31.9 Hz), 129.40, 127.44, 126.26, 125.48, 125.23 (q, J = 3.8 Hz, 2x), 124.58, 124.28 (d, J = 270.6 Hz), 121.74, 116.60, 61.14, 56.97.
1,2-Dimethoxy-4-(2-methoxyphenyl)naphthalene (8f). Yield = 80% (235 mg); colorless gum; HRMS (ESI, M+ + 1) calcd for C19H19O3 295.1334, found 295.1336; 1H NMR (400 MHz, CDCl3): δ 8.20–8.17 (m, 1H), 7.51–7.46 (m, 2H), 7.44 (dd, J = 1.6, 8.0 Hz, 1H), 7.31–7.264 (m, 2H), 7.24 (s, 1H), 7.10 (dd, J = 1.2, 7.6 Hz, 1H), 7.07 (d, J = 8.0 Hz, 1H), 4.06 (s, 3H), 4.00 (s, 3H), 3.73 (s, 3H); 13C NMR (100 MHz, CDCl3): δ 157.22, 147.56, 142.21, 133.41, 132.04, 129.18, 129.06, 129.02, 128.23, 126.42, 125.80, 123.76, 121.38, 120.53, 116.74, 111.03, 61.11, 56.81, 55.56.
4-Biphenyl-4-yl-1,2-dimethoxynaphthalene (8g). Yield = 75% (255 mg); colorless solid; mp = 118–120 °C (recrystallized from hexanes and EtOAc); HRMS (ESI, M+ + 1) calcd for C24H21O2 341.1542, found 341.1548; 1H NMR (400 MHz, CDCl3): δ 8.24–8.21 (m, 1H), 7.90–7.88 (m, 1H), 7.74 (d, J = 8.4 Hz, 2H), 7.71–7.69 (m, 2H), 7.58 (d, J = 8.4 Hz, 2H), 7.54–7.48 (m, 3H), 7.42–7.38 (m, 1H), 7.36–7.32 (m, 1H), 7.30 (s, 1H), 4.07 (s, 3H), 4.03 (s, 3H); 13C NMR (100 MHz, CDCl3): δ 147.62, 142.42, 140.77, 140.21, 139.48, 136.47, 130.51 (2x), 129.39, 128.85 (2x), 127.76, 127.40, 127.12 (2x), 127.02 (2x), 126.11, 126.06, 124.23, 121.60, 116.42, 61.18, 56.95. Single-crystal X-ray diagram: crystal of compound 8g was grown by slow diffusion of EtOAc into a solution of compound 8g in CH2Cl2 to yield colorless prisms. The compound crystallizes in the triclinic crystal system, space group P
, a = 6.6111(3) Å, b = 9.8830(5) Å, c = 14.4700(8) Å, V = 867.14(8) Å3, Z = 2, dcalcd = 1.304 g cm−3, F(000) = 360, 2θ range 1.488–26.356°, R indices (all data) R1 = 0.0460, wR2 = 0.1231.
A representative procedure of skeleton 9 is as follows
Sn(OTf)2 (42 mg, 0.1 mmol) was added to a solution of 4w–x (1.0 mmol) in MeNO2 (5 mL) at 25 °C. The reaction mixture was stirred for 8 h at 25 °C and the solvent was concentrated. The residue was diluted with water (10 mL) and the mixture was extracted with CH2Cl2 (3 × 20 mL). The combined organic layers were washed with brine, dried, filtered and evaporated to afford crude product. Purification on silica gel (hexanes/EtOAc = 100/1–10/1) afforded 9.
