DOI:
10.1039/C7RA02076G
(Paper)
RSC Adv., 2017,
7, 28476-28482
Preparation and investigation of temperature-responsive calix[4]arene-based molecular gels†
Received
19th February 2017
, Accepted 15th May 2017
First published on 31st May 2017
Abstract
A novel calix[4]arene-based tetracholesteryl derivative was synthesized, and its gelation behaviours in 30 organic solvents were studied. It showed that 1 gelled long chain alkane, some of alcohol, kerosene, ethyl acetate benzene and toluene. Interestingly the gel of 1/kerosene possessed special property, which was that the mixture solution of the compound 1/kerosene heating for a while was put on the flat contact heater and formed a gel film at 25 °C and 65 °C. Unlike ordinary film, gel film had higher strength and toughness at 65 °C, by contrast other was fragile and broken easily at 25 °C, this phenomenon was few reported before. In order to explore the phenomenon, the mechanism of the gel of 1/kerosene was discussed by Scanning Electron Microscope (SEM), High Nuclear Magnetic Resonance (H NMR), Fourier Transform Infrared Spectrometer (IR) and micro-rheology. SEM revealed that the xerogel of 1/kerosene assembled network through some long and thick fibers at 65 °C, with concentration reducing fibers become thin and its networks were clearly observed. In contrast the morphology of xerogel was porous at low concentration and was a bulk at high temperature system. According to EI data in micro-rheology, the gelation process could be divided into three stages at 65 °C and they could be that some cholesterol segments in the gelator interacting with each other formed some small aggregation in the first stage, and other cholesterol section of the gelator sufficiently were cross-linked with time and assembled into large assembly in the second plateau. In the final moment the large assembly build gel network and the system of gel tended to stable. But there are two stages at low temperature and could be some cholesterol segments that didn't take part in assembly, which may be the cause that the performance of gel was fragile.
Introduction
Physical gels based on self-assembly structure of molecule because of potential application in drug/protein delivery,1,2 oil spill recovery,3–5 have become one of active frontiers of novel materials in recent years. Researcher struggled to prepare novel physical gel by bonding some functional groups and introducing extraordinary metal ions in gelator molecular or solvent.6,7 By far some common gelators containing amino acids, saccharides, cholesterol, azo-and metal-compounds were synthesized and reported.8–13 Relatives to those functional groups, supramolecular hosts possessing unique recognized abilities to some molecular and ions were also introduced to physical gels and endowed gel some smart properties.14–18
Physical gels containing supramolecular hosts have stimuli-responsive to oxidation/reduction,19 sonication,20 temperature,21,22 shear,23 pH24,25 and were widely applied to catalyst,26 separation,27 extraction,28 drug delivery29 and sensor.30 Crown ether is the first generation of supramolecular host and in 1991 was firstly imported to the structure of gelator by Shinkai professor, who and his cooperators synthesized crown ether derivatives suspending cholesterol and used them prepare gels with thermo-, light- and metal-responsive.31 Huang's group created the quadruple-responsive supramolecular polymer network gel, which was constructed by the benzo-21-crown-7 host and its guest dialkylammonium and they existed in the same molecules.32 Cyclodextrins as the second supramolecular host endowed to physical gel special properties. Leong33 prepared smart-shear-thixotropy gel and explained assembling mechanism of gel, in which cyclodextrins (CD) acted as physical crosslinking and induced building supromolecular polymer network. Harada group34 synthesized four polymer gelators ending with α-CD, β-CD, 1-naphthyl, 2-naphthyl and used them preparing four hydrogels in which the gelators containing 1-naphthyl, 2-naphthyl set up again gel network in DMSO by exchanging solvent. In their work, four kind of gels dressing up different colours were put into quartz vessel with a little water and the result showed three gels containing β-CD, 1-naphthyl, 2-naphthyl respectively combined with together when the quartz vessel was shook, other gel possessing α-CD kept in the vessel lonely. Cucurbit is another supramolecular host but recently several gels relating to it have reported.35,36 Yang35 created a thermo-responsive supramolecular hydrogels, which containing butan-1-aminium 4-methylbenzenesulfonate and cucurbit[6]uril. It uncovered the interesting behaviour of the tempting action of cucurbit[6]uril to form [2]pseudorotaxane. Kim36 and his colleagues utilized the any modification of cucurbit[7]uril to prepare pH-responsive hydrogel and excavated the host–guest interaction of cucurbit[7]uril and water/hydronium by X-ray.
