DOI:
10.1039/C7RA03831C
(Paper)
RSC Adv., 2017,
7, 27177-27188
Synthesis of aluminum complexes supported by 2-(1,10-phenanthrolin-2-yl)phenolate ligands and their catalysis in the ring-opening polymerization of cyclic esters†
Received
4th April 2017
, Accepted 17th May 2017
First published on 23rd May 2017
Abstract
A series of aluminum complexes supported by 2-(1,10-phenanthrolin-2-yl)phenolate ligands were synthesized and characterized. The reaction of o-bromophenol with n-butyllithium followed by nucleophilic addition to the phenanthroline and oxidation process gave the ligand precursors 2-(1,10-phenanthrolin-2-yl)phenol derivatives 1a–1d. Treatment of 1a–1d with an equiv. of AlR3 (R = Et, Bui) afforded the corresponding aluminum aryloxides 2a–2e. Treatment of 2b with 2 equiv. of benzyl alcohol formed complex 2f. All new compounds were characterized by 1H and 13C NMR spectroscopy and elemental analyses. The structure of complex 2c was further characterized by single crystal X-ray diffraction techniques. Complexes 2a–2e/BnOH and 2f were active to catalyze the ring-opening polymerization of ε-caprolactone, leading to polycaprolactone with good molecular weight control and relatively narrow molecular weight distribution. Complexes 2a–2e/BnOH and 2f catalyzed the polymerization of rac-lactide and 2b, 2c, 2e and 2f were demonstrated to lead to isotactic enriched polylactides. The above catalysts also catalyzed the ROP of rac-β-butyrolactone and led to atactic poly(hydroxybutyrate). Complex 2f also catalyzed block copolymerization of the cyclic esters to form PCL-b-PHB, PCL-b-PLA, and PHB-b-PLA block copolymers.
Introduction
Aliphatic polyesters such as poly(lactic acid) (PLA) and poly(ε-caprolactone) (PCL), as well as their copolymers have attracted considerable attention due to their biocompatible, biodegradable, and permeable properties which are important in biomedical and pharmaceutical applications.1 PLA, whose starting materials can be derived from renewable resources such as corn and sugar beet, is a promising material for an attractive alternative to petrochemical-derived polyolefin.1d–f,2 PCL shows specific advantages such as its miscibility with different polymers, its adhesive properties at low temperature, and its ability to disperse pigments. It is also ideally suited for long-term drug delivery due to its slow degradation in comparison to other polymers.1c,3 Poly(β-hydroxybutyrate) (PHB) as an environmentally friendly polymer material offers many advantages over traditional petrochemically derived plastics. For example, it possesses better physical properties than polypropylene for food packaging applications and is nontoxic and biodegradable. PLA and PCL are generally synthesized by the ring-opening polymerization (ROP) of corresponding cyclic esters initiated by metal complexes. PHB is mainly produced using bacterial fermentation techniques from renewable resources.1b,1g,4 It can also be synthesized via catalytic ROP of β-butyrolactone (β-BL). The metal complexes for the ROP of the cyclic esters include alkali metals,5 alkaline-earth metals,6 group IIIA metals,7 group IVA metals,8 rare earth metals,9 and transition metals10 complexes, etc. However, different catalysts can lead to the polymers with different molecular weights, polydispersity indices, and stereo-microstructures. Hence search for new catalysts for synthesis of the polymers is still an important topic. In the previous studies on metal catalysts for the ROP of cyclic esters, aluminum complexes have attracted considerable attention due to oxophilicity and Lewis acidity of the metal center. Numerous Al complexes bearing a range of multidentate ligands including NO, ONO, NNO, ONNO, and NNNN frameworks have been evaluated.11 We reported several aluminum complexes bearing pyridine- or quinoline-based NNO-chelate ligands and demonstrated them to be able to catalyze the ROP of ε-caprolactone (ε-CL) in the presence of benzyl alcohol.8c,12 However, the complexes were inactive towards the ROP of rac-lactide (rac-LA) in the presence or absence of BnOH. Based on our previous work and the results reported in literatures,7k,l,8c,12 we designed and synthesized aluminum complexes supported by 2-(1,10-phenanthrolin-2-yl)phenolate ligands and found these complexes to be active catalysts in the ROP of rac-LA, ε-CL and rac-β-BL. Here we report the results.
Results and discussion
Synthesis and characterization of complexes 2a–2f
Synthetic routes of the ligand precursors and the complexes are summarized in Scheme 1. 2-(1,10-Phenanthrolin-2-yl)phenol derivatives 1a–1d were synthesized according to improved literature procedures and characterized by 1H and 13C NMR spectroscopy and elemental analysis.13 Reaction of 1a–1d with AlEt3 in toluene at room temperature afforded complexes 2a–2d, respectively. 2e was similarly prepared by treatment of 1b with triisobutylaluminum in toluene. 2f was obtained via reaction of 1b with 1.1 equiv. of AlEt3 followed by in situ alcoholysis of the resultant complex with 2 equiv. of BnOH at room temperature. Complexes 2a–2d are soluble in toluene and can be crystallized from the toluene solutions. Complex 2f was crystallized from a diethyl ether solution. Complexes 2a–2f were characterized by 1H and 13C NMR spectroscopy and elemental analyses. The analytical results are accordant with their respective formula. The 1H NMR spectrum of each of 2a–2e exhibits two sets of Al–R signals, which correspond two Al–R groups in different chemical environments. This might result from the fact that the phenanthroline ring and the phenolic ring are noncoplanar. The 1H NMR spectrum of complex 2f exhibits two sets of Al–OCH2Ph signals due to similar reason. But we cannot rule out the possibility of a dimeric structure. Attempts to grow its single crystals for X-ray diffraction analysis were unsuccessful. Complex 2c was further characterized by single crystal X-ray diffraction. The ORTEP drawing is presented in Fig. 1, along with selected bond lengths and angles. In the molecule, the aluminum atom is five-coordinate and the coordination geometry of the aluminum atom can be best described as a distorted trigonal bipyramid. The N1Al1C37C39 atoms are approximately coplanar, sum of the angles at aluminum center being 357.68°. The axial positions are occupied by N2 and O1 atoms, the angle of O(1)–Al(1)–N(2) being 161.86(13)°. This angle is smaller than corresponding ones in enolato aluminum complexes [Al(Me2){OC(But)
CHP(Ph2)
N(8-C9H6N)}] [168.01(14)°] and [Al(Me2){OC(Me)
CHC(Me)
N(8-C9H6N)}] [164.67(8)°],8c and larger than that in bipyridine–phenolate based aluminum complex [AlMe2(BpyPh2,4-But)] [155.87(10)°].7k The Al1–N1 distance of 2.065(2) Å is shorter than that of Al1–N3 [2.183(3) Å], and both are within normal range for a five-coordinate aluminum complex.7k,8c The Al1–O1 distance of 1.813(2) Å is comparable to that in [AlMe2(BpyPh2,4-But)] [1.819(2) Å].7k
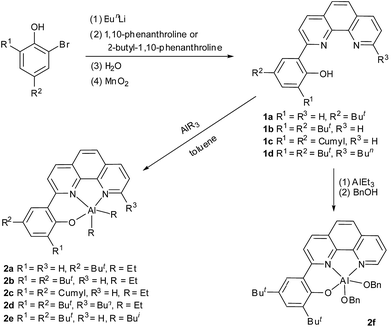 |
| Scheme 1 Synthesis of ligand precursors 1a–1d and complexes 2a–2f. | |
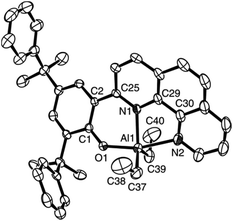 |
| Fig. 1 ORTEP drawing (20% probability) of complex 2c. Selected bond lengths (Å) and angles (deg): Al(1)–O(1) 1.813(2), Al(1)–C(37) 1.936(4), Al(1)–C(39) 1.941(4), Al(1)–N(1) 2.065(2), Al(1)–N(2) 2.183(3), O(1)–C(1) 1.301(3); O(1)–Al(1)–C(37) 95.59(13), O(1)–Al(1)–C(39) 102.08(13), C(37)–Al(1)–C(39) 125.03(18), O(1)–Al(1)–N(1) 86.64(10), C(37)–Al(1)–N(1) 116.42(15), C(39)–Al(1)–N(1) 116.23(14), O(1)–Al(1)–N(2) 161.86(13), C(37)–Al(1)–N(2) 87.96(14), C(39)–Al(1)–N(2) 90.22(14), N(1)–Al(1)–N(2) 75.91(12). | |
The ring-opening polymerization of ε-caprolactone
The ROP of ε-CL in toluene using 2a–2f as the catalysts has been conducted as shown in Table 1. Each of complexes 2a–2e is active for the ROP of ε-CL in the presence of BnOH, and complex 2f is active without requirement of BnOH. In the presence of BnOH, complex 2b exhibits highest activity. It led to 99% monomer conversion of 100 equiv. of ε-CL in 1 h at 50 °C. Complexes 2c and 2e exhibited close catalytic activity to each other, but both are a little lower than complex 2b under the same conditions. Complexes 2a and 2d showed the lowest activity in this series. Complex 2f is more active than 2b. For example, 2f catalyzed 100 equiv. of ε-CL to reach 99% monomer conversion in 1.5 h at 25 °C; whereas 2b drove 100 equiv. of ε-CL to only 89.3% monomer conversion in 1.5 h at 25 °C. An approximate catalytic activity order is 2f > 2b > 2e ≈ 2c > 2d, 2a. Complex 2f also exhibits higher activity than aluminum bipyridine phenolate complex [AlMe2(BpyPh2,4-But)] reported in literature.7k From above activity order of 2a–2f it can be seen that bulky ortho-position substituents on the aromatic rings of phenoxy groups benefit increase of the catalytic activity of the complexes. 2-Position substituent on the phenanthroline ring makes against catalysis of the complex (Table 1, entry 6). The GPC molecular weights of the polymers match the calculated values very well when complexes 2a–2c were employed as the catalysts (Table 1, entries 1–5). However, the GPC molecular weights of the polymers are lower than the calculated values when complexes 2d and 2e were employed as the catalysts (Table 1, entries 6–8). In most cases, the polydispersities are narrow. However, the polydispersities was broadened with increase of polymerization temperatures (Table 1, entries 2–4, 7 and 8).
