DOI:
10.1039/C7RA02699D
(Paper)
RSC Adv., 2017,
7, 18690-18695
Singlet oxygen generation properties of an inclusion complex of cyclic free-base porphyrin dimer and fullerene C60†
Received
6th March 2017
, Accepted 22nd March 2017
First published on 28th March 2017
Abstract
To gain insight into the singlet oxygen (1O2) generation properties of supramolecular complexes of cyclic free-base porphyrin dimer with fullerene C60, we evaluated the 1O2 quantum yield (ΦΔ) and rate constant (Kobs) of 1O2 generation for a cyclic free-base porphyrin dimer (CPD) linked by butadiyne bearing four 4-pyridyl groups and its inclusion complex (C60⊂CPD) with C60. We demonstrate that CPD and C60⊂CPD possess the ability to generate 1O2 under visible light irradiation. Moreover, it was found that the ΦΔ value of C60⊂CPD is lower than that of CPD. Based on the kinetic and thermodynamic consideration concerning the electron transfer processes between the porphyrin dimer and C60, this work revealed that the lower ΦΔ value of the C60 inclusion complex would be attributed to the formation of the charge-separated state C60·−-CPD·+, leading to a low intersystem crossing (ISC) efficiency for the formation of the triplet excited state 3(CPD)*.
Introduction
Photosensitizers possessing the ability to generate singlet oxygen (1O2) have created considerable interest in recent years from the viewpoint of fundamental studies in photochemistry and their potential applications in photodynamic therapy (PDT).1–6 These photosensitizers generally produce 1O2 through the following processes: initially the photosensitizer absorbs light (hν) to generate the singlet excited state of the photosensitizer (1S*), then the photoexcited sensitizer (1S*) undergoes intersystem crossing (ISC) to generate the triplet excited state (3S*). Subsequent energy transfer from the photoexcited sensitizer (3S*) to triplet oxygen (3O2) produces 1O2. Thus, to generate 3S* efficiency is one of the most effective strategies to give high 1O2 quantum yield (ΦΔ). In particular, porphyrin dyes have been regarded as promising candidates for photosensitizers as a result of their strong Soret (400–500 nm) and moderate Q band (500–700 nm) absorption properties, as well as their electrochemical, photochemical and thermal stabilities. The typical photosensitizer, free-base tetraphenylporphyrin (H2TPP) exhibits ΦΔ value of 0.6–0.7 (in benzene).7 Much effort in molecular design and development of porphyrin photosensitizers have been made to further improve the ΦΔ value so far.7–11 Some researchers reported that porphyrin–fullerene C60 dyads as well as boron dipyrromethene (BODIPY)-C60 dyads exhibits a higher ΦΔ value than each porphyrin or C60, which is attributed to the formation of long-lived triplet excited state (3S*) by the effective ISC due to intramolecular energy transfer or electron transfer (charge separation) between photosensitizer and C60.11,12
Recently, we have designed and developed cyclic free-base porphyrin dimer CPD linked by butadiyne bearing four 4-pyridyl groups and its inclusion complex C60⊂CPD with C60 (Fig. 1).13 It was expected that C60⊂CPD has favorable photochemical and electrochemical properties for PDT through the electrochemical measurements and the transient absorption spectroscopy, based on the fact that the singlet excited state C60-1(CPD)* undergoes intrasupramolecular electron transfer to give a completely charge-separated state C60·−-CPD·+. Thus, in this work, to gain insight into the 1O2 generation properties of supramolecular complex of cyclic free-base porphyrin dimer with C60, we evaluated the ΦΔ and rate constant (Kobs) of 1O2 generation for CPD and C60⊂CPD. Here we reveal that cyclic free-base porphyrin dimer and its inclusion complex with fullerene C60 possess the ability to generate 1O2 under visible light irradiation, based on the kinetic and thermodynamic consideration concerning the electron transfer processes between the porphyrin dimer and C60.