Calixarene as the third supramolecular host attracted great attention because they have a big adjustable hydrophobic cavity and multiple modify sites. Calixarene has been studies as a component of gelators.37–44 Our group have reported two gel works based on calix[4]arene and for the first time constructed a novel gelator with calix[4]arene fragment and two cholesterol. The first work of our group based on calix[4]arene was that novel gelator was synthesized and used it prepared smart shear-thixotropy gel in the mixture solvent of decane and acetonitrile.17 Chiral L- and D-phenylalanine residue as a linker connecting calix[4]arene unit and cholesteryl structure in the second work, and gelation tests revealed that isopropanol, only one of the solvents, could be gelled by the compound connecting D-phenylalanine in its linker and mechanical agitation or sonication not only promotes the gelation, but also enhances the strength of the gel.43 The gel showed the excellent properties of shear thixotropy and mechano-responsive, and this could be owe to the gelator combining with calix[4]arene and two cholesterols.17,43 Based on our previous work, we first combined calix[4]arene unit with four cholesterol groups via hexanediamine moieties in the linkers as a novel gelators (compound 1). Compared with preceded gelators, new compound 1 increased two cholesterol units and amine groups, which could drive the formation of gel network. Gelation tests revealed that 1 could long chain alkane, some of alcohol, kerosene, ethyl acetate, benzene, toluene and one interesting property of gel of 1/kerosene was transparent high strength and a certain toughness gel forming immediately at high temperature and become fragile at the low temperature, which might be correlate with mechanism of assembly process. Under certain condition, this special property might have potential application in some special environment. This paper reports the details in the following.
Result and discussion
Gelation behaviours of the compounds
Gelation behaviours of the compounds 1 was studied in 30 different organic solvents including polar/apolar solvents in a concentration of 2.5% (w/v), and the results are summarized in Table 1. It showed that 1 gelled long chain alkane, butanol, pentanol, hexanol, octanol, heptanol, nonanol, kerosene, ethyl acetate benzene and toluene, and found that the gel of 1/kerosene had high strength at high temperature and easily broken at low temperature for a while. The kerosene, mixing hydrocarbons, could be suit to the cavity of gel network at difference temperature and could participate in the formation progress of gel network. This was proved that the network of gel collapsed during vacuum freeze-drying because kerosene might interact with compound 1. In order to explore this phenomenon, two hot solutions of 1/kerosene (2.5%, w/v) were put into two glass model that were placed on two different temperature-controlled flat contact heater, in which the temperature of one contact heater set at 65 °C, the other was 25 °C. So the different temperature of supramolecular gel films were made when gel forming from above-mentioned solution and glass model reached the same temperature. When removing the model, gel film were picked perfectly by a piece of steel sheet-shape at 65 °C, this may be it has better toughness, in contrast other was broken rapidly at 25 °C (Fig. 1), it could be deduced that the cholesterol moieties of compound 1 assembled partially so that the crosslinking densities of the gelator decreased and its gel was easily broken in low temperature and the temperature of gel effected on formation of the gel network, this was a unusual gel behaviour for recent years.
Table 1 Gelation behaviors of compounds 1 (2.5%, w/v)a
Solvent |
1 |
Solvent |
1 |
Solvent |
1 |
S = solution; I = insoluble; P = precipitate; G = turbid gel; G* = gels forming at room temperature. |
Methanol |
I |
n-Hexane |
I |
Acetone |
I |
Ethanol |
P |
n-Heptane |
P |
Benzene |
G |
n-Propanol |
P |
n-Octane |
G |
Toluene |
G |
n-Butanol |
G* |
n-Nonane |
G |
THF |
S |
n-Pentanol |
PG |
n-Decane |
G |
n-Pentane |
P |
n-Hexanol |
G* |
Cyclohexane |
PG |
DMSO |
I |
n-Heptanol |
G* |
Acetonitrile |
I |
DMF |
P |
n-Octanol |
G* |
Isopropanol |
P |
CH2Cl2 |
S |
n-Nonanol |
G* |
Kerosene |
G |
CHCl3 |
S |
n-Decanol |
S |
Ethyl acetate |
G |
CCl4 |
S |
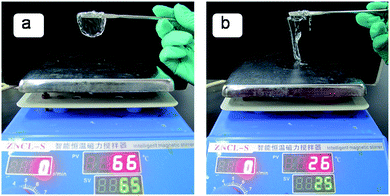 |
| Fig. 1 Photograph of the gel film of 1/kerosene system (2.5%, w/v) at different temperature. | |
Morphology studies
In order to studied the morphologies of the molecular assemblies of the compounds 1, the xerogel system of 1 were observed at different temperature, concentration and solvent via SEM. The gel had been prepared through heating–cooling treatment and placed for a few minute to reach the system balance. The corresponding xerogel of the system of 1/kerosene used in the SEM measurement was prepared by replacing the kerosene of gel with methanol at homologous temperature. For other systems, the process of gelation was applied with the same treatment, and the subsequent xerogels were prepared by freeze-drying in liquid nitrogen, and then evaporated via using a vacuum pump at a temperature of −85 °C for 24 h.