Table 1 The ROP of ε-CL catalyzed by complexes 2a–2fa
Entry |
Cat. |
[Cat.]0 : [BnOH]0 : [CL]0 |
T (°C) |
Time (h) |
Conv.b (%) |
Mn,calcc (kg mol−1) |
Mn,GPCd (kg mol−1) |
Đe |
Unless otherwise specified, the polymerization reactions were carried out in toluene, [Cat.] = 0.01 M. Measured by 1H NMR spectra. Calculated from the molecular weight of ε-CL times the conversion of monomer and the ratio of [CL]0/[BnOH]0 plus the molecular weight of BnOH. Mn was obtained from GPC which was timed 0.56.14 Đ was obtained from GPC analyses. Mn,calc was calculated from the molecular weight of ε-CL times the conversion of monomer and the ratio of [CL]0/[Cat]0 plus the molecular weight of BnOH. |
1 |
2a |
1 : 1 : 100 |
50 |
5 |
48.1 |
5.6 |
5.2 |
1.14 |
2 |
2b |
1 : 1 : 100 |
70 |
0.5 |
99 |
11.4 |
11.0 |
1.31 |
3 |
2b |
1 : 1 : 100 |
50 |
1 |
99 |
11.4 |
11.0 |
1.21 |
4 |
2b |
1 : 1 : 100 |
25 |
1.5 |
89.3 |
10.3 |
8.6 |
1.08 |
5 |
2c |
1 : 1 : 100 |
50 |
2 |
99 |
11.4 |
9.6 |
1.19 |
6 |
2d |
1 : 1 : 100 |
50 |
8 |
93.2 |
10.7 |
6.1 |
1.13 |
7 |
2e |
1 : 1 : 100 |
25 |
3 |
36.3 |
4.2 |
3.2 |
1.07 |
8 |
2e |
1 : 1 : 100 |
50 |
1.5 |
96.3 |
11.1 |
7.9 |
1.10 |
9f |
2f |
1 : 0 : 100 |
25 |
1.5 |
99 |
11.4 |
8.6 |
1.12 |
Kinetic study of ε-CL polymerization catalyzed by complex 2f was performed. Plots of ln([CL]0/[CL]) versus time revealed a linear relationship (Fig. 2), which indicates that the polymerization proceeds with first-order dependence on monomer concentration. This implies that the polymerization catalyzed by 2f is well controlled. The linear relationship of molecular weights of the polymers versus ε-CL conversion along with narrow PDI is another characteristic of living polymerization (Fig. 3). The corresponding SEC traces (Fig. 4) of the obtained polymers showed monomodal peaks with narrow PDI (1.07–1.08). The end group analysis of the PCL proved that the polymer was capped with a benzoxy group (Hb) and a hydroxymethyl group (Hg) (Fig. 5). All these facts imply that the polymerization initiated through insertion of ε-CL into an Al–OCH2Ph bond followed by ring opening via acyl-oxygen cleavage.
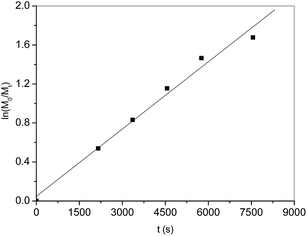 |
| Fig. 2 Plot of ln([CL]0/[CL]t) versus time for the polymerization of ε-CL catalyzed by 2f. Conditions: [Cat.]0 : [BnOH]0 : [CL]0 = 1 : 0 : 200; [Cat.] = 0.01 M; solvent: toluene; temperature: 25 °C. | |
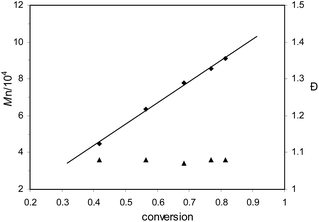 |
| Fig. 3 Plots of PCL Mn (♦, obtained from GPC analysis) and dispersity (▲: Mw/Mn) as a function of ε-CL conversion using complex 2f as the catalyst. Conditions: [2f]0 : [BnOH]0 : [CL]0 = 1 : 0 : 200; [2f]0 = 0.01 M; solvent: toluene; temperature: 25 °C. | |
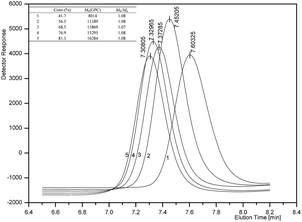 |
| Fig. 4 The GPC curves of PCL depending different conversion catalyzed by 2f. Conditions: [Cat.]0 : [BnOH]0 : [CL]0 = 1 : 0 : 200; [Cat.] = 0.01 M; solvent: toluene; temperature: 25 °C. | |
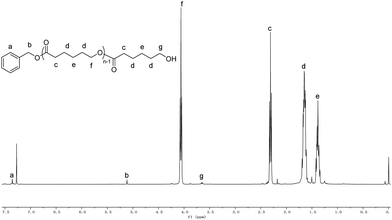 |
| Fig. 5 1H NMR spectrum of PCL initiated by 2b/BnOH (Table 1, entry 3). | |
The ring-opening polymerization of rac-lactide
Catalysis of complexes 2a–2f toward the ROP of rac-LA was evaluated, and the results showed that each of these complexes can catalyze the polymerization of rac-LA at elevated temperature (Table 2). Both 2a and 2b exhibited close activity in catalyzing polymerization of 100 equiv. of rac-LA in toluene at 70 °C in the presence of BnOH, leading to higher than 97% monomer conversion in 24 h (Table 2, entries 1 and 2). Complexes 2c and 2d revealed much lower catalytic activity than 2a and 2b under the same conditions. 89.9% conversion of 100 equiv. of rac-LA was achieved in 72 h when 2c/BnOH was employed as catalyst and 60.9% of 100 equiv. of the monomer was concerted in 72 h when 2d/BnOH was employed as catalyst (Table 2, entries 3 and 4). Complex 2e showed a little lower activity than 2a and 2b (Table 2, entry 5). The approximate activity order is 2a ≈ 2b > 2e > 2c > 2d. This activity order is different from that in catalyzing ε-CL polymerization. A bulky ortho-position substituent on the aromatic ring of phenoxy group is no longer necessary to increase the catalytic activity, and even unfavorable. However, the 2-position substituent on the phenanthroline ring is still unfavored as that shown in catalyzing ε-CL polymerization. Catalysis of 2f did not require presence of extra BnOH. Its catalytic activity was close to that of 2b under comparable conditions. It also drove polymerization of 200 equiv. of rac-LA in good activity, 88.5% monomer conversion being achieved in 25 h (Table 2, entries 7 and 8). At lower temperature than 70 °C, the catalytic activity of the complexes was markedly reduced. For example, 2e/BnOH led to only 13.5% conversion of 100 equiv. of rac-LA at 50 °C in 96 h (Table 2, entry 6); 2f drove 97.1% conversion of 100 equiv. of rac-LA at 50 °C in 72 h (Table 2, entry 9). Compared with aluminum bipyridine phenolate complexes, complexes 2a, 2b and 2f exhibit slightly higher activity than [AlMe2(BpyPh2,4-But)], but have lower activity than [AlMe2(BpyPh4-But)] and [AlMe2(BpyPh2,4-Me)].7k The GPC analysis revealed that molecular weight of the polymer catalyzed by 2a/BnOH matched the calculated value very well, but the PDI value is relatively wide (Table 2, entry 1). The GPC molecular weights of the polymers catalyzed by 2b–2e/BnOH and 2f were lower than the calculated values (Table 2, entries 2–9). A plausible reason could be the presence of side reactions such as transesterification and chain transfer in the process of polymerization. Catalysis of 2b was further evaluated with different BnOH or monomer loadings. The polymerization rate is almost same in the presence of 2 equiv. of BnOH using 2b as catalyst (Table 2, entry 10). In the absence of benzyl alcohol the reaction catalyzed by 2b resulted in very viscous species at 70 °C in 14 h. The 1H NMR analysis indicated 84.2% monomer conversion and the GPC determination showed higher molecular weight of the final polymer than the expected value (Table 2, entry 11). This is probably caused by trace amount of water or other impurities in the reaction system. Higher or lower than 100 equiv. of rac-LA loadings gave high monomer conversion at 70 °C in 24 h when catalyzed by 2b/BnOH (Table 2, entries 12–14). A linear relationship between the number average molecular weights and ([LA]0 − [LA])/[BnOH]0 is observed (Fig. 6). This fact, combined with the narrow PDI values, imply the “living” character of the polymerization process and the possibility of the presence of multiple active species at high temperature, such as LAl(Et)OBn and LAl(OBn)2. Meanwhile, side reactions such as transesterification and chain transfer are also present from the MALDI-TOF MS of PLA (Fig. S1, ESI†).