6-Methoxy-1-(4-methoxyphenyl)naphthalene (9a). Yield = 61% (161 mg); colorless solid; mp = 83–85 °C (recrystallized from hexanes and EtOAc); HRMS (ESI, M+ + 1) calcd for C18H17O2 265.1229, found 265.1229; 1H NMR (400 MHz): δ 7.84 (d, J = 9.2 Hz, 1H), 7.74 (d, J = 8.4 Hz, 1H), 7.50–7.40 (m, 3H), 7.27 (dd, J = 1.2, 6.8 Hz, 1H), 7.20 (d, J = 2.8 Hz, 1H), 7.10 (dd, J = 2.8, 9.2 Hz, 1H), 7.05–7.01 (m, 2H), 3.95 (s, 3H), 3.90 (s, 3H); 13C NMR (100 MHz): δ 158.89, 157.39, 139.89, 135.10, 133.23, 131.02 (2x), 127.70, 127.23, 126.16, 126.03, 124.75, 118.52, 114.14, 113.67 (2x), 55.34, 55.30. Single-crystal X-ray diagram: crystal of compound 9a was grown by slow diffusion of EtOAc into a solution of compound 9a in CH2Cl2 to yield colorless prisms. The compound crystallizes in the monoclinic crystal system, space group P121/c1, a = 7.654(4) Å, b = 13.820(8) Å, c = 13.657(8) Å, V = 1429.0(14) Å3, Z = 4, dcalcd = 1.229 g cm−3, F(000) = 560, 2θ range 2.11–26.46°, R indices (all data) R1 = 0.0711, wR2 = 0.1581.
2,6-Dimethoxy-1-(4-methoxyphenyl)naphthalene (9b). Yield = 40% (118 mg); colorless solid; mp = 65–67 °C (recrystallized from hexanes and EtOAc); HRMS (ESI, M+ + 1) calcd for C19H19O3 295.1334, found 295.1335; 1H NMR (400 MHz, CDCl3): δ 7.82 (d, J = 8.8 Hz, 1H), 7.76 (d, J = 8.8 Hz, 1H), 7.51–7.41 (m, 2H), 7.27 (dd, J = 1.2, 6.8 Hz, 1H), 7.21 (d, J = 2.4 Hz, 1H), 7.11 (dd, J = 2.8, 9.2 Hz, 1H), 7.02–6.96 (m, 2H), 3.98 (s, 3H), 3.97 (s, 3H), 3.90 (s, 3H). Single-crystal X-ray diagram: crystal of compound 9b was grown by slow diffusion of EtOAc into a solution of compound 9b in CH2Cl2 to yield colorless prisms. The compound crystallizes in the monoclinic crystal system, space group C1c1, a = 8.6905(3) Å, b = 21.9250(7) Å, c = 8.2652(3) Å, V = 1494.97(9) Å3, Z = 4, dcalcd = 1.308 g cm−3, F(000) = 624, 2θ range 1.86–26.52°, R indices (all data) R1 = 0.0291, wR2 = 0.0743.
Naphthalene (10a). Na2CO3 (106 mg, 1.0 mmol) was added to a solution of allyl bromide (240 mg, 2.0 mmol) in dimethoxyethane (DME, 5 mL) at 25 °C. The reaction mixture was stirred at 25 °C for 5 min. Then, o-formylphenylboronic acid (150 mg, 1.0 mmol) was added to the reaction mixture at 25 °C. The reaction mixture was stirred at 25 °C for 5 min. Pd(OAc)2 (11 mg, 0.05 mmol) was added to the reaction mixture at 25 °C. The reaction mixture was stirred at 80 °C for 5 h. The reaction mixture (in situ formed 4y) was cooled to 25 °C and Sn(OTf)2 (42 mg, 0.1 mmol) was added to the reaction mixture. The reaction mixture was stirred for 8 h at 25 °C and the solvent was concentrated. The residue was diluted with water (10 mL) and the mixture was extracted with CH2Cl2 (3 × 20 mL). The combined organic layers were washed with brine, dried, filtered and evaporated to afford crude product. Purification on silica gel (hexanes/EtOAc = 100/1–10/1) afforded 10a (51%, 65 mg).
Acknowledgements
The authors would like to thank the Ministry of Science and Technology of the Republic of China for its financial support (MOST 105-2113-M-037-001).