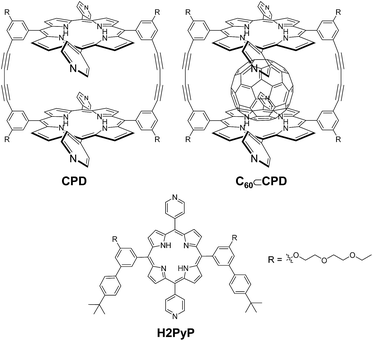 |
| Fig. 1 Chemical structures of cyclic free-base porphyrin dimer (CPD) linked by butadiyne bearing four 4-pyridyl groups, its inclusion complex (C60⊂CPD) with C60 and ABAB porphyrin monomer H2PyP as a reference. | |
Results and discussion
The cyclic free-base porphyrin dimer CPD in CH2Cl2/MeOH exhibits strong Soret band at around 420 nm and relatively weak Q band in the range 500–650 nm (Fig. 2, λabsmax/nm (ε/M−1 cm−1) = 416 (708
000), 514 (31
200), 548 (7400), 587 (9000), 642 (2900)). The molar extinction coefficients (ε) of Soret and Q bands for CPD are higher than those of H2PyP (λabsmax/nm (ε/M−1 cm−1) = 418 (419
000), 513 (19
200), 548 (6200), 588 (6000), 643 (3000))13e as an ABAB porphyrin monomer with two pyridyl groups and two phenyl groups. The fact is attributed to the porphyrin dimer structure of CPD with two porphyrin units. For the C60 inclusion complex C60⊂CPD, it is difficult to obtain its exact absorption spectra because the 1
:
1 complex of CPD with C60 is in dissociation equilibrium in solution of 10−5 to 10−6 M concentration which is suitable for the measurement of photoabsorption spectra of porphyrins. In our previous work, however, we have demonstrated that upon addition of C60 to the solution of the cyclic porphyrin dimer, its Soret band was redshifted with a decrease in intensity, whereas its Q band was slightly redshifted but increased in intensity.13 1O2 generation by CPD, C60⊂CPD or H2PyP in CH2Cl2/MeOH (=1/1, v/v) was evaluated by monitoring the photoabsorption spectral change of the known 1O2 scavenger 1,3-diphenylisobenzofuran (DPBF) accompanied by the reaction of DPBF with the generated 1O2, that is, DPBF can trap 1O2 through its photooxidation.14 CH2Cl2/MeOH was bubbled with air for 15 min. The air-saturated solution containing CPD, C60⊂CPD or H2PyP and DPBF was irradiated with 509 nm (300 μW cm−2, ε = 27
300 M−1 cm−1@λabs = 509 nm for CPD and ε = 17
000 M−1 cm−1@λabs = 509 nm for H2PyP, respectively) obtained by passage of xenon light through monochromator. For both CPD and C60⊂CPD as well as H2PyP the absorption band of DPBF at around 410 nm decreased with the increase in the photoirradiation time (Fig. 3), which indicate the reaction of DPBF with 1O2 generated upon the excitation of the porphyrin dimer. To gain insight into the effect of the cyclic porphyrin dimers on the efficiency of DPBF photooxidation, the changes in optical density (ΔOD) of DPBF are plotted against the photoirradiation time (Fig. 4a), and the slope (msl) is used to estimate the ΦΔ value for CPD, C60⊂CPD and H2PyP. The msl value (−1.5 × 10−2) of H2PyP is larger than those of CPD (−1.2 × 10−2) and C60⊂CPD (−9.8 × 10−3). Moreover, it was revealed that the msl value of CPD is larger than that of C60⊂CPD. Thus, the ΦΔ values of CPD, C60⊂CPD and H2PyP were estimated by the relative method using Rose Bengal (RB) (ΦΔ = 0.80, msl = −1.5 × 10−2, see Fig. S1†) in methanol15 as the standard (Table 1). The ΦΔ value of CPD, C60⊂CPD and H2PyP is 0.62, 0.52 and 0.91 respectively, which is in good agreement with the msl value. This result suggests