Table 2 The ROP of rac-lactide catalyzed by complexes 2a–2fa
Entry |
Cat. |
[Cat.]0 : [BnOH]0 : [LA]0 |
Time (h) |
Conv.b (%) |
Mn,calcc (kg mol−1) |
Mn,GPCd (kg mol−1) |
Đe |
Pmf |
Unless otherwise specified, the polymerization reactions were carried out at 70 °C in toluene, [Cat.] = 0.01 M. Measured by 1H NMR spectra. Calculated from the molecular weight of rac-LA times the conversion of monomer and the ratio of [LA]0/[BnOH]0 plus the molecular weight of BnOH. Mn was obtained from GPC which was timed 0.58.14 Đ was obtained from GPC analyses. Pm is the probability of meso linkages between monomer units determined from the methine region of the homonuclear decoupled 1H NMR spectroscopy at 25 °C in CDCl3 (Fig. S2, ESI). The polymerization reactions were carried out at 50 °C. Mn,calc was calculated from the molecular weight of rac-LA times the conversion of monomer and the ratio of [LA]0/[Cat]0 plus the molecular weight of BnOH. |
1 |
2a |
1 : 1 : 100 |
24 |
97.4 |
14.1 |
13.6 |
1.46 |
0.48 |
2 |
2b |
1 : 1 : 100 |
24 |
97.1 |
14.1 |
7.6 |
1.19 |
0.74 |
3 |
2c |
1 : 1 : 100 |
72 |
89.9 |
13.1 |
5.1 |
1.11 |
0.62 |
4 |
2d |
1 : 1 : 100 |
72 |
60.9 |
8.9 |
6.2 |
1.06 |
0.47 |
5 |
2e |
1 : 1 : 100 |
24 |
90 |
13.1 |
6.9 |
1.10 |
0.76 |
6g |
2e |
1 : 1 : 100 |
96 |
13.5 |
|
|
|
|
7 |
2f |
1 : 0 : 100 |
24 |
94.5 |
13.7h |
6.6 |
1.13 |
0.75 |
8 |
2f |
1 : 0 : 200 |
25 |
88.5 |
25.6h |
17.9 |
1.11 |
0.76 |
9g |
2f |
1 : 0 : 100 |
72 |
97.1 |
14.1h |
7.2 |
1.11 |
0.78 |
10 |
2b |
1 : 2 : 100 |
24 |
96.2 |
7.0 |
4.7 |
1.17 |
0.74 |
11 |
2b |
1 : 0 : 100 |
14 |
84.2 |
|
20.9 |
1.24 |
0.75 |
12 |
2b |
1 : 1 : 50 |
23 |
93.8 |
6.9 |
4.4 |
1.15 |
0.72 |
13 |
2b |
1 : 1 : 150 |
24 |
94.9 |
20.6 |
12.9 |
1.24 |
0.76 |
14 |
2b |
1 : 1 : 200 |
24 |
98.7 |
28.6 |
16.0 |
1.21 |
0.77 |
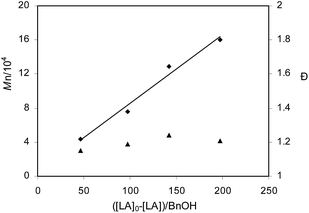 |
| Fig. 6 Plots of Mn (♦, obtained from GPC analysis) and dispersity (▲, Mw/Mn) as a function of ([LA]0 − [LA])/BnOH] for the polymerization of rac-LA catalyzed by 2b/BnOH at 70 °C. Conditions: [Cat.]0 = 0.01 M; solvent: toluene. | |
Homonuclear decoupled 1H NMR spectra in the methine regions of the PLA showed that isotactic enriched PLA were obtained when complexes 2b, 2c, 2e and 2f were employed as the catalysts. The Pm value range from 0.62 to 0.78. Complex 2c led to lower iso-selectivity and complexes 2b and 2f showed higher iso-selectivity. The iso-selectivity could be further enhanced by lowering the reaction temperature (Table 2, entry 9). Complexes 2a and 2d exhibited poor selectivity. They showed slightly heterotactic bias. These results proved that a bulky ortho-position substituent on the aromatic ring of phenoxy group is important to increase the selectivity, but too bulky substituent like cumyl group is disadvantageous to enhancement of the selectivity. That is to say, the steric hindrance of the substituent is too large or too small to be disadvantageous for the selectivity of the polymerization. This is inconsistent with previous studies that the enhancement of stereoselectivity requires bulky substituent.15 The presence of 2-position substituent on the phenanthroline ring is also disadvantageous to improve the selectivity. In addition, the catalytic selectivity of complexes 2b and 2f is also higher than that of aluminum bipyridine phenolate complex [AlMe2(BpyPh2,4-But)], which led to polymer with a Pm value of 0.70 at 70 °C.
In order to better understand the polymerization process, the kinetics of rac-LA polymerization with complex 2f as the catalyst was studied. The plots of ln([LA]0/[LA]t) versus reaction time at different catalyst concentrations are shown in Fig. 7. The plots revealed linear relationship in each case, indicating the first order dependence of the polymerization on monomer concentration. The linear increase of Kapp with complex 2f concentration (Fig. 8) also indicates the order to be first-order. Therefore, the overall rate law in the form of −d[LA]/dt = kp[LA][2f] (kp = 10.3 dm3 mol−1 h−1) was established. That is, the rate determining step involves one metal center and one monomer molecule stoichiometrically.
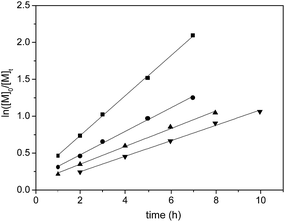 |
| Fig. 7 Plot of ln([LA]0/[LA]t) versus time for the polymerization of rac-LA catalyzed by 2f in toluene at 70 °C, [LA]0 = 0.5 M. (■) [LA]0/[Al]0 = 20, Kapp = 0.26936 ± 0.0041 h−1; (●) [LA]0/[Al]0 = 30, Kapp = 0.15847 ± 0.00445 h−1; (▲) [LA]0/[Al]0 = 40, Kapp = 0.11997 ± 0.00376 h−1; (▼) [LA]0/[Al]0 = 60, Kapp = 0.10436 ± 0.0034 h−1. | |
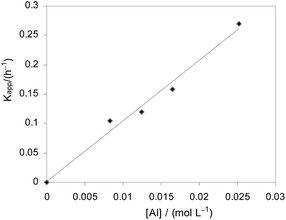 |
| Fig. 8 Kapp versus the concentration of 2f for the rac-LA polymerization at 70 °C in toluene ([LA]0 = 0.5 M, kp = 10.3 dm3 mol−1 h−1). | |
The ring-opening polymerization of rac-β-butyrolactone
Complexes 2a–2d and 2f were also assessed in the ring opening polymerization of rac-β-BL. Polymerizations were carried out in toluene solution at 70 °C (Table 3). Both 2b/BnOH and 2f showed good catalytic activity towards the ROP of rac-β-BL. They drove polymerization of 100 equiv. of monomer in higher than 90% conversions in 12 h. Complex 2d exhibited the lowest activity in catalyzing the ROP. 2.9% conversion be achieved in 24 at the same monomer loading and reaction temperature as above. The molecular weights determined by GPC are markedly lower than the calculated values. This is ascribed to the presence of transesterification and chain transfer side reactions, which is further confirmed by MALDI-TOF MS analysis of PHB (Fig. S3, ESI†). It seems that the controllability showed by these complexes is lower than those found in the ROP of ε-CL and rac-LA. The polymer was atactic, as determined by the 13C NMR analysis in the carbonyl and methylene regions (Fig. S4, ESI†).
Table 3 The ROP of β-butyrolactone catalyzed by complexes 2a–2d and 2fa
Entry |
Cat. |
[Cat.]0 : [BnOH]0 : [β-BL]0 |
Time (h) |
Conv.b (%) |
Mn,calcc (kg mol−1) |
Mn,GPCd (kg mol−1) |
Đe |
Unless otherwise specified, the polymerization reactions were carried out at 70 °C in toluene, [Cat.] = 0.01 M. Measured by 1H NMR spectra. Calculated from the molecular weight of rac-BL times the conversion of monomer and the ratio of [BL]0/[BnOH]0 plus the molecular weight of BnOH. Mn was obtained from GPC which was timed 0.54.14 Đ was obtained from GPC analysis. Mn,calc was calculated from the molecular weight of rac-BL times the conversion of monomer and the ratio of [BL]0/[Cat]0 plus the molecular weight of BnOH. |
1 |
2a |
1 : 1 : 100 |
12 |
37.9 |
3.4 |
2.2 |
1.10 |
2 |
2b |
1 : 1 : 100 |
5 |
41.9 |
3.7 |
1.2 |
1.23 |
3 |
2b |
1 : 1 : 100 |
12 |
94.4 |
8.3 |
3.5 |
1.14 |
4 |
2c |
1 : 1 : 100 |
24 |
72.1 |
6.3 |
2.0 |
1.14 |
5 |
2d |
1 : 1 : 100 |
24 |
2.9 |
|
|
|
6 |
2f |
1 : 0 : 100 |
12 |
93.5 |
8.1f |
2.3 |
1.11 |
Block copolymerization
The synthesis of block copolymers was attempted by using catalyst 2f in toluene at different temperature (Table 4). For instance, the polymerization of 100 equiv. of ε-CL in toluene catalyzed by 2f at 25 °C went to completion in 1.5 hour. Then 100 equiv. of rac-LA were added to the reaction system and the reaction was run for further 24 hours at 70 °C. 1H NMR spectrum of the resulting mixture showed ε-CL and rac-LA were almost completely converted (Table 4, entry 2). The sequential addition of the two monomers led to the achievement of a PCL-b-PLA copolymer, which represents an experimental evidence of the living behavior of the polymerization promoted by this class of initiators. Other block copolymers can be synthesized in a similar manner (Fig. S5 and S6, ESI†). In order to obtain the PHB-b-PLA copolymer, rac-LA had to be added to living PHB chains. The opposite sequence of monomers addition led mainly to PLA and a low amount of the block copolymer (Table 4, entry 3). The importance of the order of the monomer addition in the block copolymerization was previously underlined in literature.16
Table 4 Formation of block copolymer catalyzed by complex 2fa
Entry |
[Cat.]0 : [M1]0 : [M2]0 |
Time (h) |
T (°C) |
Conv. b (M1) % |
Conv. b (M2) % |
Mn,GPCc (kg mol−1) |
Đc |
The polymerization reactions were carried out in toluene, [Cat.] = 0.01 M. [Cat.]0 : [M1]0 : [M2]0 = 1 : 100 : 100. Measured by 1H NMR spectra. Mn and Đ of copolymer was obtained from GPC analyses, GPC Mn without correction. |
1 |
2f : CL : BL |
1.5 + 12 |
25 + 70 |
99 |
82 |
15.0 |
1.24 |
2 |
2f : CL : LA |
1.5 + 24 |
25 + 70 |
99 |
99 |
21.0 |
1.16 |
3 |
2f : LA : BL |
24 + 12 |
70 |
93 |
11 |
13.2 |
1.18 |
4 |
2f : BL : LA |
12 + 24 |
70 |
98 |
84 |
|
|
In order to confirm formation of the copolymers, we monitored the polymerization process of ε-CL and rac-LA catalyzed by 2f and charactered the polymers. When 100 equiv. of ε-CL monomer conversion approached to 99%, a 1/8 volume of sample was taken from the polymerization system for testing and showed its GPC Mn = 11
174. Then 100 equiv. of rac-LA was added into the reaction system to generate the block copolymer PCL-b-PLA with GPC Mn = 17
187 at 84.7% rac-LA conversion (Fig. S7, ESI†). 1H NMR and 13C NMR spectra of the PCL-b-PLA displayed all the characteristic signals, including the main bodies of the two blocks, BnO end and the monomer ends (Fig. 9 and 10). The 13C NMR spectra show the connecting portions of diblock copolymers of PCL-b-PLA, which proved the formation of block copolymers.17 The 2D DOSY NMR spectrum of the copolymer provided further evidence for the copolymerization (Fig. S8, ESI†). The spectrum showed that the multiplets of the poly(ε-caprolactone) block (centered at 4.05, 2.30 and 1.65 ppm) and the multiplets of the poly(rac-lactide) block (centered at 5.15 and 1.57 ppm) lied at the same diffusion coefficient, and therefore belonged to the same polymeric chains.16a,18 The 1H NMR spectrum of the polymer (Fig. 9) indicated polymer chains end-capped with a benzoxy group and a hydroxyl group. End group analysis of PCL-b-PHB copolymer by 1H NMR spectroscopy also showed the polymer chains end-capped with a benzoxy group and a hydroxyl group (Fig. S5, ESI†).