Fig. 2 showed the concentration and temperature-depended xerogel morphology of 1/kerosene gel system with SEM. The xerogel of 1/kerosene assembled network through some long and thick fibers at 65 °C, with gel concentration reducing fibers become thin at 1%, 0.5% (w/v) and its networks were clearly observed in the Fig. 2b. In contrast at 25 °C the morphology of xerogel network was porous and a bulk at high concentration. Based on previous result, it concluded that the assembly of gelator were effected more greatly on temperature than concentration.
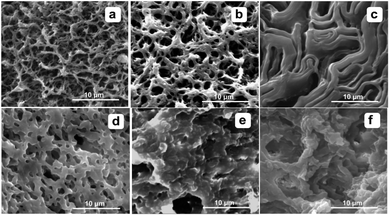 |
| Fig. 2 SEM images of 1/kerosene gel networks with different concentration at different temperature ((a)–(c), at 65 °C; (d)–(f), at 25 °C; (a) and (d), 0.5%, (b) and (c) 1.0%, (b) and (c) 2.0%). | |
The morphologies of the gelator assemblies existing in gels of 1/different solvents were showed in ESI Fig. 1.† With reference to the images shown in Fig. S1,† it is seen that the morphologies of the aggregates of 1 in decane with low polarity were lamellar (Fig. S1a†), when increasing the polarity of the solvent, the aggregates of 1/toluene become into fibers. At even higher polarity, the fibers of gelators blend to form porous network structures in 1/butanol (Fig. S1b†). It can be concluded that by increasing the polarity of solvent, the morphologies of its aggregates change from lamellar and long fibers (Fig. S1†), to fiber-based porous structures, suggesting that the network structures in the gel may be effected significantly by the polarity of solvent.
Rheological and micro-rheological studies
The mechanical property of a gelation was extremely important for its practical uses, and thereby the rheological properties of an example gel system of 1/kerosene were studied systematically. This system was chosen due to its specificity in the property of gelation at different temperature. In order to explore the property in detail, rheological measurements were studied on a series of the samples of the gel, which contained different temperature gels at the same concentration. Firstly, shear stress scanning was conducted by placing the pretreated solution containing kerosene and compound 1, and then leaving the same mixture of solution on different plate of rheometer at 25 °C, 45 °C, 65 °C, 85 °C for 1 h before the scanning in order to create better balance of gel network. In the studies, the storage modulus, G′ (associated with energy storage), and the loss modulus, G′′ (associated with loss of energy) of a sample of the gel were measured as functions of shear stress at a constant frequency of 1.0 Hz at 25 °C, 45 °C, 65 °C, 85 °C (Fig. S2†). At low stress value, the value of G′ was more than the G′′, which showed the dominant elastic character of the gel, while the materials were transformed into rubbery state or become “liquid-like” when G′′ exceeds G′, the value of which was known as yield stress.45 Fig. S2† showed the value of G′ increased and then decreased from 16
280 to 29
212 Pa to 11
395 along with increasing the temperature of the gel from 25 °C to 85 °C, which accounted stability of the gels were well dependent upon the temperature. Furthermore, increasing temperature of a gel also shows positive role in enhancing the mechanical properties of the gel in a certain scope of temperature. But the yield stress correspondingly changed from 68.16 Pa to 230.74 Pa to 214.8 Pa, which rose and lowered with increasing temperature. The result might indicate that the number of crosslinks of the gel network formed differently at lower (25 °C) temperature and higher (45 °C 65 °C 85 °C) temperature. The interaction constructing gel network at 85 °C reduced on account of fast molecular motion and leaded to G′, G′′, the yield stress lessening.
In order to explore the influence of temperature on the crosslink gelation process of compound 1/kerosene, elasticity index (EI) and Solid Liquid Balance (SLB) properties of the gel at different temperature was studied through micro-rheological measurements.46 The result was showed in Fig. 3 and 4. The curve of the EI of 1/kerosene system at 45 °C, 65 °C were decreased rapidly and then reached a short plateau in the beginning of test, and then increased to another plateau in a short time, and finally ascended to the constant with time. The results showed the graph were divided into three stages according to the EI graph and preliminary suggested the gelation process appeared three platform: in the first stage some cholesterol segments in the gelator interacting with each other formed small aggregation and produced some crosslinking sites, and other cholesterol section of the gelator sufficiently assembled into large assembly in the second plateau, in the final stage the large assembly interacted into gel network and the system of gel tended to stable state. At 25 °C, 85 °C Fig. 3 appeared only one plateau, and it could be merely two assembly process: a part cholesterol segments of compound 1 interacted to chunks and the chunks accumulated into network at 25 °C, and difference from the above mentioned all cholesterol segments participated in assembly at 85 °C.
