DOI:
10.1039/C7RA02766D
(Paper)
RSC Adv., 2017,
7, 24652-24656
Stereocontrolled C(sp3)–P bond formation with non-activated alkyl halides and tosylates†
Received
7th March 2017
, Accepted 28th April 2017
First published on 8th May 2017
Abstract
The C(sp3)–P bond is formed via the reaction between P–H compounds and non-activated alkyl electrophiles, especially secondary alkyl halides and tosylates. This reaction proceeds via an SN2 mechanism with inversion of configuration, so it can be used to form C–P bonds with stereocontrol from chiral secondary alcohols.
Organic phosphorus compounds are a group of molecules important to a broad range of fields including synthetic chemistry, biology, materials chemistry, catalytic chemistry, coordination chemistry, agriculture, and pharmaceutical chemistry,1 for which reason they have drawn considerable attention. In recent years, reactions catalyzed by transition metals (Pd,2,3 Ni,4,5 Cu6) have been developed to construct C(sp2)–P and C(sp)–P bonds. Nonetheless, longstanding methods of C(sp3)–P synthesis include (1) substitution reactions of carbon electrophiles with alkali metal phosphides or carbon nucleophiles (organolithiums or Grignard reagents) with P–Cl derivatives, which are hindered by air-unstable substrates and low functional group tolerance;7 (2) the Michaelis–Arbusov reaction8 and the base-promoted H-phosphinate alkylation,9 the former of which is limited to the generation of products of primary P-alkyl phosphine oxides and the latter of which is not only limited by the poor compatibility of functional groups due to the employment of strong basic condition (such as BuLi,9a NaH,9b Na,9c LDA,9d and KHMDS,9e) but also not easily applied to the production of secondary alkyl halides.10 In this context, developing a new method for C(sp3)–P synthesis, especially for the secondary C(sp3)–P compounds, remains a serious challenge, particularly because of the problem of β-hydride elimination.11,12
The current work reports a new method of performing the coupling reaction using non-activated primary and secondary alkyl electrophiles with P–H compounds. In this method, LiOMe served as a mild base and TMEDA served as the additive to inhibit β-hydride elimination, and no transition metal catalyst was used. The reaction can be applied to both pentavalent P(O)–H compounds (secondary phosphine oxide, and H-phosphonate) and trivalent P–H compounds, and it provides a one-step method of producing chelating bisphosphine ligands. Moreover, because the reaction proceeds via the SN2 mechanism, it can be considered a generic method for C–P bond formation with stereocontrol.
This work is encouraged by the recent advances in copper-catalyzed cross-coupling of alkyl electrophiles,13 especially for activation of secondary alkyl electrophiles,14 the catalyst system (CuI/TMEDA/LiOMe) developed by our group14c was first used to test the reaction of bromocyclopentane (1a) with diphenylphosphine oxide (2a). The initial results showed a promising yield of 48% (Table 1, entry 1) under room temperature with THF as the solvent. Multiple reaction parameters were further optimized during the development of the method. In a test of various solvents (entries 2–6), polar reagents showed higher yield. For example, a reaction yield of 74% was obtained (entry 6) using NMP as the solvent. An increase of reaction temperature to 40 °C further improved the yield to 89% (entry 7). The reaction still generated a yield of 88% (entry 8), even when CuI was not used as the catalyst, which indicated a reaction mechanism of base-promoted alkyl substitution. The reaction efficiency was reduced by other bases (LiOtBu, NaOtBu, NaOMe, Cs2CO3, K2CO3, KHMDS, LiHMDS) (entries 9–15). TMEDA (N,N,N′,N′-tetramethylethylenediamine) was proved to be critical to the reaction, which may suppress the undesirable side reactions such as the formation of olefin through elimination of hydrogen chloride, as reported previously.14,15 Other additives, including PBu3, PPh3, and DMEDA were also tested, but they were not as efficient as TMEDA (entries 16–19). Results also showed that secondary alkyl tosylate (R-OTs, entry 20) and alkyl iodide (entry 21) were also suitable substrates for this reaction, but inert alkyl chloride was notably less efficient (entry 22).