Notes and references
- For reviews on the synthesis of naphthalenes, see:
(a) C. B. de Koning, A. L. Rousseau and W. A. L. van Otterlo, Tetrahedron, 2003, 59, 7–36 CrossRef CAS
. For reviews on the benzannulation of naphthalenes, see:
(b) S. Kotha and S. M. Somnath-Halder, Tetrahedron, 2008, 64, 10775–10790 CrossRef CAS
. - Bioactive molecules with the core naphthalene skeleton, see:
(a) T. Ukita, Y. Nakamura, A. Kubo, Y. Yamomoto, M. Takahashi, J. Kotera and T. Ikeo, J. Med. Chem., 1999, 42, 1293–1305 CrossRef CAS PubMed
;
(b) H. Yeo, Y. Li, L. Fu, J.-L. Zhu, E. A. Gullen, G. E. Dutschman, Y. Lee, R. Chung, E.-S. Huang, D. J. Austin and Y.-C. Cheng, J. Med. Chem., 2005, 48, 534–546 CrossRef CAS PubMed
;
(c) W. Shen, X. Zou, M. Chen, P. Liu, Y. Shen, S. Huang, H. Guo and L. Zhang, Eur. J. Pharmacol., 2011, 667, 330–338 CrossRef CAS PubMed
;
(d) J. Aszno, K. Chiba, M. Tada and T. Yoshii, Phytochemistry, 1996, 42, 713–717 CrossRef
. - Natural products with the core naphthalene skeleton, see:
(a) R. S. Ward, Nat. Prod. Rep., 1999, 16, 75–96 RSC
;
(b) S. Apers, A. Vlietinck and L. Pieters, Phytochem. Rev., 2003, 2, 201–217 CrossRef CAS
;
(c) N. Abdissa, F. Pan, A. Gruhonjic, J. Grafenstein, P. A. Fitzpatrick, G. Landberg, K. Rissanen, A. Yenesew and M. Erdelyi, J. Nat. Prod., 2016, 79, 2181–2187 CrossRef CAS PubMed
;
(d) L. Ding, S. Fotso, F. Li, S. Qin and H. Laatsch, J. Nat. Prod., 2008, 71, 1068–1069 CrossRef CAS PubMed
. - Functionalized materials with the core naphthalene skeleton, see:
(a) K.-T. Lin, H.-M. Kuo, H.-S. Sheu and C.-K. Lai, Tetrahedron, 2013, 69, 9045–9055 CrossRef CAS
;
(b) M. Tanaka, B. Elias and J. K. Barton, J. Org. Chem., 2010, 75, 2423–2428 CrossRef CAS PubMed
;
(c) C. Thalacker, C. Röger and F. Würthner, J. Org. Chem., 2006, 71, 8098–8105 CrossRef CAS PubMed
;
(d) J. G. Rodriguez and J. L. Tejedor, J. Org. Chem., 2002, 67, 7631–7640 CrossRef CAS PubMed
;
(e) S. Chowdhury and P. E. Georghiou, J. Org. Chem., 2002, 67, 6808–6811 CrossRef CAS PubMed
;
(f) C. Röger and F. Würthner, J. Org. Chem., 2007, 72, 8070–8075 CrossRef PubMed
. - Acids or Lewis acids mediated synthesis of naphthalene skeleton via intramolecular Friedel–Crafts annulation, see:
(a) J. Wennerburg, C. Olofsson and T. Frejd, J. Org. Chem., 1998, 63, 3595–3598 CrossRef
;
(b) A. I. Meyers and J. J. Willemsen, Tetrahedron, 1998, 54, 10493–10511 CrossRef CAS
;
(c) E. A. Couladouros and A. T. Strongilos, Tetrahedron Lett., 2000, 41, 535–538 CrossRef CAS
;
(d) G. Bringmann, M. Ochse and R. Götz, J. Org. Chem., 2000, 65, 2069–2077 CrossRef CAS PubMed
;