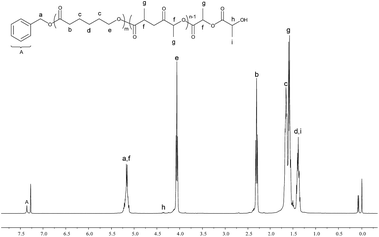 |
| Fig. 9 1H NMR spectrum (CDCl3, 400 MHz, 25 °C) of the PCL-b-PLA copolymer. | |
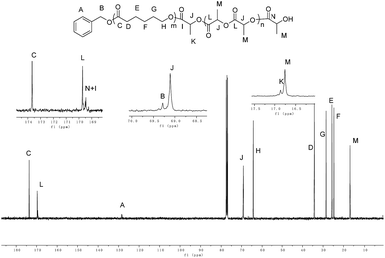 |
| Fig. 10 13C NMR spectrum (CDCl3, 101 MHz, 25 °C) of the PCL-b-PLA copolymer. | |
Conclusions
We have synthesized and characterized a series of aluminum complexes supported by 2-(1,10-phenanthrolin-2-yl)phenolate ligands. In the presence of BnOH, the complexes efficiently catalyzed the ROP of ε-CL, rac-LA and rac-β-BL. The catalytic activity of the complexes is affected by the substituents on the ligands. In each case the 2-position substituent on the phenanthroline ring of the ligand is unfavored. The pluses and minuses of the bulky ortho-position substituent on the aromatic ring of phenoxy group depend on reaction substrates that they catalyzed, but too bulky substituent is often unfavorable. The polymerization reaction led to PCL with good molecular weight control and narrow molecular weight distribution. Isotactic enriched PLA were obtained when 2b, 2c, 2e and 2f were used as the catalysts, while atactic poly(hydroxybutyrate) was got no matter which complex was employed. Kinetic analysis using 2f as the catalyst revealed that the polymerization of ε-CL was first-order dependence on monomer concentration, and the polymerization of rac-LA proceeded with first-order dependence on both monomer and catalyst concentration. The block polymerization were also performed utilizing the “living” character of the aluminum catalysts, forming block copolymers including PCL-b-PHB, PCL-b-PLA, and PHB-b-PLA.
Experimental
All air or moisture sensitive manipulations were performed under dry N2 using standard Schlenk techniques. Solvents were distilled under N2 over sodium/benzophenone (n-hexane and diethyl ether) or sodium (toluene) and degassed prior to use. AlEt3 was purchased from Acros Organics and used as received. C6D6 and CDCl3 were purchased from Cambridge Isotope Laboratories, Inc., and were degassed and stored over Na/K alloy (C6D6) or 4 Å molecular sieves (CDCl3). ε-CL and rac-β-BL were stirred over CaH2 for 24 h and distilled under vacuum. rac-LA was recrystallized three times from toluene prior to use. o-Bromophenols and 2-butyl-1,10-phenanthroline were prepared according to the reported methods.19 Other chemicals and solvents were purchased from commercial venders. NMR spectra were recorded on a Bruker av400 spectrometer at ambient temperature. The chemical shifts of 1H and 13C NMR spectra were referenced to TMS or internal solvent resonances. Elemental analyses were performed on an Elementar Vario EL cube analyzer. Gel permeation chromatography (GPC) measurements were performed on a Waters 150C instrument equipped with UltraStyragel columns (103, 104, and 105 Å) and a 410 refractive index detector, using monodispersed polystyrene as the calibration standard. THF (HPLC grade) was used as an eluent at a flow rate of 1 cm3 min−1.
Synthesis of 1a
To a stirred solution of 2-bromo-4-(tert-butyl)phenol (1.37 g, 6.0 mmol) in Et2O (20 cm3), BunLi (2.5 M solution in hexane, 5.3 cm3, 13.25 mmol) was added dropwise at −78 °C. After stirring for 2 h at room temperature, the resulting mixture was added dropwise to 1,10-phenanthroline (0.90 g, 5.0 mmol) in toluene (30 cm3). The dark purple solution was stirred at the same temperature for additional 20 h. The reaction mixture was quenched with water (20 cm3) and extracted with ethyl acetate. The combined organic layers were dried over Na2SO4 and then removed solvent by rotary evaporation. The residue was used for the next step without further purification. To a stirred solution of the crude product in ethyl acetate (20 cm3), MnO2 (8.7 g, 100 mmol) was added at room temperature. The black suspension was stirred at 60 °C for 12 h. The resulting mixture was dried over MgSO4 and filtered through a celite pad to remove MnO2. The organic layers were evaporated and purified by column chromatography (eluting with hexane/ethyl acetate = 2
:
1) to afford 1a as a yellow solid in overall 26.8% yield (0.44 g, 1.34 mmol). Anal. calcd for C22H20N2O·0.1C4H8O2: C, 79.78; H, 6.22; N, 8.31%. Found: C, 79.95 H, 6.29; N, 8.22%. 1H NMR (400 MHz, CDCl3): δ 16.13 (s, 1H, OH), 9.16 (d, J = 4.2 Hz, 1H, Ar), 8.30 (d, J = 8.7 Hz, 1H, Ar), 8.24–8.17 (m, 2H, Ar), 7.96 (d, J = 2.2 Hz, 1H, Ar), 7.73 (s, 2H, Ar), 7.60 (dd, J = 8.0, 4.3 Hz, 1H, Ar), 7.45 (dd, J = 8.6, 2.3 Hz, 1H, Ar), 7.14 (d, J = 8.6 Hz, 1H, Ar), 1.41 (s, 9H, But). 13C NMR (101 MHz, CDCl3): δ 159.49, 157.70, 150.74, 144.80, 142.87, 140.80, 137.25, 135.86, 129.70, 128.98, 126.39, 126.31, 125.75, 123.41, 122.68, 118.83, 118.36, 117.93, 34.33, 31.74.
Synthesis of 1b
Compound 1b was synthesized using the same procedure as for 1a. Thus, 2-bromo-4,6-di-tert-butylphenol (1.42 g, 5 mmol) was treated successively with BunLi (4.4 cm3, 2.5 M solution in hexane, 11 mmol) and 1,10-phenanthroline (0.90 g, 5.0 mmol). The resulting species was oxidized with MnO2 to afford, after purified by column chromatography (eluting with hexane/ethyl acetate = 5
:
1), 1b as an orange solid in overall 41.3% yield (0.794 g, 2.06 mmol). Anal. calcd for C26H28N2O·0.15C4H8O2: C, 80.33; H, 7.40; N, 7.04%. Found: C, 80.40 H, 7.57; N, 7.02%. 1H NMR (400 MHz, CDCl3): δ 16.11 (s, 1H, OH), 9.21 (dd, J = 4.3, 1.6 Hz, 1H, Ar), 8.32–8.18 (m, 3H, Ar), 7.85 (d, J = 2.3 Hz, 1H, Ar), 7.74 (d, J = 2.4 Hz, 2H, Ar), 7.61 (dd, J = 8.1, 4.3 Hz, 1H, Ar), 7.49 (d, J = 2.1 Hz, 1H, Ar), 1.60 (s, 9H, But), 1.41 (s, 9H, But). 13C NMR (101 MHz, CDCl3): δ 158.82, 158.69, 150.77, 144.91, 142.77, 139.35, 138.22, 137.16, 135.98, 129.02, 127.03, 126.27, 126.17, 125.87, 123.29, 121.12, 119.39, 117.87, 35.63, 34.52, 31.81, 29.94.
Synthesis of 1c
Compound 1c was synthesized using the same procedure as for 1a. Thus, 2-bromo-4,6-bis(α,α-dimethylbenzyl) phenol (2.45 g, 6 mmol) was treated successively with BunLi (5.3 cm3, 13.25 mmol) and 1,10-phenanthroline (0.90 g, 5.0 mmol). The resulting species was oxidized with MnO2 to afford, after purified by column chromatography (eluting with hexane/ethyl acetate = 2
:
1), 1c as a light yellow solid in overall 10.3% yield (0.21 g, 0.52 mmol). Anal. calcd for C36H32N2O: C, 85.01; H, 6.34; N, 5.51%. Found: C, 85.03 H, 6.48; N, 5.43. 1H NMR (400 MHz, CDCl3): δ 15.40 (s, 1H, OH), 9.01 (d, J = 3.0 Hz, 1H, Ar), 8.10 (d, J = 8.7 Hz, 1H, Ar), 8.06 (d, J = 7.9 Hz, 1H, Ar), 7.97 (d, J = 8.7 Hz, 1H, Ar), 7.73 (s, 1H, Ar), 7.57 (s, 2H, Ar), 7.48 (dd, J = 7.9, 4.3 Hz, 1H, Ar), 7.40 (s, 1H, Ar), 7.38–7.29 (m, 6H, Ar), 7.29–7.19 (m, 3H, Ar), 7.12 (t, J = 7.0 Hz, 1H, Ar), 1.83 (s, 6H, Me), 1.80 (s, 6H, Me). 13C NMR (101 MHz, CDCl3): δ 158.27, 157.93, 151.35, 151.26, 150.45, 144.53, 142.60, 138.89, 137.49, 137.10, 135.89, 129.23, 128.85, 128.13, 127.85, 126.96, 126.24, 126.16, 126.08, 125.78, 125.70, 125.05, 123.38, 123.13, 119.27, 118.38, 42.81, 31.20, 29.86.