Table 1 Reaction between 1a and 2a under various conditions

|
Entry |
X |
Catalyst (10 mol%) |
Additive (20 mol%) |
Base (2 equiv.) |
Solvent (0.5 mL) |
Temp. (°C) |
Yielda (%) |
Reaction conditions: R–X (0.25 mmol), HP(O)Ph2 (0.5 mmol), CuI (10 mol%), additive (20 mol%), base (0.5 mmol), GC yields after 24 hours (average of two runs). Isolated yields. |
1 |
Br |
CuI |
TMEDA |
LiOMe |
THF |
25 |
48 |
2 |
Br |
CuI |
TMEDA |
LiOMe |
Toluene |
25 |
12 |
3 |
Br |
CuI |
TMEDA |
LiOMe |
Dioxane |
25 |
21 |
4 |
Br |
CuI |
TMEDA |
LiOMe |
DMF |
25 |
68 |
5 |
Br |
CuI |
TMEDA |
LiOMe |
DMSO |
25 |
65 |
6 |
Br |
CuI |
TMEDA |
LiOMe |
NMP |
25 |
74 |
7 |
Br |
CuI |
TMEDA |
LiOMe |
NMP |
40 |
89 |
8 |
Br |
— |
TMEDA |
LiOMe |
NMP |
40 |
88(85b) |
9 |
Br |
— |
TMEDA |
LiOtBu |
NMP |
40 |
78 |
10 |
Br |
— |
TMEDA |
NaOtBu |
NMP |
40 |
69 |
11 |
Br |
— |
TMEDA |
NaOMe |
NMP |
40 |
71 |
12 |
Br |
— |
TMEDA |
Cs2CO3 |
NMP |
40 |
58 |
13 |
Br |
— |
TMEDA |
K2CO3 |
NMP |
40 |
16 |
14 |
Br |
— |
TMEDA |
LiHMDS |
NMP |
40 |
42 |
15 |
Br |
— |
TMEDA |
KHMDS |
NMP |
40 |
54 |
16 |
Br |
— |
PnBu3 |
LiOMe |
NMP |
40 |
73 |
17 |
Br |
— |
PPh3 |
LiOMe |
NMP |
40 |
63 |
18 |
Br |
— |
DMEDA |
LiOMe |
NMP |
40 |
72 |
19 |
Br |
— |
— |
LiOMe |
NMP |
40 |
55 |
20 |
OTs |
— |
TMEDA |
LiOMe |
NMP |
40 |
85(81b) |
21 |
I |
— |
TMEDA |
LiOMe |
NMP |
40 |
76 |
22 |
Cl |
— |
TMEDA |
LiOMe |
NMP |
40 |
21 |
The results of the current study indicated that the reaction of LiOMe/TMEDA/NMP can be used to generate C(sp3)–P bonds efficiently using the secondary alkyl bromides (or tosylates) and that there was no need for the Cu-catalyst. The reaction was tested further with various substrates (Scheme 1), including cyclic and acyclic secondary alkyl bromides (3a–3d, 3g, 3j) and tosylates (3e, 3f). All of those substrates showed positive reactions regardless of different chain lengths and branching. Primary alkyl electrophiles also gave good to excellent yields under the same reaction conditions (3h–3m), but tertiary alkyl electrophilic reagents did not succeed. Some functional groups were also compatible with the reaction, including dioxolane (3e, 3l), N-Ts (3g), cyano group (3m), amide (3n), ester (3o, 3p) and heterocyclic groups (3k).