(e) D. L. Boger and P. Turnbull, J. Org. Chem., 1998, 63, 8004–8011 CrossRef CAS
;
(f) A. V. Kelin and Y. Y. Kozyrkov, Synthesis, 1998, 729–734 CrossRef CAS
;
(g) A. H. Schmidt, G. Kircher and E. Brau, J. Org. Chem., 1998, 63, 1954–1960 CrossRef CAS
;
(h) T. R. Hoye and L. Mi, J. Org. Chem., 1997, 62, 8586–8588 CrossRef CAS PubMed
;
(i) A. R. Katritzky, G. Zhang and L. Xie, J. Org. Chem., 1997, 62, 721–725 CrossRef CAS PubMed
;
(j) M. A. Ciufolini and T. J. Weiss, Tetrahedron Lett., 1994, 35, 1127–1130 CrossRef CAS
;
(k) I. Hanna, V. Michaut and L. Ricard, Tetrahedron Lett., 2001, 42, 231–234 CrossRef CAS
;
(l) S. Kajikawa, H. Nishino and K. Kurosawa, Tetrahedron Lett., 2001, 42, 3351–3354 CrossRef CAS
;
(m) M.-Y. Chang, Y.-H. Huang and H.-S. Wang, Tetrahedron, 2016, 72, 1888–1895 CrossRef CAS
;
(n) S. Ponra, M. R. Vitale, V. Michelet and V. Ratovelomanana-Vidal, J. Org. Chem., 2015, 80, 3250–3257 CrossRef CAS PubMed
. - Bases promoted synthesis of naphthalene skeleton via intramolecular anionic annulation, for 2-allylbenzamides/MeLi, see:
(a) M. P. Sibi, J. W. Dankwardt and V. Snieckus, J. Org. Chem., 1986, 51, 271–273 CrossRef CAS
;
(b) J.-m. Fu and V. Snieckus, Can. J. Chem., 2000, 78, 905–919 CrossRef CAS
;
(c) X. Wang and V. Snieckus, Tetrahedron Lett., 1991, 32, 4879–4882 CrossRef CAS
. For 2-allylbenzoates/MeONa, see:
(d) T. Hattori, A. Takeda, K. Suzuki, N. Koike, E. Koshiishi and S. Miyano, J. Chem. Soc., Perkin Trans. 1, 1998, 3661–3672 RSC
. For 2-allylbenzoketones/t-BuOK, see:
(e) C. B. de Koning, J. P. Michael and A. L. Rousseau, J. Chem. Soc., Perkin Trans. 1, 2000, 787–790 RSC
;
(f) C. B. de Koning, J. P. Michael and A. L. Rousseau, Tetrahedron Lett., 1997, 38, 893–896 CrossRef CAS
;
(g) C. B. de Koning, S. S. Manzini, J. P. Michael, E. M. Mmutlane, T. R. Tshabidi and W. A. L. van Otterlo, Tetrahedron, 2005, 61, 555–564 CrossRef CAS
. For 2-allylbenzoketones/DBU, see:
(h) M.-Y. Chang and M.-H. Wu, Tetrahedron, 2013, 69, 129–136 CrossRef CAS
; For 2-alkynylbenzoketones/KHMDS, see:
(i) F. Makra, J. C. Rohloff, A. V. Muehldorf and J. O. Link, Tetrahedron Lett., 1995, 36, 6815–6818 CrossRef CAS
. - Transition metals promoted the synthesis of naphthalene skeleton, for chromium, see:
(a) C. A. Merlic, C. C. Aldrich, J. Albaneze-Walker and A. Saghatelian, J. Am. Chem. Soc., 2000, 122, 3224–3225 CrossRef CAS PubMed
;
(b) C. A. Merlic, C. C. Aldrich, J. Albaneze-Walker, A. Saghatelian and J. Mammen, J. Org. Chem., 2001, 66, 1297–1309 CrossRef CAS PubMed
;
(c) M. W. Davies, C. N. Johnson and J. P. A. Harrity, J. Org. Chem., 2001, 66, 3525–3532 CrossRef CAS PubMed