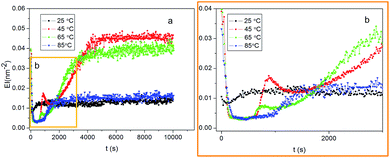 |
| Fig. 3 EI versus times at different temperature (2.5%, w/v). | |
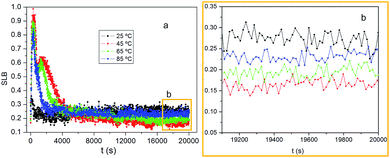 |
| Fig. 4 SLB versus times at different temperature (2.5%, w/v). | |
SLB illuminated the ratio between solid and liquid behaviour, reaction rate. SLB = 0.5 expressed that the liquid and solid parts are equal, known as sol–gel transition point, 0.5 < SLB < 1 expressed the liquid behaviour dominating, and 0 < SLB < 0.5 expressed the solid behaviour dominating, which was gel behaviour.47 As shown in Fig. 4, SLB curves finally remained below 0.5 and above 0 at 25 °C, 45 °C, 65 °C, 85 °C, which declared the solid behaviour dominated. At 45 °C, 65 °C, SLB graphs increased quickly and exceeded 0.5, indicating the system showed liquid behaviour, then rapidly decreased to 0.6 and 0.5 respectively, that shortly stayed, which was set up intersection between cholesterols and it was the same with EI. With time SLB graphs decreases to 0.2 or so and reached a constant, which proved that viscous dominant solution changed into a viscoelastic gel. The SLB curves directly reached to about 0.25, gel phase at 25 °C, 85 °C. As shown in Fig. 3, when crosslinker concentrations range was 0.2 or so, there was an obvious decline of the time, which implicated that the time of sol–gel transition was reduced. Fig. 4b showed the values of balance SLB were about 0.27, 0.15, 0.2, 0.23, corresponding temperature 25 °C, 45 °C, 65 °C, 85 °C, which indicated the degree of crosslinking firstly increased and then decreased with temperature increasing.
1H NMR spectroscopy studies
To obtain further information on the structural nature of the gel networks under study, the aggregation behaviours of the gelators/1 in CDCl3 were studied in detail by conducting temperature-dependent and concentration-dependent 1H NMR measurements. The results showed in Fig. 5. It was seen that the chemical shifts of the amide protons (>9 ppm) of the compounds moved remarkably to up-field along with increasing the temperature of the systems from 298 K to 308 K and then to 318 K. Specifically for compound 1, the shifts are 0.06 and 0.03 ppm, respectively (Fig. 5a), 0.04 and 0.06 ppm, respectively (Fig. 5a), suggesting that both amide groups in compound 1 might have taken part in the aggregation process, and hydrogen bonding could be the most possible mode. Further reference to the data shown in Fig. 5b, it shows that the two signals of the protons of amide groups affixed to the cholesterol structure appeared and took place some displacement (0.10 ppm, 0.05 ppm) at 0.5%, 1.0%, which might be hydrogen bond interaction inner compound 1. With the concentration of systems increasing, the interaction of hydrogen bond of compound 1 moved from intramolecule to intermolecule and the two signals of the protons of amide groups mixed together when the concentration of solution changed from 1.0% to 2.0%. The merge peak shifts 0.03 ppm for the systems concentration changing from 2.0% to 3.0%, in supporting of the argument that hydrogen bonding contributes to the self-assembly of the gelators.
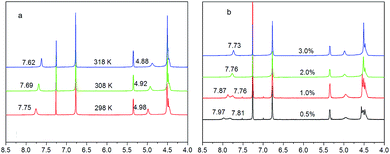 |
| Fig. 5 Partial 1H NMR spectra of 1 in CDCl3 at different temperatures 2.0%, w/v, (a) and at different concentration ((b), room temperature). | |
FTIR spectroscopy studies
As well-known, hydrogen bonding was the main driving force for the self-assembly of cholesterol-based gelator.48 In order to further probe the function of hydrogen bonding in the gelation process, FTIR spectra of the xerogels of 1/kerosene and the CDCl3 solutions of the compound 1 were recorded. Fig. 6 showed that the bands of 1 in its CDCl3 solution at 3416, 1689 and 1680 cm−1 could be assigned to the stretching vibrations of NH and CO, as well as the bending vibration of NH. The band of 3416 cm−1 moved to 3423 cm−1, the other two bands (1689 cm−1 and 1680 cm−1 in solution) fused into one peak, which was 1685 cm−1 in gel. FTIR absorption positions of the carbonyl groups, the hydroxyl groups and the amide groups moved, which confirmed the existence of hydrogen bonds in the gel networks and might drive molecular assembly.