that as for the ABAB porphyrin monomer H2PyP the ISC efficiency from 1S* to the 3S* may be higher than in the cyclic free-base porphyrin dimer CPD. Moreover, it is worth noting that the ΦΔ value of C60⊂CPD is lower than that of CPD. Our previous work demonstrates that the decay of photoexcited state of C60⊂CPD has two steps (Fig. 5): the first step has a lifetime of 18 ps, which corresponds to the disappearance of the singlet excited state of C60-1(CPD)* (ca. −3.6 eV), that is, C60-1(CPD)* undergoes intrasupramolecular electron transfer to give a completely charge-separated state C60·−-CPD·+ (ca. −3.7 eV).13 C60·−-CPD·+ decays with a lifetime of 470 ps to the ground state. The singlet excited state C60-1(CPD)* has a slower ISC to its triplet excited state C60-3(CPD)* (ca. −4.0 eV), in addition, the C60-3(CPD)* would undergo energy transfer to C60, leading to the formation of 3C60*-(CPD). Thus, on the basis of the photodynamics of the cyclic free-base porphyrin dimer and its inclusion complex with C60, the lower ΦΔ value of the C60 inclusion complex would be attributed to the formation of charge-separated state, leading to low ISC efficiency because the ISC is in kinetically competition with the intrasupramolecular electron transfer, that is, the formation of triplet excited state 3(CPD)* is in kinetically unfavorable compared to that of the charge-separated state C60·−-CPD·+.
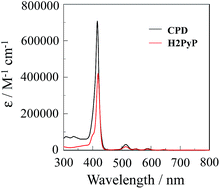 |
| Fig. 2 Photoabsorption spectra of CPD and H2PyP in CH2Cl2/MeOH. | |
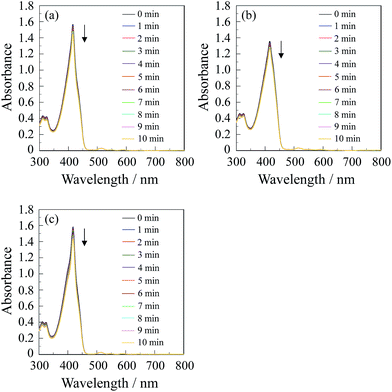 |
| Fig. 3 Photoabsorption spectral changes for the photooxidation of DPBF (Abs. = ca. 1.0) using (a) CPD (1.3 × 10−6 M), (b) C60⊂CPD (1.0 × 10−6 M) and (c) H2PyP (1.4 × 10−6 M) as photosensitizer under photoirradiation with 509 nm (300 μW cm−2) in CH2Cl2/MeOH (=1/1, v/v). | |
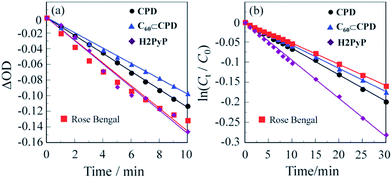 |
| Fig. 4 (a) Plots of ΔOD for DPBF against the photoirradiation time for the photooxidation of DPBF using CPD, C60⊂CPD, H2PyP or Rose Bengal as photosensitizers under photoirradiation with 509 nm (300 μW cm−2) in CH2Cl2/MeOH (=1/1, v/v) and MeOH, respectively. (b) Plots of ln(Ct/C0) for DHN against the photoirradiation time for the photooxidation of DHN using CPD, C60⊂CPD, H2PyP or Rose Bengal as photosensitizers under photoirradiation with visible light (>385 nm, 30 mW cm−2) in CH2Cl2/MeOH (=1/1, v/v). | |
Table 1 1O2 quantum yield (ΦΔ) and first-order rate constant (Kobs) for the photooxidation of DPBF and DHN in CH2Cl2/MeOH (=1/1, v/v), respectively, using CPD, C60⊂CPD or H2PyP as photosensitizer
Photosensitizer |
ΦΔa |
Kobsb/min−1 |
1O2 quantum yield (relative decomposition rate of DPBF), with Rose Bengal (RB) as standard (ΦΔ = 0.80 in methanol,15 see Fig. S1) and 3-diphenylisobenzofuran (DPBF) as 1O2 scavenger. First-order rate constant for the reaction of DHN with 1O2 generated upon photoexcitation of the photosensitizer. The Kobs for RB is 5.3 × 10−3 min−1 (see Fig. S2). |
CPD |
0.62 |
6.6 × 10−3 |
C60⊂CPD |
0.52 |
5.8 × 10−3 |
H2PyP |
0.91 |
9.5 × 10−3 |
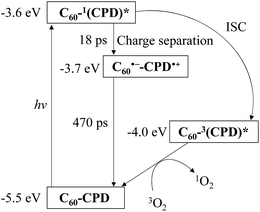 |
| Fig. 5 The photodynamics of C60⊂CPD and 1O2 generation. | |