Synthesis of 1d
Compound 1d was synthesized using the same procedure as for 1a. Thus, 2-bromo-4,6-di-tert-butylphenol (1.59 g, 5.6 mmol) was treated successively with BunLi (4.9 cm3, 12.32 mmol) and 2-butyl-1,10-phenanthroline (1.1 g, 4.6 mmol). The resulting species was oxidized with MnO2 to afford, after purified by column chromatography (eluting with hexane/ethyl acetate = 2
:
1) to afford 1d as an orange solid in overall 42% yield (0.86 g, 1.93 mmol). Anal. calcd for C30H36N2O·0.06C4H8O2: C, 81.45; H, 8.25; N, 6.28%. Found: C, 81.39 H, 8.03; N, 6.32. 1H NMR (400 MHz, CDCl3): δ 16.51 (s, 1H, OH), 8.28 (d, J = 8.7 Hz, 1H, Ar), 8.22 (d, J = 8.8 Hz, 1H, Ar), 8.10 (d, J = 8.2 Hz, 1H, Ar), 7.86 (d, J = 2.3 Hz, 1H, Ar), 7.74–7.67 (m, 2H, Ar), 7.50–7.45 (m, 2H, Ar), 3.17 (t, J = 7.8 Hz, 2H, CH2), 2.24–2.15 (m, 2H, CH2), 1.61–1.51 (m, 2H, CH2), 1.60 (s, 9H, But), 1.41 (s, 9H, But), 1.08 (t, J = 7.4 Hz, 3H, Me). 13C NMR (101 MHz, CDCl3): δ 163.26, 159.07, 158.48, 144.14, 142.50, 139.06, 138.30, 137.00, 135.87, 127.07, 126.83, 126.19, 125.93, 124.68, 123.67, 120.86, 118.81, 117.73, 38.74, 35.67, 34.52, 31.83, 30.41, 29.86, 22.90, 14.31.
Synthesis of 2a
A solution of 1a (0.328 g, 1.0 mmol) in toluene (15 cm3) was cooled to 0 °C. AlEt3 (1.83 cm3, 0.6 M solution in heptane, 1.1 mmol) was added dropwise to the cooled solution. The color of the solution instantly changed from light yellow to deep red. The resultant mixture was warmed to room temperature and stirred for 12 h. The solution was filtered and the filtrate was concentrated to afford deep red crystalline solid of 2a (0.198 g, 48%). Anal. calcd for C26H29AlN2O: C, 75.70; H, 7.09; N, 6.79%. Found: C, 75.43 H, 6.84; N, 6.59. 1H NMR (400 MHz, C6D6): δ 8.57 (dd, J = 4.7, 1.4 Hz, 1H, Ar), 7.64 (d, J = 8.9 Hz, 1H, Ar), 7.60 (d, J = 2.4 Hz, 1H, Ar), 7.48 (d, J = 8.8 Hz, 1H, Ar), 7.39 (dd, J = 8.8, 2.5 Hz, 1H, Ar), 7.35 (d, J = 8.8 Hz, 1H, Ar), 7.30 (dd, J = 8.2, 1.3 Hz, 1H, Ar), 6.99–6.94 (m, 2H, Ar), 6.73 (dd, J = 8.1, 4.7 Hz, 1H, Ar), 1.54 (t, J = 8.0 Hz, 6H, Me), 1.32 (s, 9H, But), 0.75–0.64 (m, 2H, Al–CH2), 0.53–0.43 (m, 2H, Al–CH2). 13C NMR (101 MHz, C6D6): δ 165.23, 161.07, 146.34, 140.91, 140.03, 138.22, 138.12, 136.78, 132.71, 127.98, 126.14, 125.55, 124.56, 124.30, 124.01, 123.28, 119.39, 34.16, 31.75, 11.96, 6.98.
Synthesis of 2b
A solution of 1b (0.23 g, 0.60 mmol) in toluene (15 cm3) was cooled to 0 °C. AlEt3 (1.1 cm3, 0.6 M solution in heptane, 0.66 mmol) was added to the cooled solution. The resultant mixture was warmed to room temperature and stirred for 12 h. The solution was filtered and the filtrate was concentrated to afford deep red crystalline solid of 2b (0.28 g, 81%). Anal. calcd for C30H37AlN2O: C, 76.89; H, 7.96; N, 5.98%. Found: C, 76.78 H, 7.94; N, 6.08. 1H NMR (400 MHz, C6D6): δ 8.60 (d, J = 4.7 Hz, 1H, Ar), 7.84 (d, J = 2.4 Hz, 1H, Ar), 7.63–7.56 (m, 1H, Ar), 7.54 (s, 1H, Ar), 7.29 (d, J = 8.8 Hz, 2H, Ar), 6.98–6.90 (m, 2H, Ar), 6.76–6.69 (m, 1H, Ar), 1.99 (s, 9H, But), 1.43 (s, 9H, But), 1.39 (t, J = 8.1 Hz, 6H, Me), 0.78–0.67 (m, 2H, Al–CH2), 0.54–0.43 (m, 2H, Al–CH2). 13C NMR (101 MHz, C6D6): δ 164.31, 161.70, 146.49, 141.78, 140.67, 140.23, 137.84, 137.42, 136.70, 129.18, 127.91, 126.14, 125.31, 124.60, 124.14, 123.92, 123.16, 119.88, 36.18, 34.49, 31.92, 30.40, 11.82, 6.97, 6.52.
Synthesis of 2c
1c (0.182 g, 0.36 mmol) was dissolved in toluene (10 cm3) and cooled to 0 °C. To the solution was added AlEt3 (0.72 cm3, 0.6 M solution in heptane, 0.43 mmol). The resultant mixture was warmed to room temperature and stirred overnight. The solution was filtered and the filtrate was concentrated to afford deep red crystalline solid of 2c (0.16 g, 76.4%). Anal. calcd for C40H41AlN2O·C7H8: C, 82.42; H, 7.21; N, 4.09%. Found: C, 82.25 H, 6.98; N, 4.25. 1H NMR (400 MHz, C6D6): δ 8.56 (dd, J = 4.8, 1.4 Hz, 1H, Ar), 7.77 (d, J = 2.4 Hz, 1H, Ar), 7.72–7.67 (m, 2H, Ar), 7.50 (d, J = 2.4 Hz, 1H, Ar), 7.49–7.42 (m, 3H, Ar), 7.36 (t, J = 7.8 Hz, 2H, Ar), 7.29–7.08 (m, 7H, Ar), 7.07–7.00 (m, 3H, Ar), 6.87 (s, 2H, Ar), 6.65 (dd, J = 8.1, 4.8 Hz, 1H, Ar), 2.12 (s, 6H, Me), 2.11 (s, toluene), 1.78 (s, 6H, Me), 1.12 (t, J = 8.1 Hz, 6H, Me), 0.43–0.33 (m, 2H, CH2), 0.24–0.14 (m, 2H, CH2). 13C NMR (101 MHz, C6D6): δ 163.96, 161.19, 151.91, 151.84, 146.35, 141.72, 140.66, 140.22, 137.89, 137.58, 136.83, 136.59, 131.35, 129.33, 128.57, 128.45, 128.19, 127.94, 127.37, 126.62, 126.02, 125.98, 125.70, 125.58, 125.25, 125.20, 124.37, 124.11, 123.89, 119.72, 43.22, 43.00, 31.41, 30.08, 11.43, 6.16.
Synthesis of 2d
A solution of 1d (0.47 g, 1.07 mmol) in toluene (15 cm3) was cooled to 0 °C. AlEt3 (2.0 cm3, 0.6 M solution in heptane, 1.2 mmol) was added to the cooled solution. The resultant mixture was warmed to room temperature and stirred overnight. The solution was filtered and the filtrate was concentrated to afford red crystalline solid of 2d (0.26 g, 46.3%). Anal. calcd for C34H45AlN2O: C, 77.83; H, 8.64; N, 5.34%. Found: C, 77.77 H, 8.70; N, 5.21. 1H NMR (400 MHz, C6D6): δ 7.84 (d, J = 2.5 Hz, 1H, Ar), 7.58 (d, J = 8.9 Hz, 1H, Ar), 7.53 (d, J = 2.4 Hz, 1H, Ar), 7.41 (d, J = 8.4 Hz, 1H, Ar), 7.33 (d, J = 8.8 Hz, 1H, Ar), 7.07 (d, J = 8.7 Hz, 1H, Ar), 7.00 (d, J = 8.7 Hz, 1H, Ar), 6.94 (d, J = 8.4 Hz, 1H, Ar), 3.35–3.27 (m, 2H, CH2), 1.97 (s, 9H, But), 1.67–1.57 (m, 2H, CH2), 1.44 (s, 9H, But), 1.43–1.34 (m, 8H, CH2 + Me), 0.90 (t, J = 7.3 Hz, 3H, Me), 0.81–0.68 (m, 2H, CH2), 0.52–0.43 (m, 2H, CH2). 13C NMR (101 MHz, C6D6): δ 164.06, 162.53, 161.59, 141.30, 141.05, 140.18, 138.24, 137.58, 136.75, 128.97, 126.76, 125.70, 125.17, 125.07, 124.86, 124.61, 123.44, 120.78, 36.70, 36.18, 34.50, 32.37, 31.96, 30.35, 23.33, 14.22, 11.71, 6.50.