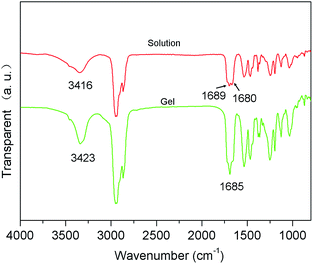 |
| Fig. 6 FTIR spectra of compound 1 in its CDCl3 solutions and xerogels of kerosene/1. | |
Experimental section
Reagents and materials
Cholesteryl chloroformate, hexanediamine (Sinopharm group Co. Ltd) and p-tert-butylphenol (Aladdin Chemistry Co. Ltd., 98%) used directly without further purification. Toluene was distilled over sodium in the presence of benzophenone under N2 atmosphere and collected fraction at 108–110 °C before use. All other reagents were of analytical grade and used directly without further purification.
Synthetic procedures
The synthetic routes of the target compound 1 are schematically shown in Scheme 1. Calix[4]arene and intermediate a are known compounds, and it was synthesized and characterized in the way as reported by us a few years ago.17,43
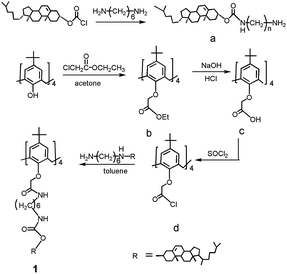 |
| Scheme 1 Synthesis route of compounds 1. | |
Synthesis of intermediate b49. Calix[4]arene (0.648 g, 1 mmol), potassium carbonate (0.825 g, 6 mmol) and potassium iodide (0.650 g, 4 mmol) were dissolved in 20 mL of acetone, and then ethyl chloroacetate (0.742 g, 6 mmol) was added slowly and stirred. When the mixture was refluxed for 24 h under nitrogen atmosphere, ethyl chloroacetate (0.367 g, 3 mmol) was added again and was refluxed for 7 day under the same condition. Most of solvent was evaporated at reduced pressure when the previous reaction finished. The residue as obtained was dried, and then dissolved/suspended in chloroform. The organic system was extracted or washed with diluted hydrochloric acid for three times, and then collected and dried with anhydrous magnesium sulfate. After that, the clear solution was evaporated to dryness. The crude product as prepared was firstly purified by re-crystallization from ethanol to give compound b as white crystals in a yield of 80%. For b: 1H NMR (CDCl3/Me4Si, 300 MHz): δ (ppm): 1.07 (s, 36H, –C(CH2)3), 1.26 (t, 12H, –CH2CH3), 3.17 (d, J = 14.0, 4H, –ArCH2Ar–), 4.18 (q, 8H, –CH2CH3), 4.80 (s, 8H, –ArOCH2), 4.83 (d, J = 14.0, 4H, –ArCH2Ar–), 6.78 (s, 8H, –ArH), HRMS: m/z calcd for [(M + Na)+]: 1015.4180, found: 1015.4170.
Synthesis of intermediate c50. b (1.000 g, 1 mmol) and 15% of NaOH (30 mL) were dissolved in 75 mL of ethanol, and the mixture was stirred and heated under reflux for 24 h. The residue was diluted with cold water (50 mL), then hydrochloric acid (3 mol mL−1) was added with vigorous stirring until pH equals 1, and then abundant white solid as produced was collected via filtration. Finally, the residue was dried in oven (yield: 90%). For c: 1H NMR (CDCl3/Me4Si, 300 MHz): δ (ppm): 1.15 (s, 36H, –C(CH2)3), 3.37 (d, J = 14.0, 4H, –ArCH2Ar–), 4.34 (d, J = 14.0, 4H, –ArCH2Ar–), 4.48 (s, 8H, –ArOCH2), 7.11 (s, 8H, –ArH), and 7.82 (br s, COOH). HRMS: m/z calcd for [(M + Na)+]: 903.4180, found: 903.4170.
Synthesis of intermediate d51. c (0.500 g, 0.56 mmol) was dissolved in 80 mL of toluene containing thionyl chloride (1250 μL), and the mixture was stirred and heated under reflux for 3.5 h. Removal of the solvent and the un-reacted thionyl chloride via distillation under reduced pressure furnished the product, tetracyl chloride (d), as an off-white solid in quantitative yield. The product as obtained was used directly in subsequent preparations without purification.