In order to evaluate the photosensitizing ability of the cyclic free-base porphyrin dimer and its inclusion complex with C60, the ln(Ct/C0) is plotted against the photoirradiation time, where Ct is a concentration of 1,5-dihydroxynaphthalene (DHN) at the reaction time (t) and C0 is the initial concentration of DHN before photoirradiation. CH2Cl2/MeOH (=1/1, v/v) were bubbled with air for 15 min. The air-saturated solution containing CPD or C60⊂CPD and DHN was irradiated with visible light (>385 nm, 30 mW cm−2) obtained by passage of xenon light through a 385 nm long path filter. The photoabsorption spectral changes for the photooxidation of DHN using CPD, C60⊂CPD or H2PyP under photoirradiation with the visible light in CH2Cl2/MeOH (=1/1, v/v) are shown in Fig. 6. Evidently, the absorption band of DHN at around 300 nm decreased with the increase in the photoirradiation time. The plots of ln(Ct/C0) against the photoirradiation time indicate that for CPD, C60⊂CPD and H2PyP the ln(Ct/C0) decreased almost linearly with the increase in the photoirradiation time (Fig. 4b). Thus, this result indicates the ln(Ct/C0) bears a linear relationship with the photoirradiation time to provide the first-order rate constants (Kobs) for the photooxidation of DHN using the cyclic free-base porphyrin dimer or its inclusion complex with C60 as the photosensitizer (Table 1). Obviously, the higher Kobs values of the cyclic free-base porphyrin dimer and its inclusion complex with C60 relative to RB (see Fig. S2†) are due to the contribution of the strong Soret band of the cyclic free-base porphyrin skeleton, although the Kobs values of CPD and C60⊂CPD are lower than that of H2PyP (9.5 × 10−3 min−1). It is worth noting here that the Kobs value (6.6 × 10−3 min−1) of CPD is greater than that (5.8 × 10−3 min−1) of C60⊂CPD. Therefore, this result demonstrates that CPD exhibits more efficient photosensitizing ability due to the effective ISC compared to C60⊂CPD.
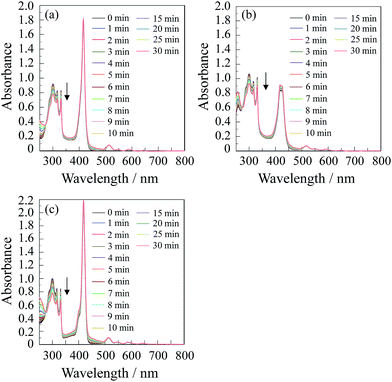 |
| Fig. 6 Photoabsorption spectral changes for the photooxidation of DHN (1.0 × 10−4 M) using (a) CPD (2.5 × 10−6 M), (b) C60⊂CPD (2.5 × 10−6 M) and (c) H2PyP (5.0 × 10−6 M) as photosensitizer under photoirradiation with visible light (>385 nm, 30 mW cm−2) in CH2Cl2/MeOH (=1/1, v/v). | |
In addition, we performed an electron paramagnetic resonance (EPR) method with 2,2,6,6-tetramethyl-4-piperidone (4-oxo-TEMP) as the spin-trapping agent, which can react with 1O2 to produce 4-oxo-TEMPO as a stable nitroxide radical.16 When the air-saturated solution containing CPD, C60⊂CPD or H2PyP and 4-oxo-TEMP was irradiated with visible light (>385 nm, 30 mW cm−2) obtained by passage of xenon light through a 385 nm long path filter, for both the free-base porphyrin dimer and its inclusion complex with C60 as well as H2PyP the ESR spectra of 4-oxo-TEMPO were clearly observed as a characteristic 1
:
1
:
1 triplet (Fig. 7). Consequently, this work demonstrated that the cyclic free-base porphyrin dimer and its inclusion complex with C60 possess the ability to generate 1O2 under visible light irradiation.