Synthesis of 2e
A solution of 1b (0.23 g, 0.6 mmol) in toluene (15 cm3) was cooled to 0 °C. A solution of AlBu3i (0.65 cm3, 1.1 M solution in hexane, 0.72 mmol) was added to the cooled solution. The resultant mixture was warmed to room temperature and stirred overnight. The solution was filtered and the filtrate was concentrated to afford red crystalline solid of 2e (0.198 g, 62.9%). Anal. calcd for C34H45AlN2O: C, 77.83; H, 8.64; N, 5.34%. Found: C, 77.57 H, 8.95; N, 5.21. 1H NMR (400 MHz, C6D6): δ 8.71 (dd, J = 4.7, 1.4 Hz, 1H, Ar), 7.82 (d, J = 2.5 Hz, 1H, Ar), 7.66 (d, J = 8.9 Hz, 1H, Ar), 7.56 (d, J = 2.3 Hz, 1H, Ar), 7.29 (t, J = 8.8 Hz, 2H, Ar), 6.98–6.92 (m, 2H, Ar), 6.77 (dd, J = 8.1, 4.7 Hz, 1H, Ar), 2.13–2.01 (m, 2H, CH), 1.97 (s, 9H, But), 1.42 (s, 9H, But), 1.13 (d, J = 6.5 Hz, 6H, Me), 1.02 (d, J = 6.5 Hz, 6H, Me), 0.75 (dd, J = 13.2, 7.5 Hz, 2H, CH2), 0.48 (dd, J = 13.2, 6.5 Hz, 2H, CH2). 13C NMR (101 MHz, C6D6): δ 164.15, 162.01, 146.68, 141.72, 140.67, 140.19, 137.90, 137.42, 136.72, 129.34, 127.94, 126.13, 125.48, 124.83, 124.29, 123.94, 123.26, 119.75, 36.12, 34.49, 31.89, 30.50, 29.46, 29.27, 29.01, 27.63, 24.87.
Synthesis of 2f
A solution of 1b (0.23 g, 0.60 mmol) in toluene (15 cm3) was cooled to 0 °C. AlEt3 (1.1 cm3, 0.6 M solution in heptane, 0.66 mmol) was added dropwise to the cooled solution. The resultant mixture was warmed to room temperature and stirred 8 h. BnOH (0.156 cm3 1.5 mmol) was added to the solution. The resultant mixture was stirred overnight. The color of the solution changed from deep red to light yellow. Solvents were removed in vacuo and the residue was dissolved in diethyl ether. The solution was filtered and the filtrate was concentrated to afford yellow crystalline solid (0.24 g, 64%). Anal. calcd for C40H41AlN2O3·0.4C4H10O: C, 76.35; H, 6.93; N, 4.28%. Found: C, 76.33 H, 6.93; N, 4.19. 1H NMR (400 MHz, CDCl3): δ 9.56–9.40 (m, 1H, Ar), 8.45 (d, J = 8.0 Hz, 1H, Ar), 8.33 (d, J = 8.8 Hz, 1H, Ar), 8.28 (d, J = 8.9 Hz, 1H, Ar), 7.89–7.78 (m, 3H, Ar), 7.62 (s, 1H, Ar), 7.59 (s, 1H, Ar), 7.10 (b, 4H, Ar), 7.02 (b, 6H, Ar), 5.05 (s, 2H), 4.88 (s, 2H), 3.47 (q, J = 7.0 Hz, Et2O), 1.60 (s, 9H, But), 1.41 (s, 9H, But), 1.21 (t, J = 7.0 Hz, Et2O). 13C NMR (101 MHz, CDCl3): δ 162.63, 160.31, 149.89, 141.31, 139.52, 139.21, 138.74, 138.13, 138.03, 129.70, 127.79, 127.60, 126.26, 126.18, 125.53, 125.01, 124.97, 124.46, 124.37, 122.75, 118.43, 66.01, 65.86, 35.85, 34.50, 31.74, 30.03, 15.44.
Polymerization of ε-caprolactone
A typical polymerization procedure was exemplified using 2a/BnOH as the catalyst. Complex 2a (24.5 mg, 0.0594 mmol) was dissolved in toluene (5.4 cm3) at 50 °C. To the stirred solution BnOH (0.60 cm3, 0.1 M solution in toluene, 0.06 mmol) and ε-CL (0.63 cm3, 5.94 mmol) were successively added. The polymerization reaction was terminated after 5 h by addition of several drops of glacial acetic acid. The mixture was stirred at room temperature for 30 min. The resulting solution was dropped into cool methanol with stirring. The white precipitate was filtered under reduced pressure and washed with cool methanol. For GPC analysis, the sample was dissolved in THF, passed through a short neutral aluminum oxide column, precipitated in methanol, and dried under vacuum.
Polymerization of rac-β-BL followed the same procedure as for the ROP of ε-CL.
Polymerization of rac-lactide
A typical polymerization procedure was exemplified using 2a/BnOH as the catalyst. Complex 2a (19.0 mg, 0.046 mmol) and BnOH (0.46 cm3, 0.1 M solution in toluene, 0.046 mmol) were added in sequence into toluene (2.0 cm3). The resulting mixture was added to a stirred mixture of rac-LA (0.66 g, 4.60 mmol) and toluene (2.1 cm3) at 70 °C. The polymerization reaction was terminated after 24 h by adding several drops of glacial acetic acid. The mixture was stirred at room temperature for 30 min. The resulting solution was dropped into cool methanol with stirring. The white precipitate was filtered under reduced pressure and washed with cool methanol. For GPC analysis, the sample was dissolved in THF, passed through a short neutral aluminum oxide column, precipitated in methanol, and dried under vacuum.
X-ray crystallography
Single crystals of complex 2c were mounted in Lindemann capillaries under N2. Diffraction data were collected at 298(2) K on a Bruker Smart CCD area detector with graphite-monochromated Mo Kα radiation (λ = 0.71073 Å). The structure was solved by direct methods using SHELXS-97 (ref. 20) and refined against F2 by full-matrix least squares using SHELXL-97.21 Hydrogen atoms were placed in calculated positions. Crystal data and experimental details of the structure determination are listed in Table 5.
Table 5 Details of the X-ray structure determination of complex 2c
R1 = ∑||Fo| − |Fc||/∑|Fo|, wR2 = [∑w(Fo2 − Fc2)2/∑w(Fo4)]1/2. |
Complex |
2c·C7H8 |
Empirical formula |
C47H49N2OAl |
fw |
684.86 |
Crystal system |
Monoclinic |
Space group |
P2(1)/n |
a (Å) |
13.1762(12) |
b (Å) |
12.9320(11) |
c (Å) |
23.471(2) |
α (deg) |
90 |
β (deg) |
96.3170(10) |
γ (deg) |
90 |
V (Å3) |
3975.1(6) |
Z |
4 |
Dcalcd (g cm−3) |
1.144 |
F(000) |
1464 |
μ (mm−1) |
0.088 |
θ range for data collecn (deg) |
2.21 to 25.02 |
No. of reflns collected |
19 732 |
No. of indep reflns (Rint) |
6997 (Rint = 0.0910) |
Restraints/params |
0/532 |
Goodness of fit on F2 |
1.019 |
Final R indicesa [I > 2σ(I)] |
R1 = 0.0596, wR2 = 0.0853 |
R indices (all data) |
R1 = 0.1763, wR2 = 0.0980 |
Largest diff peak and hole [e Å−3] |
0.156 and −0.159 |
Acknowledgements
We thank the Foundation for Talents of Anhui Province (Grant No. 2008Z011) for financial support. We also thank Professor D.-Q. Wang for the crystal structure determination.
Notes and references
-
(a) J. C. Wu, T. L. Yu, C. T. Chen and C. C. Lin, Coord. Chem. Rev., 2006, 250, 602–626 CrossRef CAS;
(b) G.-Q. Chen, Chem. Soc. Rev., 2009, 38, 2434–2446 RSC;
(c) T. K. Dash and V. B. Konkimalla, J. Controlled Release, 2012, 158, 15–33 CrossRef CAS PubMed;
(d) R. A. Cairncross, J. G. Becker, S. Ramaswamy and R. O'Connor, Appl. Biochem. Biotechnol., 2006, 131, 774–785 CrossRef PubMed;
(e) R. Auras, B. Harte and S. Selke, Macromol. Biosci., 2004, 4, 835–864 CrossRef CAS PubMed;
(f) S. Farah, D. G. Anderson and R. Langer, Adv. Drug Delivery Rev., 2016, 107, 367–392 CrossRef CAS PubMed;
(g) C. M. Thomas, Chem. Soc. Rev., 2010, 39, 165–173 RSC;
(h) Y. Q. Zhu, C. Romain and C. K. Williams, Nature, 2016, 540, 354–362 CrossRef CAS PubMed;
(i) N. Spassky, M. Wisniewski, C. Pluta and A. LeBorgne, Macromol. Chem. Phys., 1996, 197, 2627–2637 CrossRef CAS;
(j) Z. Zhong, P. J. Dijkstra and J. Feijen, Angew. Chem., Int. Ed., 2002, 41, 4510–4513 CrossRef CAS;
(k) Z. Zhong, P. J. Dijkstra and J. Feijen, J. Am. Chem. Soc., 2003, 125, 11291–11298 CrossRef CAS PubMed;
(l) Z. Tang, X. Chen, X. Pang, Y. Yang, X. Zhang and X. Jing, Biomacromolecules, 2004, 5, 965–970 CrossRef CAS PubMed;
(m) N. Nomura, R. Ishii, Y. Yamamoto and T. Kondo, Chem.–Eur. J., 2007, 13, 4433–4451 CrossRef CAS PubMed;
(n) X. Pang, R. Duan, X. Li, Z. Sun, H. Zhang, X. Wang and X. Chen, Polym. Chem., 2014, 5, 6857–6864 RSC;
(o) N. Nomura, A. Akita, R. Ishii and M. Mizuno, J. Am. Chem. Soc., 2010, 132, 1750–1751 CrossRef CAS PubMed;
(p) G. Li, M. Lamberti, D. Pappalardo and C. Pellecchia, Macromolecules, 2012, 45, 8614–8620 CrossRef CAS;
(q) Y. Wang and H. Ma, Chem. Commun., 2012, 48, 6729–6731 RSC;
(r) L. Li, B. Liu, D. Liu, C. Wu, S. Li, B. Liu and D. Cui, Organometallics, 2014, 33, 6474–6480 CrossRef CAS;
(s) A. Pilone, N. De Maio, K. Press, V. Venditto, D. Pappalardo, M. Mazzeo, C. Pellecchia, M. Kol and M. Lamberti, Dalton Trans., 2015, 44, 2157–2165 RSC;
(t) W. Li, H. Ouyang, L. Chen, D. Yuan, Y. Zhang and Y. Yao, Inorg. Chem., 2016, 55, 6520–6524 CrossRef CAS PubMed;
(u) X. Wang, V. Dorcet, Y. Luo, J.-F. Carpentier and E. Kirillov, Dalton Trans., 2016, 45, 12346–12351 RSC;
(v) C.-Y. Hsu, H.-C. Tseng, J. K. Vandavasi, W.-Y. Lu, L.-F. Wang, M. Y. Chiang, Y.-C. Lai, H.-Y. Chen and H.-Y. Chen, RSC Adv., 2017, 7, 18851–18860 RSC;
(w) J. Zhang, S. Liu, W. Zuo, H. Ye and Z. Li, New J. Chem., 2017, 41, 2358–2363 RSC.