Preparation of compound 1. a (1.340 g, 2.24 mmol), triethylamine (380 μL) were dissolved in 80 mL of toluene, and then 30 mL of toluene containing d (d is the previous products) was added drop-wise to the above solution with stirring. The mixture was stirred at 0 °C for 12 h. After the reaction, the mixture was filtered, and the solvent in the solution was evaporated. The residue as obtained is the crude product. Purification of the product was conducted by recrystallization from ethanol to give purified product 1, a white power, and obtained in a yield of 50%. For compound 1: 1H NMR (CDCl3/Me4Si, 300 MHz): δ (ppm): 0.68–2.39 (m, 256H, –C(CH3)3, cholesteryl protons), 3.23 (d, J = 12.0, 4H, –ArCH2Ar–), 4.61 (m, 16H, ArCH2CH, –ArOCH2, oxycyclohexyl), ArCH2Ar–, 4.98 m, 2H, –NHCHCO(CH2), 5.33 (S, 4H, alkenyl), 6.82 (m, 8H, –ArH), 7.75 (m, 2H, –NHCHCO(CH2)); HRMS: m/z calcd for [(M + Na)+]: 2945.25, found: 2945.18. Compound 1: C 77.21, H 10.20, N 3.83; found: C 77.57, H 10.22, N 3.73.
General methods
Preparation of gels. In a typical gelation test, the mixture of amount of the possible gelator and a measured volume of the solvent were placed in a sealed test vial, then heating for a while. And then, the system was left to equilibrate for a few minutes. Finally, the test vial was inverted to observe if the solution inside could still flow. The systems which lose fluidity are denoted as “G” and which lose partially are denoted as “PG”. Those only solution remains are denoted as “S”. However, the systems with precipitates or crystals are denoted as “P”. As for the systems, in which the potential gelator could not be dissolved even at the boiling point of the solvent, they are denoted as “I”.SEM pictures of xerogels were taken on a Quanta 200 scanning electron microscopy spectrometer (Philips-FEI). The accelerating voltage was 20 kV, and the emission was 10 mA. The xerogel was prepared by putting a block of gel of 1/kerosene in a beaker containing methanol. The kerosene in the gel diffused into methanol and the previous gel only left the network structure constructed by compound 1. Prior to examination, the xerogel was attached to a copper holder by using conductive adhesive tape, and then it was coated with a thin layer of gold.
All FTIR measurements were performed on a Brucher EQUINX55 spectrometer in an attenuated total reflection (ATR) mode with ZnSe as sample slot. The KBr pellets mixed with samples were measured in transmittance mode.
Rheological measurements were carried out with a stress-controlled rheometer (TA instrument AR-G2) equipped with steel-coated parallel-plate geometry (20 mm diameter). The gap distance was fixed at 1000 μm. The following procedure was used to load the fresh gel sample: three 2.0 mL of solution containing solvent and compound 1 was heated until dissolved and closed with a tight cone, and then cooled down to 10 °C, 25 °C, 45 °C, which were equal to the setting temperature of steel-coated parallel-plate geometry. The measurements started after the gel system reached to balance and placed for 4 hours. A solvent-trapping device was placed on the plate to avoid evaporation.
Micro-rheological measurements was completed by Rheolaser MASTER and traced the assembling process of compound 1 in kerosene. The mixture of a weighted amount of the compound 1, 3 mL of the kerosene and some tracer particle (w/v, 5%) was heated until compound 1 dissolved, then cooled to room temperature, then finally put it into the micro-rheological instrument tested.
1H NMR and HRMS measurements: 1H NMR data of samples were collected on Bruker AVANCF 300 MHz spectrometer. HRMS data were recorded on Bruker Apex IV FTMS with ESI source.
Conclusion
Novel four cholesteryl derivative of calix[4]arene with hexanediamine residues in the linkers was designed and synthesized. Gelation tests in 30 organic solvents demonstrated that 1 gels 14 solvent at room temperature and 1/kerosene could form gel film. Unlike ordinary film, gel film was high strength and toughness at 65 °C, by contrast with other was fragile and broken easily at 25 °C. SEM revealed that the xerogel of 1/kerosene assembled network through some long and thick fibers at 65 °C, with gel concentration reducing the fibers become thin and its networks were clearly observed. By contrast the morphology of xerogel was porous at low concentration and was a bulk at high temperature system. The gelation process could be divided into three stages at high temperature EI graph. But there are two stages at low temperature in EI graph and could be some cholesterol segments that didn't take part in assembly, which may be the cause that the performance of gel was fragile.