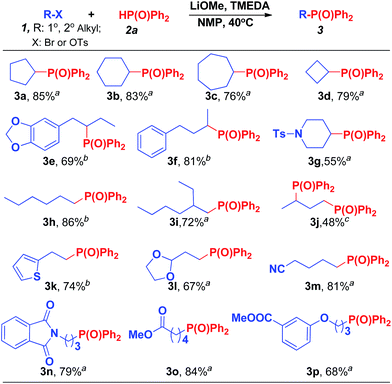 |
| Scheme 1 Electrophiles scope of the coupling reaction. aReactions were carried out at 40 °C for 24 h on a 0.25 mmol scale using R–Br. For details about each substrate please see ESI.† Yields were determined through the isolation of the desired products. b1 equiv. of R-OTs was used. c4 equiv. of 2a and LiOMe were used. | |
Regarding chemoselectivity, results showed the alkyl-OTs site to be more active than the aryl-Br site when both were present in the same substrate. In the reaction of 1b and 2a, the compound with C(sp3)–P bond was the only product, but aryl-bromide remained intact and capable of undergoing further functionalization (Scheme 2) via a coupling reaction. The high selectivity to the groups of sp2-C and sp3-C in the reaction will be useful for the synthesis of complicated compounds.
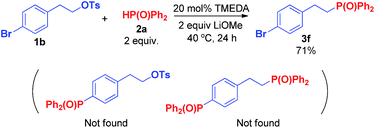 |
| Scheme 2 Selectivity between alkyl and aryl sites. | |
Results also showed that other nucleophiles can also be used in this reaction (Scheme 3). The pentavalent P(O)–H compound (H-phosphonate) can generate a medium yield under standard reaction conditions, but it produces an excellent yield if Cs2CO3 serves as the base instead of LiOMe (5a). More importantly, trivalent P–H compounds can react easily with both primary and secondary alkyl electrophiles (5b–5e), which provided a one-step method for chelating bisphosphine ligands such as dppm (5c), dppp (5d), and dppb (5e).9f,16
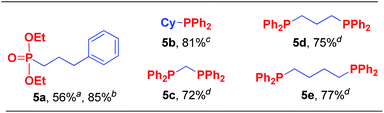 |
| Scheme 3 Nucleophiles scope of the coupling reaction. aReactions were carried out at 40 °C for 24 h on a 0.25 mmol scale using diethyl phosphite and LiOMe. For details about each substrate please see ESI.† Yields were determined through the isolation of the desired products. b2 equiv. of Cs2CO3 was used. c2 equiv. of diphenylphosphine was used. d4 equiv. of diphenylphosphine and LiOMe were used. | |
To understand the reaction mechanism, 6-bromohex-1-ene (6a) first served as the substrate in the reaction, which only generated product 7, with no cyclization product produced (Scheme 4). The results indicated a base-promoting SN2 substitution mechanism rather than radical mechanism.
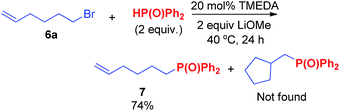 |
| Scheme 4 Radical trapping experiment. | |
Secondary alkyl tosylates can be used in this reaction, which allows the use of chiral substrates to examine the stereochemistry of the reaction. As shown in Scheme 5, chiral tosylate (8a) can be produced through the reaction of chiral alcohols in only one step. 8a and 2a can react to generate product 9a with a good yield. The X-ray crystal analysis of 9a indicated an inversion of configuration, which confirmed the hypothetical SN2 mechanism of the reaction.7d,e
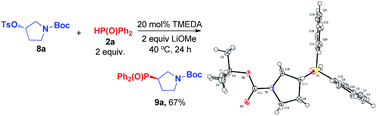 |
| Scheme 5 Inversion of configuration. | |
It is highly important that the reaction can be used to generate C–P bonds with stereocontrol when chiral secondary alcohols are the starting material. As shown in Table 2, cyclic and acyclic chiral alcohols were converted to the corresponding tosylates quantitatively. Through this reaction, diphenylphosphine oxide (2a) reacted with chiral tosylates with good yields, which, after two-step conversions, still remained as high as 95–99% ee%. Because many methods can be used for chiral alcohol synthesis,17 this new method expanded the C–P bond synthesis with stereocontrol.