-
(a) M. J. Stanford and A. P. Dove, Chem. Soc. Rev., 2010, 39, 486–494 RSC;
(b) R. H. Platel, L. M. Hodgson and C. K. Williams, Polym. Rev., 2008, 48, 11–63 CrossRef CAS;
(c) P. J. Dijkstra, H. Du and J. Feijen, Polym. Chem., 2011, 2, 520–527 RSC;
(d) L. T. Lim, R. Auras and M. Rubino, Prog. Polym. Sci., 2008, 33, 820–852 CrossRef CAS.
- M. Labet and W. Thielemans, Chem. Soc. Rev., 2009, 38, 3484–3504 RSC.
- J.-F. Carpentier, Macromol. Rapid Commun., 2010, 31, 1696–1705 CrossRef CAS PubMed.
-
(a) Y. Sun, J. Xiong, Z. Dai, X. Pan, N. Tang and J. Wu, Inorg. Chem., 2016, 55, 136–143 CrossRef CAS PubMed;
(b) W.-J. Chuang, Y.-T. Huang, Y.-H. Chen, Y.-S. Lin, W.-Y. Lu, Y.-C. Lai, M. Y. Chiang, S. C. N. Hsu and H.-Y. Chen, RSC Adv., 2016, 6, 33014–33021 RSC;
(c) H.-W. Ou, K.-H. Lo, W.-T. Du, W.-Y. Lu, W.-J. Chuang, B.-H. Huang, H.-Y. Chen and C.-C. Lin, Inorg. Chem., 2016, 55, 1423–1432 CrossRef CAS PubMed;
(d) F. M. Garcia-Valle, R. Estivill, C. Gallegos, T. Cuenca, M. E. G. Mosquera, V. Tabernero and J. Cano, Organometallics, 2015, 34, 477–487 CrossRef CAS;
(e) D. Alhashmialameer, N. Ikpo, J. Collins, L. N. Dawe, K. Hattenhauer and F. M. Kerton, Dalton Trans., 2015, 44, 20216–20231 RSC;
(f) R. K. Dean, A. M. Reckling, H. Chen, L. N. Dawe, C. M. Schneider and C. M. Kozak, Dalton Trans., 2013, 42, 3504–3520 RSC;
(g) S.-C. Rosca, D.-A. Rosca, V. Dorcet, C. M. Kozak, F. M. Kerton, J.-F. Carpentier and Y. Sarazin, Dalton Trans., 2013, 42, 9361–9375 RSC;
(h) H.-Y. Chen, L. Mialon, K. A. Abboud and S. A. Miller, Organometallics, 2012, 31, 5252–5261 CrossRef CAS;
(i) J. Zhang, C. Jian, Y. Gao, L. Wang, N. Tang and J. Wu, Inorg. Chem., 2012, 51, 13380–13389 CrossRef CAS PubMed;
(j) W.-Y. Lu, M.-W. Hsiao, S. C. N. Hsu, W.-T. Peng, Y.-J. Chang, Y.-C. Tsou, T.-Y. Wu, Y.-C. Lai, Y. Chen and H.-Y. Chen, Dalton Trans., 2012, 41, 3659–3667 RSC;
(k) N. Ikpo, C. Hoffmann, L. N. Dawe and F. M. Kerton, Dalton Trans., 2012, 41, 6651–6660 RSC;
(l) B. Calvo, M. G. Davidson and D. Garcia-Vivo, Inorg. Chem., 2011, 50, 3589–3595 CrossRef CAS PubMed;
(m) Y. Huang, Y.-H. Tsai, W.-C. Hung, C.-S. Lin, W. Wang, J.-H. Huang, S. Dutta and C.-C. Lin, Inorg. Chem., 2010, 49, 9416–9425 CrossRef CAS PubMed;
(n) A. K. Sutar, T. Maharana, S. Dutta, C.-T. Chen and C.-C. Lin, Chem. Soc. Rev., 2010, 39, 1724–1746 RSC;
(o) B. T. Ko and C. C. Lin, J. Am. Chem. Soc., 2001, 123, 7973–7977 CrossRef CAS PubMed.
-
(a) M. G. Cushion and P. Mountford, Chem. Commun., 2011, 47, 2276–2278 RSC;
(b) J. P. Davin, J.-C. Buffet, T. P. Spaniol and J. Okuda, Dalton Trans., 2012, 41, 12612–12618 RSC;
(c) Y. Sarazin, B. Liu, T. Roisnel, L. Maron and J.-F. Carpentier, J. Am. Chem. Soc., 2011, 133, 9069–9087 CrossRef CAS PubMed;
(d) M.-W. Hsiao and C.-C. Lin, Dalton Trans., 2013, 42, 2041–2051 RSC;
(e) H.-Y. Chen, H.-Y. Tang and C.-C. Lin, Polymer, 2007, 48, 2257–2262 CrossRef CAS;
(f) Y. Wang, W. Zhao, X. Liu, D. Cui and E. Y. X. Chen, Macromolecules, 2012, 45, 6957–6965 CrossRef CAS;
(g) T. Rosen, I. Goldberg, V. Venditto and M. Kol, J. Am. Chem. Soc., 2016, 138, 12041–12044 CrossRef CAS PubMed;
(h) W. Yi and H. Ma, Dalton Trans., 2014, 43, 5200–5210 RSC;
(i) L. Wang and H. Ma, Macromolecules, 2010, 43, 6535–6537 CrossRef CAS;
(j) H. Xie, Z. Mou, B. Liu, P. Li, W. Rong, S. Li and D. Cui, Organometallics, 2014, 33, 722–730 CrossRef CAS;
(k) J. Wu, Y.-Z. Chen, W.-C. Hung and C.-C. Lin, Organometallics, 2008, 27, 4970–4978 CrossRef CAS;
(l) L. F. Sanchez-Barba, A. Garces, M. Fajardo, C. Alonso-Moreno, J. Fernandez-Baeza, A. Otero, A. Antinolo, J. Tejeda, A. Lara-Sanchez and M. I. Lopez-Solera, Organometallics, 2007, 26, 6403–6411 CrossRef CAS;
(m) B. M. Chamberlain, M. Cheng, D. R. Moore, T. M. Ovitt, E. B. Lobkovsky and G. W. Coates, J. Am. Chem. Soc., 2001, 123, 3229–3238 CrossRef CAS PubMed.
-
(a) S. Ghosh, R. R. Gowda, R. Jagan and D. Chakraborty, Dalton Trans., 2015, 44, 10410–10422 RSC;
(b) P. Horeglad, M. Cybularczyk, A. Litwinska, A. M. Dabrowska, M. Dranka, G. Z. Zukowska, M. Urbanczyk and M. Michalak, Polym. Chem., 2016, 7, 2022–2036 RSC;
(c) F. Hild, N. Neehaul, F. Bier, M. Wirsum, C. Gourlaouen and S. Dagorne, Organometallics, 2013, 32, 587–598 CrossRef CAS;
(d) I. Yu, A. Acosta-Ramirez and P. Mehrkhodavandi, J. Am. Chem. Soc., 2012, 134, 12758–12773 CrossRef CAS PubMed;
(e) D. C. Aluthge, J. M. Ahn and P. Mehrkhodavandi, Chem. Sci., 2015, 6, 5284–5292 RSC;
(f) A. F. Douglas, B. O. Patrick and P. Mehrkhodavandi, Angew. Chem., Int. Ed., 2008, 47, 2290–2293 CrossRef CAS PubMed;
(g) N. Maudoux, T. Roisnel, V. Dorcet, J.-F. Carpentier and Y. Sarazin, Chem.–Eur. J., 2014, 20, 6131–6147 CrossRef CAS PubMed;
(h) M. Normand, T. Roisnel, J. F. Carpentier and E. Kirillov, Chem. Commun., 2013, 49, 11692–11694 RSC;
(i) A. Pietrangelo, M. A. Hillmyer and W. B. Tolman, Chem. Commun., 2009, 2736–2737 RSC;
(j) M. Normand, V. Dorcet, E. Kirillov and J.-F. Carpentier, Organometallics, 2013, 32, 1694–1709 CrossRef CAS;
(k) Y.-L. Hsieh, N. Huang, G.-H. Lee and C.-H. Peng, Polymer, 2015, 72, 281–291 CrossRef CAS;
(l) B.-H. Huang, C.-Y. Tsai, C.-T. Chen and B.-T. Ko, Dalton Trans., 2016, 45, 17557–17580 RSC;
(m) Y. Wei, S.-W. Wang and S.-L. Zhou, Dalton Trans., 2016, 45, 4471–4485 RSC.
-
(a) V. Poirier, T. Roisnel, S. Sinbandhit, M. Bochmann, J.-F. Carpentier and Y. Sarazin, Chem.–Eur. J., 2012, 18, 2998–3013 CrossRef CAS PubMed;
(b) L. Wang, S.-C. Rosca, V. Poirier, S. Sinbandhit, V. Dorcet, T. Roisnel, J.-F. Carpentier and Y. Sarazin, Dalton Trans., 2014, 43, 4268–4286 RSC;
(c) W.-A. Ma and Z.-X. Wang, Dalton Trans., 2011, 40, 1778–1786 RSC;
(d) A. J. Chmura, C. J. Chuck, M. G. Davidson, M. D. Jones, M. D. Lunn, S. D. Bull and M. F. Mahon, Angew. Chem., Int. Ed., 2007, 46, 2280–2283 CrossRef CAS PubMed;
(e) J. Guo, P. Haquette, J. Martin, K. Salim and C. M. Thomas, Angew. Chem., Int. Ed., 2013, 52, 13584–13587 CrossRef CAS PubMed.