Acknowledgements
We thank the International Science Technology Cooperation Program of China (2015DFR50350) and the Natural Science Foundation of Shaanxi Province of China (2014JQ2-2008, 15jk1235) and this work is also supported by Foundation of Weinan Normal University (14YK001, 15ZRRC11).
Notes and references
- A. Klaewklod, V. Tantishaiyakul, N. Hirun, T. Sangfai and L. Li, Mater. Sci. Eng., C, 2015, 50, 242–250 CrossRef CAS PubMed.
- S. M. N. Simões, F. Veiga, A. C. F. Ribeiro, A. R. Figueiras, P. Taboada, A. Concheiro and C. Alvarez-Lorenzo, Eur. J. Pharm. Biopharm., 2014, 87, 579–588 CrossRef PubMed.
- D. Wang, J. Niu, Z. Wang and J. Jin, Langmuir, 2015, 31, 1670–1674 CrossRef CAS PubMed.
- X. Chen, K. Liu, P. He, H. Zhang and Y. Fang, Langmuir, 2012, 28, 9275–9281 CrossRef CAS PubMed.
- P. Lee and M. A. Rogers, Langmuir, 2013, 29, 5617–5621 CrossRef CAS PubMed.
- Y. Zhang, B. Zhang, Y. Kuang, Y. Gao, J. Shi, X. Zhang and B. Xu, J. Am. Chem. Soc., 2013, 135, 5008–5011 CrossRef CAS PubMed.
- Y. Cao, X. Hu, Y. Li, X. Zou, S. Xiong, C. Lin, Y. Shen and L. Wang, J. Am. Chem. Soc., 2014, 136, 10762–10769 CrossRef CAS PubMed.
- X. Li, Y. Kuang, J. Shi, Y. Gao, H. Lin and B. Xu, J. Am. Chem. Soc., 2011, 133, 17513–17518 CrossRef CAS PubMed.
- Y. Li, K. Liu, J. Liu, J. Peng, X. Feng and Y. Fang, Langmuir, 2006, 22, 7016–7020 CrossRef CAS PubMed.
- M. Nakahata, S. Mori, Y. Takashima, A. Hashidzume, H. Yamaguchi and A. Harada, ACS Macro Lett., 2014, 3, 337–340 CrossRef CAS.
- Q. Chen, Y. Lv, D. Zhang, G. Zhang, C. Liu and D. Zhu, Langmuir, 2010, 26, 3165–3168 CrossRef CAS PubMed.
- J. Liu, P. He, J. Yan, X. Fang, J. Peng, K. Liu and Y. Fang, Adv. Mater., 2008, 20, 2508–2511 CrossRef CAS.
- P. Duan, Y. Li, L. Li, J. Deng and M. Liu, J. Phys. Chem. B, 2011, 115, 3322–3329 CrossRef CAS PubMed.
- P. Wei, X. Yan and F. Huang, Chem. Soc. Rev., 2015, 44, 815–832 RSC.
- X. Yang, H. Yu, L. Wang, R. Tong, M. Akram, Y. Chen and X. Zhai, Soft Matter, 2015, 11, 1242–1252 RSC.
- X. Yan, F. Wang, B. Zheng and F. Huang, Chem. Soc. Rev., 2012, 41, 6042–6065 RSC.
- X. Cai, K. Liu, J. Yan, H. Zhang, X. Hou, Z. Liu and Y. Fang, Soft Matter, 2012, 8, 3756–3761 RSC.
- T. Mecca, G. M. L. Messina, G. Marletta and F. Cunsolo, Chem. Commun., 2013, 49, 2530–2532 RSC.
- T. He, K. Li, N. Wang, Y. Liao, X. Wang and X. Yu, Soft Matter, 2014, 10, 3755–3761 RSC.
- E. A. Appel, F. Biedermann, U. Rauwald, S. T. Jones, J. M. Zayed and O. A. Scherman, J. Am. Chem. Soc., 2010, 132, 14251–14260 CrossRef CAS PubMed.
- J. W. Steed, Chem. Commun., 2011, 47, 1379–1383 RSC.
- W. Xia, M. Ni, C. Yao, X. Wang, D. Chen, C. Lin, X. Hu and L. Wang, Macromolecules, 2015, 48, 4403–4409 CrossRef CAS.
- D. Xu and S. L. Craig, J. Phys. Chem. Lett., 2010, 1, 1683–1686 CrossRef CAS PubMed.
- Z. Zhang, Q. Lv, X. Gao, L. Chen, Y. Cao, S. Yu, C. He and X. Chen, ACS Appl. Mater. Interfaces, 2015, 7, 8404–8411 CAS.