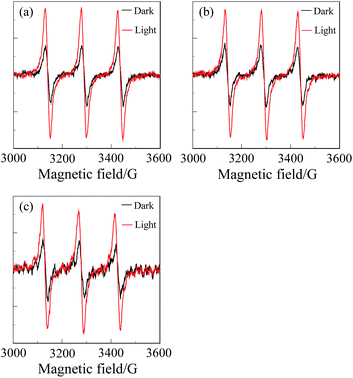 |
| Fig. 7 The ESR spectra of 4-oxo-TEMPO which is formed by the reaction of 4-oxo-TEMP with 1O2 which was generated by (a) CPD, (b) C60⊂CPD and (c) H2PyP under irradiation with visible light (temperature 298 K, microwave power 1 mW, microwave frequency 9.439 GHz, and field modulation 0.2 mT at 100 kHz). The air-saturated CH2Cl2 solution containing CPD (2.5 × 10−6 M), C60⊂CPD (2.5 × 10−6 M) or H2PyP (5.0 × 10−6 M) as the photosensitizer and 4-oxo-TEMP (50 mM) as the spin-trapping agent was irradiated with visible light (>385 nm, 30 mW cm−2 for 1 h) obtained by passage of xenon light through a 385 nm long path filter, where CH2Cl2 as low polar solvent was used because it was difficult to obtain a clear ESR signal in polar solvent such as CH2Cl2/MeOH (=1/1, v/v). | |
Conclusions
To investigate singlet oxygen (1O2) generation properties of cyclic free-base porphyrin dimer and its inclusion complex with fullerene C60, we evaluated the 1O2 quantum yield (ΦΔ) and rate constant (Kobs) of 1O2 generation for cyclic free-base porphyrin dimer CPD and its inclusion complex C60⊂CPD with C60. It was found that the ΦΔ value of C60⊂CPD is lower than that of CPD. The lower ΦΔ value of the C60 inclusion complex would be attributed to the formation of charge-separated state C60·−-CPD·+, leading to low intersystem crossing (ISC) efficiency for the formation of triplet excited state 3(CPD)*, although it was expected that the formation of C60·−-CPD·+ is favorable for 1O2 generation. Consequently, this work demonstrates that the cyclic free-base porphyrin dimer and its supramolecular complex with C60 possess the ability to generate 1O2 under visible light irradiation.