Table 2 Construction of C–P bonds with stereocontrol from chiral secondary alcoholsa
Conclusions
The current study developed a new method of synthesizing C(sp3)–P compounds through non-activated alkyl halides (or tosylates) with P–H compounds. The reaction is a rare example of phosphorylation with secondary alkyl electrophiles. Both TMEDA and LiOMe have been proven to be important to the efficiency of the reaction. In addition to pentavalent P(O)–H compounds and trivalent P–H compounds have also been found to participate in this process, which provides a convenient, one-step means of synthesizing chelating bisphosphine ligands. The reaction is actually highly selective for alkyl halides, and aryl halides are inert. The X-ray crystal analysis of the reaction products indicated a SN2 reaction mechanism with configuration inversion. In this way, the reaction provides a generic method for using chiral secondary alcohols to produce C–P bond with stereocontrol. Further investigation will be carried out on using tertiary alkyl electrophiles as the reaction substrates with more P–H compounds.
Acknowledgements
Our study was supported by the National Natural Science Foundation of China (21402179, 91426302), and the Science Challenge Project (JCKY2016212A504). We also thank Prof. Shuao Wang and Prof. Juan Diwu for the X-ray crystal analysis and helpful discussions.
Notes and references
-
(a) L. D. Quin, A Guide to Organophosphorus Chemistry, Wiley Interscience, New York, 2000 Search PubMed;
(b) D. M. Karl, Nature, 2000, 406, 31 CrossRef CAS PubMed;
(c) W. Tang and X. Zhang, Chem. Rev., 2003, 103, 3029 CrossRef CAS PubMed;
(d) T. Baumgartner and R. Réau, Chem. Rev., 2006, 106, 4681 CrossRef CAS PubMed;
(e) C. Queffélec, M. Petit, P. Janvier, D. A. Knight and B. Bujoli, Chem. Rev., 2012, 112, 3777 CrossRef PubMed;
(f) L. V. Kochian, Nature, 2012, 488, 466 CrossRef CAS PubMed;
(g) J. W. McGrath, J. P. Chin and J. P. Quinn, Nat. Rev. Microbiol., 2013, 11, 412 CrossRef CAS PubMed;
(h) D. E. C. Corbridge, Phosphorus: Chemistry, Biochemistry and Technology, CRC Press, Boca Raton, FL, 6th edn, 2013, p. 1473 Search PubMed.
- For selected examples, see:
(a) T. Hirao, T. Masunaga, Y. Ohshiro and T. Agawa, Tetrahedron Lett., 1980, 21, 3595 CrossRef CAS;
(b) T. Hirao, T. Masunaga, Y. Ohshiro and T. Agawa, Synthesis, 1981, 56 CrossRef CAS;
(c) T. Hirao, T. Masunaga, N. Yamada, Y. Ohshiro and T. Agawa, Bull. Chem. Soc. Jpn., 1982, 55, 909 CrossRef CAS;
(d) C. Korff and G. Helmchen, Chem. Commun., 2004, 40, 530 RSC;
(e) A. L. Schwan, Chem. Soc. Rev., 2004, 33, 218 RSC;
(f) N. F. Blank, J. R. Moncarz, T. J. Brunker, C. Scriban, B. J. Anderson, O. Amir, D. S. Glueck, L. N. Zakharov, J. A. Golen, C. D. Incarvito and A. L. Rheingold, J. Am. Chem. Soc., 2007, 129, 6847 CrossRef CAS PubMed;
(g) A. J. Bloomfield and S. B. Herzon, Org. Lett., 2012, 14, 4370 CrossRef CAS PubMed;
(h) S. M. Rummelt, M. Ranocchiari and J. A. van Bokhoven, Org. Lett., 2012, 14, 2188 CrossRef CAS PubMed;
(i) E. Jablonkai and G. Keglevich, Tetrahedron Lett., 2013, 54, 4185 CrossRef CAS;
(j) O. Berger, C. Petit, E. L. Deal and J.-L. Montchamp, Adv. Synth. Catal., 2013, 355, 1361 CrossRef CAS;
(k) W. C. Fu, C. M. So and F. Y. Kwong, Org. Lett., 2015, 17, 5906 CrossRef CAS PubMed;
(l) Z. Geng, Y. Zhang, L. Zheng, J. Li, D. Zou, Y. Wu and Y. Wu, Tetrahedron Lett., 2016, 57, 3063 CrossRef CAS;
(m) R. Yu, X. Chen and Z. Wang, Tetrahedron Lett., 2016, 57, 3404 CrossRef CAS.