;
(d) W. H. Moser, L. Sun and J. C. Huffman, Org. Lett., 2001, 3, 3389–3391 CrossRef CAS PubMed
. For manganese, see:
(e) B. B. Snider and Q. Zhang, J. Org. Chem., 1993, 58, 3185–3187 CrossRef CAS
;
(f) J. F. Jamie and R. W. Rickards, J. Chem. Soc., Perkin Trans. 1, 1996, 2603–2613 RSC
;
(g) A.-I. Tsai, Y.-L. Wu and C.-P. Chuang, Tetrahedron, 2001, 57, 7829–7837 CrossRef CAS
. For palladium, see:
(h) E. Yoshikawa, K. V. Radhakrishnan and Y. Yamamoto, J. Am. Chem. Soc., 2000, 122, 7280–7286 CrossRef CAS
;
(i) D. Pena, D. Perez, E. Guitian and L. Castedo, J. Org. Chem., 2000, 65, 6944–6950 CrossRef CAS PubMed
;
(j) R. C. Larock, M. J. Doty, Q. Tian and J. M. Zenner, J. Org. Chem., 1997, 62, 7536–7537 CrossRef CAS
;
(k) R. C. Larock and Q. Tian, J. Org. Chem., 1998, 63, 2002–2009 CrossRef CAS
;
(l) H. Mizufune, M. Nakamura and H. Mitsudera, Tetrahedron Lett., 2001, 42, 437–439 CrossRef CAS
. For tungsten, see:
(m) N. Iwasawa, M. Shido, K. Maeyama and H. Kusama, J. Am. Chem. Soc., 2000, 122, 10226–10227 CrossRef CAS
;
(n) K. Maeyama and N. Iwasawa, J. Org. Chem., 1999, 64, 1344–1346 CrossRef CAS
. For rhodium, see:
(o) J. W. Dankwardt, Tetrahedron Lett., 2001, 42, 5809–5812 CrossRef CAS
. For cobalt, see:
(p) Y. Kita, K. Iio, K. i. Kawaguchi, N. Fukuda, Y. Takeda, H. Ueno, R. Okunaka, K. Higuchi, T. Tsujino, H. Fujioka and S. Akai, Chem.–Eur. J., 2000, 6, 3897–3905 CrossRef CAS PubMed
. For ruthenium, see:
(q) P. Evans, R. Grigg, M. I. Ramzan, V. Sridharan and M. York, Tetrahedron Lett., 1999, 40, 3021–3024 CrossRef CAS
. For nickel, see:
(r) M. A. Bennett, C. J. Cobley, E. Wenger and A. C. Willis, Chem. Commun., 1998, 1307–1308 RSC
. For copper, see:
(s) J. W. Lim, K. H. Kim, S. H. Kim and J. N. Kim, Tetrahedron Lett., 2012, 53, 5449–5454 CrossRef CAS
. For zinc, see:
(t) S. Sakthivel and K. Srinivasan, J. Org. Chem., 2014, 79, 3244–3248 CrossRef PubMed
. For titanium, see:
(u) G. W. Kabalka, Y. Ju and Z. Wu, J. Org. Chem., 2003, 68, 7915–7917 CrossRef CAS PubMed
. For gold, see:
(v) A. R. Jagdale, J. H. Park and S. W. Youn, J. Org. Chem., 2011, 76, 7204–7215 CrossRef CAS PubMed
. - Synthetic applications of o-formyl and o-aroyl allylbenzenes by the authors, see:
(a) M.-Y. Chang and N.-C. Lee, Synlett, 2012, 23, 867–872 CrossRef CAS
;
(b) M.-Y. Chang, M.-H. Wu, N.-C. Lee and M.-F. Lee, Tetrahedron Lett., 2012, 53, 2125–2128 CrossRef CAS
;
(c) M.-Y. Chang and M.-H. Wu, Tetrahedron Lett., 2012, 53, 3173–3177 CrossRef CAS
;
(d) M.-Y. Chang, M.-H. Wu and T.-W. Lee, Tetrahedron, 2012, 68, 6224–6230 CrossRef CAS
;