Experimental
Evaluation of 1O2 quantum yield
Quantum yield (ΦΔ) for singlet oxygen (1O2) generation by cyclic free-base porphyrin dimer CPD, its inclusion complex C60⊂CPD with fullerene C60 and H2PyP in CH2Cl2/MeOH (=1/1, v/v) was evaluated by monitoring the photoabsorption spectral change of the known 1O2 scavenger 1,3-diphenylisobenzofuran (DPBF) accompanied by the reaction of DPBF with the generated 1O2, that is, DPBF can trap 1O2 through its photooxidation. CH2Cl2/MeOH was bubbled with air for 15 min. The absorbance of DPBF was adjusted to around 1.0 in air-saturated solvent. Concentration of CPD, C60⊂CPD or H2PyP was adjusted with an absorbance of ca. 0.03 at the irradiation wavelength (509 nm). The air-saturated solution containing the photosensitizer (CPD, C60⊂CPD or H2PyP) and DPBF was irradiated with 509 nm (300 μW cm−2) obtained by passage of xenon light through monochromator. The photoabsorption spectral change of DPBF with the photoirradiation was monitored with an interval of 1 min up to 10 min. The absorption band of DPBF at around 410 nm decreased with the increase in the photoirradiation time. The changes in optical density (ΔOD) of DPBF are plotted against the photoirradiation time, and the slope is used to estimate the ΦΔ values of CPD, C60⊂CPD and H2PyP. The ΦΔ values of CPD, C60⊂CPD and H2PyP were estimated by the relative method using Rose Bengal (RB) (ΦΔ = 0.80) in methanol as the standard. Therefore, the 1ΦΔ values were calculated according to the following eqn (1): |
ΦΔsam = ΦΔref × [(msam/mref) × (Lref/Lsam)]
| (1) |
where ΦΔsam and ΦΔref are the 1O2 quantum yield of photosensitizer (CPD, C60⊂CPD or H2PyP) and RB, respectively, msam and mref are the slope of the difference (ΔOD) in the change in the absorption maximum wavelength of DPBF (around 410 nm) which are plotted against the photoirradiation time, Lsam and Lref are the light harvesting efficiency, which is given by L = 1 − 10−A (“A” is the absorbance at the photoirradiation wavelength).
Photosensitizing ability
Photosensitizing ability of CPD, C60⊂CPD and H2PyP in CH2Cl2/MeOH was evaluated by plotting the ln(Ct/C0) against the photoirradiation time, where Ct is a concentration of 1,5-dihydroxynaphthalene (DHN) at the reaction time (t) and C0 is the initial concentration of DHN before photoirradiation. CH2Cl2/MeOH was bubbled with air for 15 min. The air-saturated solution containing the photosensitizer (2.5 × 10−6 M for CPD and C60⊂CPD, 5.0 × 10−6 M for H2PyP and 2.5 × 10−6 M for RB) and DHN (1.0 × 10−4 M) was irradiated with visible light (>385 nm, 30 mW cm−2) obtained by passage of xenon light through a 385 nm long path filter. The photooxidation of DHN with the photoirradiation was monitored by following the decrease in the photoabsorption at around 300 nm with an interval of 1 min up to 10 min and then an interval of 5 min up to 30 min. The concentration (Ct) of DHN at the reaction time (t) was calculated based on Lambert–Beer law (ADPBF = εcl). The ln(Ct/C0) decreased almost linearly with the increase in the photoirradiation time due to the photooxidation of DHN, that is, the slope was used to estimate the rate constants (Kobs).
1O2 detection by EPR spin-trapping method with 4-oxo-TEMP
The EPR spectra were recorded on a JEOL JES-RE1X spectrometer under the following experimental conditions: temperature 298 K, microwave power 1 mW, microwave frequency 9.439 GHz, and field modulation 0.2 mT at 100 kHz. The air-saturated CH2Cl2 solution containing CPD (2.5 × 10−6 M), C60⊂CPD (2.5 × 10−6 M) or H2PyP (5.0 × 10−6 M) as the photosensitizer and 4-oxo-TEMP (50 mM) as the spin-trapping agent was irradiated with visible light (>385 nm, 30 mW cm−2 for 1 h) obtained by passage of xenon light through a 385 nm long path filter. The ESR spectrum of 4-oxo-TEMPO which is formed by the reaction of 4-oxo-TEMP with 1O2, was clearly observed as a characteristic 1
:
1
:
1 triplet (Fig. 7).
Notes and references
- J. F. Lovell, T. W. B. Liu, J. Chen and G. Zheng, Chem. Rev., 2010, 110, 2839 CrossRef CAS PubMed.