- Pd-catalyzed Csp2–H phosphorylation reaction:
(a) Y. Kuninobu, T. Yoshida and K. Takai, J. Org. Chem., 2011, 76, 7370 CrossRef CAS PubMed;
(b) C. Hou, Y. Ren, R. Lang, X. Hu, C. Xia and F. Li, Chem. Commun., 2012, 48, 5181 RSC;
(c) C.-G. Feng, M. Ye, K.-J. Xiao, S. Li and J.-Q. Yu, J. Am. Chem. Soc., 2013, 135, 9322 CrossRef CAS PubMed;
(d) W. Liu, S. Wang, H. Yao, Z. Li, Y. Huang and C. Kong, Tetrahedron Lett., 2015, 56, 6100 CrossRef CAS;
(e) L. Yang, X. Zhang, J. Yuan, Y. Xiao and P. Mao, J. Organomet. Chem., 2016, 818, 179 CrossRef CAS.
- For selected examples, see:
(a) D. J. Ager, M. B. East, A. Eisenstadt and S. A. Laneman, Chem. Commun., 1997, 33, 2359 RSC;
(b) D. Villemin, A. Elbilali, F. Simeon, P.-A. Jaffrès, G. Maheut, M. Mosaddak and A. Hakiki, J. Chem. Res., 2003, 2003, 436 CrossRef;
(c) L.-B. Han, C. Zhang, H. Yazawa and S. Shimada, J. Am. Chem. Soc., 2004, 126, 5080 CrossRef CAS PubMed;
(d) Q. Yao and S. Levchik, Tetrahedron Lett., 2006, 47, 277 CrossRef CAS;
(e) M. V. Reddington, Bioconjugate Chem., 2007, 18, 2178 CrossRef CAS PubMed;
(f) G. Yang, C. Shen, L. Zhang and W. Zhang, Tetrahedron Lett., 2011, 52, 5032 CrossRef CAS;
(g) Y.-L. Zhao, G.-J. Wu, Y. Li, L.-X. Gao and F.-S. Han, Chem.–Eur. J., 2012, 18, 9622 CrossRef CAS PubMed;
(h) J. Yang, T. Chen and L.-B. Han, J. Organomet. Chem., 2016, 820, 120 CrossRef CAS;
(i) J. Yang, J. Xiao, T. Chen, S.-F. Yin and L.-B. Han, Chem. Commun., 2016, 52, 12233 RSC.
- Ni-catalyzed C–O/P–H cross coupling reaction:
(a) J. Yang, T. Chen and L.-B. Han, J. Am. Chem. Soc., 2015, 137, 1782 CrossRef CAS PubMed;
(b) J. Yang, J. Xiao, T. Chen and L.-B. Han, J. Org. Chem., 2016, 81, 3911 CrossRef CAS PubMed.