(e) M.-Y. Chang, M.-H. Wu and Y.-L. Chen, Tetrahedron Lett., 2012, 53, 4156–4160 CrossRef CAS
;
(f) M.-Y. Chang, H.-Y. Tai, Y.-L. Chen and R.-T. Hsu, Tetrahedron, 2012, 68, 7941–7948 CrossRef CAS
;
(g) M.-Y. Chang, M.-H. Wu and H.-Y. Tai, Org. Lett., 2012, 14, 3936–3939 CrossRef CAS PubMed
;
(h) M.-Y. Chang, C.-K. Chan, S.-Y. Lin and R.-T. Hsu, Tetrahedron, 2012, 68, 10272–10279 CrossRef CAS
;
(i) M.-Y. Chang and M.-H. Wu, Tetrahedron, 2013, 69, 129–136 CrossRef CAS
;
(j) M.-Y. Chang, C.-K. Chan and S.-Y. Lin, Tetrahedron, 2013, 69, 1532–1538 CrossRef CAS
;
(k) M.-Y. Chang, M.-H. Wu and Y.-L. Chen, Org. Lett., 2013, 15, 2822–2825 CrossRef CAS PubMed
;
(l) C.-K. Chan, Y.-L. Chan and M.-Y. Chang, Tetrahedron, 2016, 72, 547–554 CrossRef CAS
;
(m) C.-K. Chan, Y.-L. Tsai, Y.-L. Chan and M.-Y. Chang, J. Org. Chem., 2016, 81, 9836–9847 CrossRef CAS PubMed
;
(n) C.-K. Chan, Y.-H. Chen, Y.-L. Tsai and M.-Y. Chang, J. Org. Chem., 2017, 82, 3317–3326 CrossRef CAS PubMed
;
(o) C.-K. Chan, Y.-L. Tsai and M.-Y. Chang, Org. Lett., 2017, 19, 1358–1361 CrossRef CAS PubMed
;
(p) C.-K. Chan, Y.-L. Tsai and M.-Y. Chang, Org. Lett., 2017, 19, 1870–1873 CrossRef CAS PubMed
. - Metal Triflates mediated synthetic applications by the authors, for Bi(OTf)3, see:
(a) M.-Y. Chang, Y.-C. Cheng and Y.-J. Lu, Org. Lett., 2015, 17, 3142–3145 CrossRef CAS PubMed
;
(b) M.-Y. Chang, Y.-C. Cheng and Y.-J. Lu, Org. Lett., 2015, 17, 1264–1267 CrossRef CAS PubMed
;
(c) C.-K. Chan, Y.-C. Chen, Y.-L. Chen and M.-Y. Chang, Tetrahedron, 2015, 71, 9187–9195 CrossRef CAS
. For In(OTf)3, see:
(d) M.-Y. Chang, Y.-J. Lu and Y.-C. Cheng, Tetrahedron, 2015, 71, 6840–6845 CrossRef CAS
. For Sc(OTf)3, see:
(e) M.-Y. Chang, Y.-C. Chen, C.-K. Chan and G. G. Huang, Tetrahedron, 2015, 71, 2095–2104 CrossRef CAS
. For Fe(OTf)3, see:
(f) M.-Y. Chang, Y.-H. Chen and Y.-C. Cheng, Tetrahedron, 2016, 72, 518–524 CrossRef CAS
. For Sn(OTf)2, see:
(g) C.-K. Chan, Y.-H. Chen and M.-Y. Chang, Tetrahedron, 2016, 72, 5121–5131 CrossRef CAS
. For Ga(OTf)3, see:
(h) H.-S. Wang, C.-K. Chan and M.-Y. Chang, Tetrahedron, 2016, 72, 5132–5141 CrossRef CAS
. For Tm(OTf)3, see:
(i) M.-Y. Chang and Y.-C. Cheng, Synlett, 2016, 27, 1931–1935 CrossRef CAS
. - Metal triflates promoted intramolecular annulation, for o-benzylbenzaldehydes/In(OTf)3, see:
(a) Y. Kuninobu, T. Tatsuzaki, T. Matsuki and K. Takai, J. Org. Chem., 2011, 76, 7005–7009 CrossRef CAS PubMed
. For 2′-allylchalcones/Cu(OTf)2, see:
(b) Y. Qin, S. Luo and J.-P. Cheng, Org. Lett., 2014, 16, 5032–5035 CrossRef CAS PubMed
. - For reviews on metal triflates-mediated reactions, see:
(a) U. Landziata, ARKIVOC, 2014, i, 307–336 Search PubMed
;
(b) S. Luo, Z. Lizhi, A. Talukdar, G. Zhang, M. Xueling, J.-P. Cheng and P. G. Wang, Mini-Rev. Org. Chem., 2005, 2, 177–202 CrossRef CAS
;
(c) S. Kobayashi, M. Sugimura, H. Kitagawa, L. Hidetoshi and W. W.-L. Lam, Chem. Rev., 2002, 102, 2227–2302 CrossRef CAS PubMed
. - Selected examples on the metal triflates mediated benzannulation, for In(OTf)3, see:
(a) R. Yanada, K. Hashimoto, R. Tokizane, Y. Miwa, H. Minami, K. Yanada, M. Ishikura and Y. Takemoto, J. Org. Chem., 2008, 73, 5135–5138 CrossRef CAS PubMed
. For Yb(OTf)3, see:
(b) X.-S. Wang, J. Zhou, K. Yang and C.-S. Yao, Tetrahedron Lett., 2010, 51, 5721–5723 CrossRef CAS
. For Sc(OTf)3, see:
(c) Y. Nishina, T. Kida and T. Ureshino, Org. Lett., 2011, 13, 3960–3963 CrossRef CAS PubMed
. For Nd(OTf)3, see:
(d) C. Sabot, E. Queis, X. Brune and P.-Y. Renard, Chem. Commun., 2012, 48, 768–770 RSC
. For Cu(OTf)2, see: H. Arslan, F. J. Uribe-Romo, B. J. Smith and W. R. Ditchtel, Chem. Sci., 2013, 4, 3973–3978 RSC
For Fe(OTf)3, see:
(e) K. Bera, S. Sarkar and U. Jana, Tetrahedron Lett., 2015, 56, 312–315 CrossRef CAS
. - Sn(OTf)2-mediated reactions, for aldol reactions, see:
(a) S. Kobayashi and I. Hachiya, J. Org. Chem., 1992, 57, 1324–1326 CrossRef CAS
. For Mannish-type reactions, see:
(b) N. A. Kulkarni and K. Chen, Tetrahedron Lett., 2006, 47, 611–613 CrossRef CAS
;
(c) Y. Yang, D. P. Phillips and S. Pan, Tetrahedron Lett., 2011, 52, 1549–1552 CrossRef CAS
. For rearrangements, see:
(d) G.-C. Xu, M. Ma, L.-P. Liu and M. Shi, Synlett, 2005, 1869–1872 CAS
;
(e) T. H. Lambert and D. W. C. MacMillan, J. Am. Chem. Soc., 2002, 124, 13646–13647 CrossRef CAS PubMed
. For (3 + 2) annulations, see:
(f) A. G. Smith, M. C. Slade and J. S. Johnson, Org. Lett., 2011, 13, 1996–1999 CrossRef CAS PubMed
;
(g) D. P. Kranz, G. A. Meierzu, S. El Sheikh, J. M. Neudrfl and H. G. Schmalz, Eur. J. Org. Chem., 2011, 15, 2860–2866 CrossRef
;
(h) A. F. G. Goldberg, N. R. O'Connor, R. A. Craig II and B. M. Stoltz, Org. Lett., 2012, 14, 5314–5317 CrossRef CAS PubMed
. For other routes, see:
(i) D. Ferraris, W. J. Drury III, C. Cox and T. Lectka, J. Org. Chem., 1998, 63, 4568–4569 CrossRef CAS
;
(j) G. Mancilla, M. Femenía-Rios, A. J. Macías-Sánchez and I. G. Collado, Tetrahedron, 2008, 64, 11732–11737 CrossRef CAS
;
(k) B. N. Kakde, N. Kumar, P. K. Mondal and A. Bisai, Org. Lett., 2016, 18, 1752–1755 CrossRef CAS PubMed
. - CCDC 923283 (5e), 923284 (5f), 1519809 (8d), 1523594 (8g), 790259 (9a) and 923282 (9b) contain the supplementary crystallographic data for this paper.†.