-
(a) Y.-L. Duan, J.-X. He, W. Wang, J.-J. Zhou, Y. Huang and Y. Yang, Dalton Trans., 2016, 45, 10807–10820 RSC;
(b) N. Ajellal, D. M. Lyubov, M. A. Sinenkov, G. K. Fukin, A. V. Cherkasov, C. M. Thomas, J.-F. Carpentier and A. A. Trifonov, Chem.–Eur. J., 2008, 14, 5440–5448 CrossRef CAS PubMed;
(c) J. Jenter, P. W. Roesky, N. Ajellal, S. M. Guillaume, N. Susperregui and L. Maron, Chem.–Eur. J., 2010, 16, 4629–4638 CrossRef CAS PubMed;
(d) H. Ma, T. P. Spaniol and J. Okuda, Inorg. Chem., 2008, 47, 3328–3339 CrossRef CAS PubMed;
(e) Y. Cui, W. Gu, Y. Wang, B. Zhao, Y. Yao and Q. Shen, Catal. Sci. Technol., 2015, 5, 3302–3312 RSC;
(f) W. Gu, P. Xu, Y. Wang, Y. Yao, D. Yuan and Q. Shen, Organometallics, 2015, 34, 2907–2916 CrossRef CAS;
(g) Z. Mou, B. Liu, X. Liu, H. Xie, W. Rong, L. Li, S. Li and D. Cui, Macromolecules, 2014, 47, 2233–2241 CrossRef CAS;
(h) C. Bakewell, A. J. P. White, N. J. Long and C. K. Williams, Angew. Chem., Int. Ed., 2014, 53, 9226–9230 CrossRef CAS PubMed;
(i) C. Bakewell, C. Thi-Phuong-Anh, N. Long, X. F. Le Goff, A. Auffrant and C. K. Williams, J. Am. Chem. Soc., 2012, 134, 20577–20580 CrossRef CAS PubMed;
(j) J.-F. Carpentier, Organometallics, 2015, 34, 4175–4189 CrossRef CAS.
-
(a) A. B. Biernesser, K. R. Delle Chiaie, J. B. Curley and J. A. Byers, Angew. Chem., Int. Ed., 2016, 55, 5251–5254 CrossRef CAS PubMed;
(b) A. B. Biernesser, B. Li and J. A. Byers, J. Am. Chem. Soc., 2013, 135, 16553–16560 CrossRef CAS PubMed;
(c) S. Fortun, P. Daneshmand and F. Schaper, Angew. Chem., Int. Ed., 2015, 54, 13669–13672 CrossRef CAS PubMed;
(d) D. Mandal, D. Chakraborty, V. Ramkumar and D. K. Chand, RSC Adv., 2016, 6, 21706–21718 RSC;
(e) A. J. Chmura, M. G. Davidson, M. D. Jones, M. D. Lunn, M. F. Mahon, A. F. Johnson, P. Khunkamchoo, S. L. Roberts and S. S. F. Wong, Macromolecules, 2006, 39, 7250–7257 CrossRef CAS;
(f) A. Sauer, A. Kapelski, C. Fliedel, S. Dagorne, M. Kol and J. Okuda, Dalton Trans., 2013, 42, 9007–9023 RSC;
(g) X.-X. Zheng, C. Zhang and Z.-X. Wang, J. Organomet. Chem., 2015, 783, 105–115 CrossRef CAS;
(h) M. Honrado, A. Otero, J. Fernandez-Baeza, L. F. Sanchez-Barba, A. Garces, A. Lara-Sanchez and A. M. Rodriguez, Dalton Trans., 2014, 43, 17090–17100 RSC;
(i) Y. Yang, H. Wang and H. Ma, Inorg. Chem., 2015, 54, 5839–5854 CrossRef CAS PubMed;
(j) X.-F. Yu, C. Zhang and Z.-X. Wang, Organometallics, 2013, 32, 3262–3268 CrossRef CAS;
(k) S. Abbina and G. Du, ACS Macro Lett., 2014, 3, 689–692 CrossRef CAS PubMed;
(l) C. K. Williams, L. E. Breyfogle, S. K. Choi, W. Nam, V. G. Young, M. A. Hillmyer and W. B. Tolman, J. Am. Chem. Soc., 2003, 125, 11350–11359 CrossRef CAS PubMed;
(m) M. Cheng, A. B. Attygalle, E. B. Lobkovsky and G. W. Coates, J. Am. Chem. Soc., 1999, 121, 11583–11584 CrossRef CAS.
-
(a) D. Pappalardo, L. Annunziata and C. Pellecchia, Macromolecules, 2009, 42, 6056–6062 CrossRef CAS;
(b) L. Chen, W. Li, D. Yuan, Y. Zhang, Q. Shen and Y. Yao, Inorg. Chem., 2015, 54, 4699–4708 CrossRef CAS PubMed;
(c) W.-L. Kong and Z.-X. Wang, Dalton Trans., 2014, 43, 9126–9135 RSC;
(d) J. S. Klitzke, T. Roisnel, E. Kirillov, O. d. L. Casagrande Jr and J.-F. Carpentier, Organometallics, 2014, 33, 309–321 CrossRef CAS;
(e) W.-H. Sun, M. Shen, W. Zhang, W. Huang, S. Liu and C. Redshaw, Dalton Trans., 2011, 40, 2645–2653 RSC;
(f) H.-C. Tseng, M. Y. Chiang, W.-Y. Lu, Y.-J. Chen, C.-J. Lian, Y.-H. Chen, H.-Y. Tsai, Y.-C. Lai and H.-Y. Chen, Dalton Trans., 2015, 44, 11763–11773 RSC;
(g) K. Press, I. Goldberg and M. Kol, Angew. Chem., Int. Ed., 2015, 54, 14858–14861 CrossRef CAS PubMed;
(h) A. Pilone, K. Press, I. Goldberg, M. Kol, M. Mazzeo and M. Lamberti, J. Am. Chem. Soc., 2014, 136, 2940–2943 CrossRef CAS PubMed;
(i) S. Tabthong, T. Nanok, P. Sumrit, P. Kongsaeree, S. Prabpai, P. Chuawong and P. Hormnirun, Macromolecules, 2015, 48, 6846–6861 CrossRef CAS;
(j) X. Pang, R. Duan, X. Li and X. Chen, Polym. Chem., 2014, 5, 3894–3900 RSC;
(k) P. Hormnirun, E. L. Marshall, V. C. Gibson, A. J. P. White and D. J. Williams, J. Am. Chem. Soc., 2004, 126, 2688–2689 CrossRef CAS PubMed;
(l) T. M. Ovitt and G. W. Coates, J. Am. Chem. Soc., 2002, 124, 1316–1326 CrossRef CAS PubMed;
(m) T. M. Ovitt and G. W. Coates, J. Am. Chem. Soc., 1999, 121, 4072–4073 CrossRef CAS;
(n) P. Wang, X. Hao, J. Cheng, J. Chao and X. Chen, Dalton Trans., 2016, 45, 9088–9096 RSC.
- W.-L. Kong, Z.-Y. Chai and Z.-X. Wang, Dalton Trans., 2014, 43, 14470–14480 RSC.
- R. Gebbink, M. Watanabe, R. C. Pratt and T. D. P. Stack, Chem. Commun., 2003, 630–631 RSC.
- M. Save, M. Schappacher and A. Soum, Macromol. Chem. Phys., 2002, 203, 889–899 CrossRef CAS.
-
(a) X. Pang, R. Duan, X. Li, Z. Sun, H. Zhang, X. Wang and X. Chen, RSC Adv., 2014, 4, 57210–57217 RSC;
(b) N. Nomura, R. Ishii, M. Akakura and K. Aoi, J. Am. Chem. Soc., 2002, 124, 5938–5939 CrossRef CAS PubMed.
-
(a) A. Meduri, T. Fuoco, M. Lamberti, C. Pellecchia and D. Pappalardo, Macromolecules, 2014, 47, 534–543 CrossRef CAS;
(b) C. M. Dong, K. Y. Qiu, Z. W. Gu and X. D. Feng, J. Polym. Sci., Part A: Polym. Chem., 2001, 39, 357–367 CrossRef CAS.
-
(a) L. Liu, Z. Y. Wei and M. Qi, Chin. Chem. Lett., 2007, 18, 744–746 CrossRef CAS;
(b) X. Wang, J. Liu, S. Xu, J. Xu, X. Pan, J. Liu, S. Cui, Z. Li and K. Guo, Polym. Chem., 2016, 7, 6297–6308 RSC;
(c) Y. Wang, B. Liu, X. Wang, W. Zhao, D. Liu, X. Liu and D. Cui, Polym. Chem., 2014, 5, 4580–4588 RSC.
-
(a) D. A. Jayawickrama, C. K. Larive, E. F. McCord and D. C. Roe, Magn. Reson. Chem., 1998, 36, 755–760 CrossRef CAS;
(b) C. F. Hansell, P. Espeel, M. M. Stamenović, I. A. Barker, A. P. Dove, F. E. D. Prez and R. K. O'Reilly, J. Am. Chem. Soc., 2011, 133, 13828–13831 CrossRef CAS PubMed.
-
(a) T. Wang, F. Chen, J. Qin, Y.-M. He and Q.-H. Fan, Angew. Chem., Int. Ed., 2013, 52, 7172–7176 CrossRef CAS PubMed;
(b) F. Della Monica, E. Luciano, G. Roviello, A. Grassi, S. Milione and C. Capacchione, Macromolecules, 2014, 47, 2830–2841 CrossRef CAS;
(c) L. F. Tietze, T. Hungerland, A. Duefert, I. Objartel and D. Stalke, Chem.–Eur. J., 2012, 18, 3286–3291 CrossRef CAS PubMed;
(d) T.-Q. Xu, W. Gao, Y. Mu and L. Ye, Polyhedron, 2007, 26, 3357–3362 CrossRef CAS.
- G. M. Sheldrick, Acta Crystallogr., Sect. A: Found. Crystallogr., 1990, 46, 467–473 CrossRef.
- G. M. Sheldrick, SHELXL97: Programs for Structure Refinement, Universität Göttingen, Gösttingen, Germany, 1997 Search PubMed.
Footnote |
† Electronic supplementary information (ESI) available: Copies of MALDI-TOF mass spectra of PLA and PHB, NMR spectra of polymers and 1H and 13C NMR spectra of ligand precursors 1a–1d and complexes 2a–2f. CCDC 1541685. For ESI and crystallographic data in CIF or other electronic format see DOI: 10.1039/c7ra03831c |
|
This journal is © The Royal Society of Chemistry 2017 |