- For selected examples, see:
(a) S. Kaye, J. M. Fox, F. A. Hicks and S. L. Buchwald, Adv. Synth. Catal., 2001, 343, 789 CrossRef CAS;
(b) D. Gelman, L. Jiang and S. L. Buchwald, Org. Lett., 2003, 5, 2315 CrossRef CAS PubMed;
(c) J. J. Becker and M. R. Gagne, Organometallics, 2003, 22, 4984 CrossRef CAS;
(d) C. Huang, X. Tang, H. Fu, Y. Jiang and Y. Zhao, J. Org. Chem., 2006, 71, 5020 CrossRef CAS PubMed;
(e) H. Rao, Y. Jin, H. Fu, Y. Jiang and Y. Zhao, Chem.–Eur. J., 2006, 12, 3636 CrossRef CAS PubMed;
(f) M. Niu, H. Fu, Y. Jiang and Y. Zhao, Chem. Commun., 2007, 43, 272 RSC;
(g) Y. Gao, G. Wang, L. Chen, P. Xu, Y. Zhao, Y. Zhou and L.-B. Han, J. Am. Chem. Soc., 2009, 131, 7956 CrossRef CAS PubMed;
(h) G. Evano, K. Tadiparthi and F. Couty, Chem. Commun., 2011, 47, 179 RSC;
(i) R. Zhuang, J. Xu, Z. Cai, G. Tang, M. Fang and Y. Zhao, Org. Lett., 2011, 13, 2110 CrossRef CAS PubMed;
(j) M. Fañanás-Mastral and B. L. Feringa, J. Am. Chem. Soc., 2014, 136, 9894 CrossRef PubMed;
(k) J. Oliv er-Messeguer, L. Liu, S. García-García, C. Canós-Giménez, . Domínguez, R. Gavara, A. Doménech-Carbó, P. Concepción, A. Leyva-Pérez and A. Corma, J. Am. Chem. Soc., 2015, 137, 3894 CrossRef PubMed;
(l) Q. Chen, X. Yan, C. Wen, J. Zeng, Y. Huang, X. Liu and K. Zhang, J. Org. Chem., 2016, 81, 947 Search PubMed.
- For selected examples, see:
(a) R. B. King, J. Bakos, C. D. Hoff and L. Markó, J. Org. Chem., 1979, 44, 1729 CrossRef CAS;
(b) H. Brunner and H. Leyerer, J. Organomet. Chem., 1987, 334, 369 CrossRef CAS;
(c) Y. Miyamoto, M. Yamashita and T. Oshikawa, Bull. Chem. Soc. Jpn., 1992, 65, 2004 CrossRef CAS;
(d) P. O'Brien and S. Warren, J. Chem. Soc., Perkin Trans. 1, 1996, 2567 RSC;
(e) C. Carcedo, A. Dervisi, I. A. Fallis, L. Ooi and K. M. A. Malik, Chem. Commun., 2004, 1236 RSC;
(f) Z. Liu and H. Du, Org. Lett., 2010, 12, 3054 CrossRef CAS PubMed;
(g) X. Wang, Z. Han, Z. Wang and K. Ding, Angew. Chem., Int. Ed., 2011, 51, 936 CrossRef PubMed.
- For selected examples, see:
(a) A. K. Bhattachary and G. Thyarajan, Chem. Rev., 1981, 81, 415 CrossRef;
(b) P.-Y. Renard, P. Vayron, E. Leclerc, A. Valleix and C. Mioskowski, Angew. Chem., Int. Ed., 2003, 42, 2389 CrossRef CAS PubMed;
(c) P.-Y. Renard, P. Vayron and C. Mioskowski, Org. Lett., 2003, 5, 1661 CrossRef CAS PubMed;
(d) G. Yang, C. Shen, L. Zhang and W. Zhang, Tetrahedron Lett., 2011, 52, 5032 CrossRef CAS;
(e) G. G. Rajeshwaran, M. Nandakumar, R. Sureshbabu and A. K. Mohanakrishnan, Org. Lett., 2011, 13, 1270 CrossRef CAS PubMed;
(f) C. S. Demmer, N. Krogsgaard-Larsen and L. Bunch, Chem. Rev., 2011, 111, 7981 CrossRef CAS PubMed.
