Abstract
A series of C-glycosylated cinnamoylfuran derivatives were synthesized in excellent yield from free sugars. The C-glycosylated furan derivatives were prepared under aqueous reaction conditions. The anticancer potentials of the synthesized compounds were evaluated on the basis of their comparative cytotoxic effects on cancer cells (MCF-7 and HeLa) and normal cells (NKE). Among 28 compounds evaluated for their cytotoxic effects on cancer cells, three compounds (compounds 8, 24 and 28) were shown to have significant cytotoxic effects on MCF-7 and HeLa cell lines and comparatively less toxicity against normal NKE cell line. Based on its selectivity index, compound 24 was considered the most promising anticancer agent amongst the above three compounds. Further biochemical studies with compound 24 showed that both intrinsic and extrinsic pathways of apoptosis contribute to compound 24 mediated cytotoxicity.
Cytotoxic effect of C-glycosyl cinnamoylfuran derivatives by MTT cell proliferation assay. The newly synthesized C-glycosyl-3-cinnamoylfuran derivatives (7–34) were screened in vitro for their anti-proliferative effect on MCF-7 (Human breast carcinoma), HeLa (Human cervical carcinoma) and NKE (Normal Kidney Epithelial) cells. MCF-7 is the most studied human breast cancer cell line and results from this cell line have had a fundamental impact upon breast cancer research and patient outcomes. The MCF-7 cell line is one of a very few breast cancer cell lines that expresses substantial levels of ER, mimicking the majority of invasive human breast cancers that express ER.25 Similarly, HeLa has been widely used as a model for cervical cancer over the past 50 years of cervical cancer research.26 Owing to these facts we chose these two cells lines for our experiments. Etoposide was used as a standard control, considering its tremendous anti-proliferative activity.27 In this experiment, MCF-7, HeLa and NKE cells were treated with various concentrations (0 μM, 5 μM, 10 μM, 20 μM, 30 μM and 50 μM) of the C-glycoside derivatives and the cell viability was measured by MTT assay.28 The results are summarized in Table 2 and expressed in terms of IC50 values (half-maximal inhibitory concentration).
Table 2 The IC50 (μM) values of the compounds for the inhibition of proliferationa
Compd |
MCF-7 |
HeLa |
NKE |
IC50 = concentration at which 50% inhibition in motility was achieved. |
7 |
≥60 |
≥60 |
— |
8 |
17.77 ± 1.46 |
15.98 ± 1.85 |
46.03 ± 10.56 |
9 |
≥60 |
10.93 ± 5.36 |
109 ± 7.85 |
10 |
64.54 ± 13.16 |
≥60 |
75.07 ± 27.83 |
11 |
≥60 |
≥60 |
— |
12 |
≥60 |
≥60 |
— |
13 |
≥60 |
≥60 |
— |
14 |
68.75 ± 12.84 |
81.48 ± 76.22 |
≥60 |
15 |
90.77 ± 105.53 |
35.93 ± 10.13 |
59.05 ± 59.05 |
16 |
54.07 ± 6.49 |
≥60 |
≥60 |
17 |
≥60 |
≥60 |
— |
18 |
≥60 |
≥60 |
— |
19 |
43.49 ± 7.39 |
47.58 ± 10.37 |
≥60 |
20 |
≥60 |
≥60 |
— |
21 |
45.37 ± 14.31 |
≥60 |
99.92 ± 27.89 |
22 |
33.0 ± 1.5 |
≥60 |
24.38 ± 11.38 |
23 |
≥60 |
≥60 |
— |
24 |
9.588 ± 5.25 |
14.22 ± 3.94 |
54.14 ± 1.56 |
25 |
≥60 |
≥60 |
— |
26 |
≥60 |
≥60 |
— |
27 |
32.35 ± 3.6 |
≥60 |
≥60 |
28 |
22.98 ± 4.19 |
22.29 ± 4.03 |
40.29 ± 11.87 |
29 |
34.79 ± 6.86 |
≥60 |
≥60 |
30 |
≥60 |
≥60 |
— |
31 |
43.84 ± 7.66 |
30.25 ± 6.76 |
30.23 ± 23 |
32 |
≥60 |
≥60 |
— |
33 |
91.27 ± 53.63 |
≥60 |
23.84 ± 17.45 |
34 |
≥60 |
≥60 |
— |
Etoposide |
24.77 ± 3.4 |
29.12 ± 8.56 |
— |
Out of 28 tested compounds, compound 8 (IC50: 17.77 ± 1.46 μM), compound 24 (IC50: 9.588 ± 5.25 μM) and compound 28 (IC50: 22.98 ± 4.19 μM) showed higher efficacy than etoposide (IC50: 24.77 ± 3.4 μM) in MCF-7 cells. In another set of HeLa cells, compound 8 (IC50: 15.98 ± 1.85 μM), compound 24 (IC50: 14.22 ± 3.94 μM), compound 28 (IC50: 22.29 ± 4.03 μM) and also compound 9 (IC50: 10.93 ± 5.36 μM) exerted lower IC50 as compared to etoposide (IC50: 29.12 ± 8.56 μM). From these results, it was found that compound 24 was more potent in both MCF-7 and HeLa cells as compared to compound 8 and compound 28, whereas compound 9 was only effective in HeLa cells. Consequently, an evaluation of the cytotoxic effect of compounds 8, 24 and 28 against NKE cells showed significantly higher IC50 values than their IC50 values against MCF-7 and HeLa cells [compound 24 (IC50: 54.14 ± 1.56 μM), compound 8 (IC50: 46.03 ± 10.56 μM) and compound 28 (IC50: 40.29 ± 11.87 μM)]. Subsequently, when the selectivity indexes (SI) were calculated based on the IC50 value ratio of compounds in NKE vs. MCF-7, it was found that compound 24 (SI = 7.93 ± 4.18) possessed higher SI in comparison to compound 8 (SI = 2.56 ± 0.38) and compound 28 (SI = 4.05 ± 0.06). In the case of HeLa cells, compound 24 also showed a better SI value than the other two compounds [compound 24 (SI = 3.42 ± 0.30), 8 (SI = 3.33 ± 0.34) and 28 (SI = 1.71 ± 0.20)].
The activity of compound 24 was further tested against MDA-MB-231 cells. Interestingly, it was also observed that compound 24 was very effective in inhibiting the proliferation of MDA-MB-231 cells and the calculated IC50 value was 19.16 ± 5.97 μM (Fig. 1). Based on the preliminary MTT experimental data and selectivity index, compound 24 has been selected as a promising anti-cancer agent and considered for further evaluation.
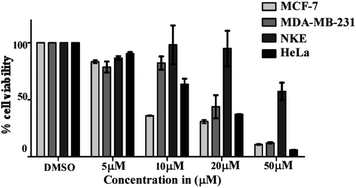 |
| Fig. 1 Determination of dose-dependent cytotoxicity of compound 24 on various human cell lines. | |