- M. C. DeRosa and R. J. Crutchley, Coord. Chem. Rev., 2002, 233–234, 351 CrossRef CAS.
- M. Pawlicki, H. A. Collins, R. G. Denning and H. L. Anderson, Angew. Chem., Int. Ed., 2009, 48, 3244 CrossRef CAS PubMed.
- K. A. Leonard, M. I. Nelen, L. T. Anderson, S. L. Gibson, R. Hilf and M. R. Detty, J. Med. Chem., 1999, 42, 3942 CrossRef CAS PubMed.
- J. M. Dąbrowski and L. G. Arnaut, Photochem. Photobiol. Sci., 2015, 14, 1765 Search PubMed.
- T. Patrice, Photodynamic Therapy, Royal Society of Chemistry, 2003 Search PubMed.
-
(a) R. Bonnett, Chem. Soc. Rev., 1995, 24, 19 RSC;
(b) M. Ethirajan, Y. Chen, P. Joshi and R. K. Pandey, Chem. Soc. Rev., 2011, 40, 340 RSC.
-
(a) J. P. Belair, C. J. Ziegler, C. S. Rajesh and D. A. Modarelli, J. Phys. Chem. A, 2002, 106, 6445 CrossRef CAS;
(b) P. C. Lo, J. D. Huang, D. Y. Y. Cheng, E. Y. M. Chan, W. P. Fong, W. H. Ko and D. K. P. Ng, Chem.–Eur. J., 2004, 10, 4831 CrossRef CAS PubMed;
(c) A. Karotki, M. Khurana, J. R. Lepock and B. C. Wilson, Photochem. Photobiol., 2006, 82, 443 CrossRef CAS PubMed;
(d) L. Delanaye, M. A. Bahri, F. Tfibel, M.-P. Fontaine-Aupart, A. Mouithys-Mickalad, B. Heine, J. Piette and M. Hoebeke, Photochem. Photobiol. Sci., 2006, 5, 317 RSC;
(e) M. Morone, L. Beverina, A. Abbotto, F. Silvestri, E. Collini, C. Ferrante, R. Bozio and G. A. Pagani, Org. Lett., 2006, 8, 2719 CrossRef CAS PubMed;
(f) M. Khurana, H. A. Collins, A. Karotki, H. L. Anderson, D. T. Cramb and B. C. Wilson, Photochem. Photobiol., 2007, 84, 1441 CrossRef PubMed.
-
(a) A. P. Thomas, P. S. S. Babu, S. A. Nair, S. Ramakrishnan, D. Ramaiah, T. K. Chandrashekar, A. Srinivasan and M. R. Pillai, J. Med. Chem., 2012, 55, 5110 CrossRef CAS PubMed;
(b) K. Hirakawa, Y. Nishimura, T. Arai and S. Okazaki, J. Phys. Chem. B, 2013, 117, 13490 CrossRef CAS PubMed;
(c) H. Horiuchi, M. Hosaka, H. Mashio, M. Terata, S. Ishida, S. Kyushin, T. Okutsu, T. Takeuchi and H. Hiratsuka, Chem.–Eur. J., 2014, 20, 6054 CrossRef CAS PubMed;
(d) Q. Yu, E. M. Rodriguez, R. Naccache, P. Forgione, G. Lamoureux, F. Sanz-Rodriguez, D. Scheglman and J. A. Capobianco, Chem. Commun., 2014, 50, 12150 RSC;
(e) D. Yao, V. Hugues, M. Blanchard-Desce, O. Mongin, C. O. Paul-Roth and F. Paul, New J. Chem., 2015, 39, 7730 RSC.