- For selected examples, see:
(a) K. Hemmi, H. Takeno, M. Hashimoto and T. Kamiya, Chem. Pharm. Bull., 1982, 30, 111 CrossRef CAS PubMed;
(b) A. C. Baillie, C. L. Cornell, B. J. Wright and K. Wright, Tetrahedron Lett., 1992, 33, 5133 CrossRef CAS;
(c) E. K. Baylis, Tetrahedron Lett., 1995, 36, 9385 CrossRef CAS;
(d) J. Kehler, B. Ebert, O. Dahl and P. Krogsgaard-Larsen, Tetrahedron, 1999, 55, 771 CrossRef CAS;
(e) R. A. Fairhurst, S. P. Collingwood, D. Lambert and R. J. Taylor, Synlett, 2001, 467 CAS;
(f) M. T. Honaker, B. J. Sandefur, J. L. Hargett, A. L. McDaniel and R. N. Salvatore, Tetrahedron Lett., 2003, 44, 8373 CrossRef CAS;
(g) I. Abrunhosa-Thomas, C. E. Sellers and J.-L. Montchamp, J. Org. Chem., 2007, 72, 28 CrossRef PubMed;
(h) J. L. Montchamp, Acc. Chem. Res., 2014, 47, 77 CrossRef CAS PubMed.
- For activated alkyl halides, see:
(a) K. Bravo-Altamirano, Z. H. Huang and J. L. Montchamp, Tetrahedron, 2005, 61, 6315 CrossRef CAS;
(b) L. Coudray and J. L. Montchamp, Eur. J. Org. Chem., 2008, 4101 CrossRef CAS PubMed;
(c) G. Lavén and J. Stawinski, Synlett, 2009, 225 Search PubMed.
- An alternative method for the formation of secondary C(sp3)–P compounds is hydrophosphination of alkene. However, examples of Markovnikov addition are scarce, only for restricted substrates. See:
(a) M. R. Douglass, C. L. Stern and T. J. Marks, J. Am. Chem. Soc., 2001, 123, 10221 CrossRef CAS PubMed;
(b) M. A. Kazankova, M. O. Shulyupin and I. P. Beletskaya, Synlett, 2003, 2155 CAS;
(c) L. Routaboul, F. Toulgoat, J. Gatignol, J.-F. Lohier, B. Norah, O. Delacroix, C. Alayrac, M. Taillefer and A.-C. Gaumont, Chem.–Eur. J., 2013, 19, 8760 CrossRef CAS PubMed;
(d) P. E. Sues, A. J. Lough and R. H. Morris, J. Am. Chem. Soc., 2014, 136, 4746 CrossRef CAS PubMed.
- Another alternative method for the formation of secondary C(sp3)–P compounds is Cu-Catalyzed Csp3–H phosphorylation reaction of activated substrates. See:
(a) J. Ke, Y. Tang, H. Yi, Y. Li, Y. Cheng, C. Liu and A. Lei, Angew. Chem., Int. Ed., 2015, 54, 6604 CrossRef CAS PubMed (aryl ketone O-acetyloximes); ;
(b) H. Zhi, S. P.-M. Ung, Y. Liu, L. Zhao and C.-J. Li, Adv. Synth. Catal., 2016, 358, 2553 CrossRef CAS (glycine derivatives).
- For selected examples, see:
(a) J. Terao, H. Todo, S. A. Begum, H. Kuniyasu and N. Kambe, Angew. Chem., Int. Ed., 2007, 46, 2086 CrossRef CAS PubMed;
(b) G. Cahiez, O. Gager and J. Buendia, Angew. Chem., Int. Ed., 2010, 49, 1278 CrossRef CAS PubMed;
(c) C.-T. Yang, Z.-Q. Zhang, Y.-C. Liu and L. Liu, Angew. Chem., Int. Ed., 2011, 50, 3904 CrossRef CAS PubMed;
(d) P. Ren, L.-A. Stern and X. Hu, Angew. Chem., Int. Ed., 2012, 51, 9110 CrossRef CAS PubMed;
(e) Z.-Q. Zhang, C.-T. Yang, L.-J. Liang, B. Xiao, X. Lu, J.-H. Liu, Y.-Y. Sun, T. B. Marder and Y. Fu, Org. Lett., 2014, 16, 6342 CrossRef CAS PubMed;
(f) X.-Y. Lu, C.-T. Yang, J.-H. Liu, Z.-Q. Zhang, X. Lu, X. Lou, B. Xiao and Y. Fu, Chem. Commun., 2015, 51, 2388 RSC.