- Selected examples on the metal triflates mediated Claisen rearrangement, for Zn(OTf)2, see:
(a) M. C. Pirrung and J. N. Nalbandian, Tetrahedron Lett., 2013, 54, 3752–3754 CrossRef CAS PubMed
. For Cu(OTf)2 and Yb(OTf)3, see:
(b) M. Hiersemann and L. Abraham, Org. Lett., 2001, 3, 49–52 CrossRef CAS PubMed
. For Bi(OTf)3, see:
(c) B. Sreedar, V. Swapna and C. Sridhar, Synth. Commun., 2004, 34, 1433–1440 CrossRef
. - For reviews on the Claisen rearrangement, see:
(a) A. M. Castro, Chem. Rev., 2004, 104, 2939–3002 CrossRef CAS PubMed
;
(b) U. Nubbemeyer, Synthesis, 2003, 961–1008 CAS
;
(c) M. Hiersemann and L. Abraham, Eur. J. Org. Chem., 2002, 1461–1471 CrossRef CAS
;
(d) Y. Chai, S. Hong, H. A. Lindsay, C. MaFarland and M. C. McIntosh, Tetrahedron, 2002, 58, 2905–2928 CrossRef CAS
. - Selected examples on the concept of a Lewis acid assisted Brønsted acid (LBA), see:
(a) H. Yamamoto and K. Futatsugi, Angew. Chem., Int. Ed., 2005, 44, 1924–1942 CrossRef CAS PubMed
;
(b) C. H. Cheon, O. Kanno and F. D. Toste, J. Am. Chem. Soc., 2011, 133, 13248–13251 CrossRef CAS PubMed
;
(c) O. Kanno, W. Kuriyama, Z. J. Wang and F. D. Toste, Angew. Chem., Int. Ed., 2011, 50, 9919–9922 CrossRef CAS PubMed
. -
(a) S. Kobayashi, S. Nagayama and T. Busujima, J. Am. Chem. Soc., 1998, 120, 8287–8288 CrossRef CAS
;
(b) T. C. Wabnitz, J.-Q. Yu and J. B. Spencer, Chem.–Eur. J., 2004, 10, 484–493 CrossRef CAS PubMed
;
(c) D. C. Rosenfeld, S. Shekhar, A. Takemiya, M. Utsunomiya and J. F. Hartwig, Org. Lett., 2006, 8, 4179–4182 CrossRef CAS PubMed
;
(d) B. Cacciuttolo, S. Poulain-Martni, F. Fontaine-Vive, M. A. H. Abdo, H. El-Kashef and E. Duñach, Eur. J. Org. Chem., 2014, 7458–7468 CrossRef CAS
. -
(a) C. F. Baes Jr and R. E. Mesmer, The Hydrolysis of Cations, Wiley-Interscience, 1976 Search PubMed
;
(b) G. Lèmiere, B. Cacciuttolo, E. Belhassen and E. Duñach, Org. Lett., 2012, 14, 2750–2753 CrossRef PubMed
;
(c) T. Ollevier and E. Nadeau, Org. Biomol. Chem., 2007, 5, 3126–3134 RSC
;
(d) S. Répichet, A. Zwick, L. Vendier, C. Le Roux and J. Dubac, Tetrahedron Lett., 2002, 43, 993–995 CrossRef
.
|
This journal is © The Royal Society of Chemistry 2017 |