-
(a) M. Prein and W. Adam, Angew. Chem., Int. Ed., 2014, 53, 6938 CrossRef PubMed;
(b) H. Shinmori, F. Kodaira, S. Matsugo, S. Kawabata and A. Osuka, Chem. Lett., 2005, 34, 322 CrossRef CAS;
(c) L. G. Arnaut, M. M. Pereira, J. M. Dąbrowski, E. F. F. Silva, F. A. Schaberle, A. R. Abreu, L. B. Rocha, M. M. Barsan, K. Urbańska, G. Stoche and C. M. A. Brett, Chem.–Eur. J., 2014, 20, 5346 CrossRef CAS PubMed;
(d) F. Hammerer, G. Garcia, S. Chen, F. Royer, S. Achelle, C. Fiorini-Debuisschert, M.-P. Telulade-Fichou and P. Maillard, J. Org. Chem., 2014, 79, 1406 CrossRef CAS PubMed;
(e) J. Schmitt, V. Heitz, A. Sour, F. Bolze, H. Ftouni, J.-F. Nicoud, L. Flamigni and B. Ventura, Angew. Chem., Int. Ed., 2015, 54, 169 CrossRef CAS PubMed.
- M. E. Milanesio, M. G. Alvarez, V. Rivarola, J. J. Silber and E. N. Durantini, Photochem. Photobiol., 2005, 81, 891 CrossRef CAS PubMed.
-
(a) L. Huang, X. Yu, W. Wu and J. Zhao, Org. Lett., 2012, 14, 2594 CrossRef CAS PubMed;
(b) A. Kamkaew, S. H. Lim, H. B. Lee, L. V. Kiew, L. Y. Chung and K. Burgess, Chem. Soc. Rev., 2013, 42, 77 RSC;
(c) L. Huang, X. Cui, B. Therrien and J. Zhao, Chem.–Eur. J., 2013, 19, 17472 CrossRef CAS PubMed.
-
(a) H. Nobukuni, Y. Shimazaki, H. Uno, Y. Naruta, K. Okubo, T. Kojima, S. Fukuzumi, S. Seki, H. Sakai, T. Hasobe and F. Tani, Chem.–Eur. J., 2010, 16, 11611 CrossRef CAS PubMed;
(b) H. Nobukuni, T. Kamimura, H. Uno, Y. Shimazaki, Y. Naruta and F. Tani, Bull. Chem. Soc. Jpn., 2012, 85, 862 CrossRef CAS;
(c) K. Sakaguchi, T. Kamimura, H. Uno, S. Mori, S. Ozako, H. Nobukuni, M. Ishida and F. Tani, J. Org. Chem., 2014, 79, 2980 CrossRef CAS PubMed;
(d) T. Kamimura, K. Ohkubo, Y. Kawashima, H. Nobukuni, Y. Naruta, F. Tani and S. Fukuzumi, Chem. Sci., 2013, 4, 1451 RSC;
(e) T. Kamimura, K. Ohkubo, Y. Kawashima, S. Ozako, K. Sakaguchi, S. Fukuzumi and F. Tani, J. Phys. Chem. C, 2015, 119, 25634 CrossRef CAS;
(f) Y. Ooyama, K. Uenaka, T. Kamimura, S. Ozako, M. Kanda, T. Koide and F. Tani, RSC Adv., 2016, 6, 16150 RSC.
- K. Golinick and A. Griesbeck, Tetrahedron, 1985, 41, 2057 CrossRef.
- W. Wu, J. Sun, X. Cui and J. Zhao, J. Mater. Chem. C, 2013, 1, 4577 RSC.
-
(a) Y. Yamakoshi, N. Umezawa, A. Ryu, K. Arakane, N. Miyata, Y. Goda, T. Masumizu and T. Nagano, J. Am. Chem. Soc., 2003, 125, 12803 CrossRef CAS PubMed;
(b) S. Oriana, S. Aroua, J. O. B. Söllner, X.-J. Ma, Y. Iwamoto and Y. Yamakoshi, Chem. Commun., 2013, 49, 9302 RSC.
Footnote |
† Electronic supplementary information (ESI) available. See DOI: 10.1039/c7ra02699d |
|
This journal is © The Royal Society of Chemistry 2017 |
Click here to see how this site uses Cookies. View our privacy policy here.