- For selected examples, see:
(a) M. Sai, H. Someya, H. Yorimitsu and K. Oshima, Org. Lett., 2008, 10, 2545 CrossRef CAS PubMed;
(b) C.-T. Yang, Z.-Q. Zhang, H. Tajuddin, C.-C. Wu, J. Liang, J.-H. Liu, Y. Fu, M. Czyzewska, P. G. Steel, T. B. Marder and L. Liu, Angew. Chem., Int. Ed., 2012, 51, 528 CrossRef CAS PubMed;
(c) C.-T. Yang, Z.-Q. Zhang, J. Liang, J.-H. Liu, X.-Y. Lu, H.-H. Chen and L. Liu, J. Am. Chem. Soc., 2012, 134, 11124 CrossRef CAS PubMed;
(d) R. Shen, T. Iwasaki, J. Teraob and N. Kambe, Chem. Commun., 2012, 48, 9313 RSC;
(e) J.-H. Liu, C.-T. Yang, X.-Y. Lu, Z.-Q. Zhang, L. Xu, M. Cui, X. Lu, B. Xiao, Y. Fu and L. Liu, Chem.–Eur. J., 2014, 20, 15334 CrossRef CAS PubMed;
(f) A. H. Cherney, N. T. Kadunce and S. E. Reisman, Chem. Rev., 2015, 115, 9587 CrossRef CAS PubMed;
(g) F.-X. Luo, X. Xu, D. Wang, Z.-C. Cao, Y.-F. Zhang and Z.-J. Shi, Org. Lett., 2016, 18, 2040 CrossRef CAS PubMed.
-
(a) D. Noda, Y. Sunada, T. Hatakeyama, M. Nakamura and H. Nagashima, J. Am. Chem. Soc., 2009, 131, 6079 Search PubMed;
(b) T. Hatakeyama, T. Hashimoto, Y. Kondo, Y. Fujiwara, H. Seike, H. Takaya, Y. Tamada, T. Ono and M. Nakamura, J. Am. Chem. Soc., 2010, 132, 10674 CrossRef CAS PubMed;
(c) T. Hatakeyama, Y. Okada, Y. Yoshimoto and M. Nakamura, Angew. Chem., Int. Ed., 2011, 50, 10973 CrossRef CAS PubMed.
-
(a) E. Vaillard, C. Mück-Lichtenfeld, S. Grimme and A. Studer, Angew. Chem., Int. Ed., 2007, 46, 6533 CrossRef PubMed;
(b) Y. Li, S. Das, S. Zhou, K. Junge and M. Beller, J. Am. Chem. Soc., 2012, 134, 9727 CrossRef CAS PubMed.
- For reviews:
(a) E. J. Corey and C. J. Helal, Angew. Chem., Int. Ed., 1998, 37, 1986 CrossRef CAS;
(b) F. Schmidt, R. T. Stemmler, J. Rudolph and C. Bolm, Chem. Soc. Rev., 2006, 35, 454 CAS;
(c) M. Shibasaki and M. Kanai, Chem. Rev., 2008, 108, 2835 CrossRef PubMed.
Footnote |
† Electronic supplementary information (ESI) available: Experimental details, NMR data. CCDC 1510283. For ESI and crystallographic data in CIF or other electronic format see DOI: 10.1039/c7ra02766d |
|
This journal is © The Royal Society of Chemistry 2017 |
Click here to see how this site uses Cookies. View our privacy policy here.