- X. Ji, J. Chen, X. Chi and F. Huang, ACS Macro Lett., 2014, 3, 110–113 CrossRef CAS.
- X. Yan, F. Wang, B. Zheng and F. Huang, Chem. Soc. Rev., 2012, 41, 6042–6065 RSC.
- G. Yu, X. Yan, C. Hana and F. Huang, Chem. Soc. Rev., 2013, 42, 6697–6722 RSC.
- X. Ji, K. Jie, S. C. Zimmerman and F. Huang, Polym. Chem., 2015, 6, 1912–1917 RSC.
- F. Zeng, Y. She and C. Chen, Soft Matter, 2013, 9, 4875–4882 RSC.
- A. I. Carrillo, J. Bachl, J. Mayr, P. J. Plaza-González, J. M. Cátala-Civera and D. Díaz, Phys. Chem. Chem. Phys., 2015, 17, 6212–6216 RSC.
- K. Murata, M. Aoki, T. Nishi, A. Ikeda and S. Shinkai, J. Chem. Soc., Chem. Commun., 1991, 1715–1718 RSC.
- X. Yan, D. Xu, X. Chi, J. Chen, S. Dong, X. Ding, Y. Yu and F. Huang, Adv. Mater., 2012, 24, 362–369 CrossRef CAS PubMed.
- J. Li, X. Li, Z. Zhou, X. Ni and K. W. Leong, Macromolecules, 2001, 34, 7236–7237 CrossRef CAS.
- Y. Zheng, A. Hashidzume, Y. Takashima, H. Yamaguchi and A. Harada, Nat. Commun., 2012, 3, 1841 Search PubMed.
- H. Yang, Y. Tan and Y. Wang, Soft Matter, 2009, 5, 3511–3516 RSC.
- I. Hwang, W. S. Jeon, H.-J. Kim, D. Kim, H. Kim, N. Selvapalam, N. Fujita, S. Shinkai and K. Kim, Angew. Chem., Int. Ed., 2007, 46, 210–213 CrossRef CAS PubMed.
- B. Xing, M.-F. Choi and B. Xu, Chem. Commun., 2002, 362–363 RSC.
- T. Becker, C. Yong Goh, F. Jones, M. J. McIldowie, M. Mocerino and M. I. Ogden, Chem. Commun., 2008, 3900–3902 RSC.
- J. Zhou, X. Chen and Y. Zheng, Chem. Commun., 2007, 5200–5202 RSC.
- Y. Zheng, S. Ran, Y. Hua and X. Liu, Chem. Commun., 2009, 1121–1123 RSC.
- J. Zhang, D. Guo, L. Wang, Z. Wang and Y. Liu, Soft Matter, 2011, 7, 1756–1762 RSC.
- T. Mecc, G. M. L. Messina, G. Marletta and F. Cunsolo, Chem. Commun., 2013, 49, 2530–2532 RSC.
- X. Cai, Y. Wu, J. Liu, L. Wang and Y. Fang, Soft Matter, 2013, 9, 5807–5814 RSC.
- H. Yang, S. Zhang, K. Liu and Y. Fang, RSC Adv., 2016, 6, 109969–109977 RSC.
- M. A. Rao, Rheology of fluid and semisolid foods, 1999, vol. 105–108, pp. 244–255 Search PubMed.
- M. Ma, B. Fang, Y. Lu, M. Tian, X. Qiu, G. Li, H. Jin, Y. He, C. Huang, Y. Liu, L. Wang and K. Li, J. Dispersion Sci. Technol., 2015, 1076–1082 Search PubMed.
- M. Xue, K. Liu, J. Peng, Q. Zhang and Y. Fang, J. Colloid Interface Sci., 2008, 327, 94–101 CrossRef CAS PubMed.
- J. Peng, K. Liu, X. Liu, H. Xia, J. Liu and Y. Fang, New J. Chem., 2008, 32, 2218–2224 RSC.
- K. Iwamoto and S. Shinkai, J. Org. Chem., 1992, 57, 7066–7073 CrossRef CAS.
- W. Wang, S. Gong, Y. Chen and J. Ma, New J. Chem., 2005, 29, 1390–1392 RSC.
- E. M. Collins, M. A. Mckervey, E. Madigan, M. B. Moran, M. Owens, G. Ferguson and S. J. Harris, J. Chem. Soc., Perkin Trans. 1, 1991, 3137–3142 RSC.
Footnote |
† Electronic supplementary information (ESI) available: SEM of solvent polar dependence of the gel-networks of 1/kerosene and rheological properties of an example gel system of 1/kerosene. See DOI: 10.1039/c7ra02076g |
|
This journal is © The Royal Society of Chemistry 2017 |