DOI:
10.1039/C7RA01146F
(Paper)
RSC Adv., 2017,
7, 18861-18866
A spiro-centered thermopolymerizable fluorinated macromonomer: synthesis and conversion to the high performance polymer†
Received
25th January 2017
, Accepted 22nd March 2017
First published on 28th March 2017
Abstract
A new spiro-centered thermopolymerizable fluorinated macromonomer is reported here. This four-functional macromonomer is synthesized by a convergent strategy, and shows a low melting point (93 °C) and good solubility in the common organic solvents. When heated to a high temperature, the macromonomer converts into a cross-linked network (PD1), which exhibits a Tg of near 316 °C and a 5% weigh loss at a temperature of 417 °C in N2. PD1 also shows a low dielectric constant (Dk = 2.58 at 30 MHz) and high hydrophobicity (a water contact angle of θ = 98°), as well as low water uptake (about 0.45%, kept in boiling water for 72 h). These results suggest that the macromonomer has a potential application in the production of the high performance polymers, in particular, it is very suitable for encapsulation resins or the coatings utilized in the microelectronics industry.
Introduction
High performance polymers have attracted much attention in past decades because of their excellent properties and wide applications in many areas of industry.1–10 These materials usually show high thermostability, good mechanical properties, excellent insulating behavior and moisture resistance.11–17 Therefore, they have played very important roles in the materials utilized in aerospace, electrical and microelectronics industries. However, most of the polymers show unsatisfactory processability, which needs to be improved. For example, a widely used polyimide with excellent thermostability and high strength, commercially known as Kapton, shows low solubility in the common organic solvents.18–20 Moreover, PEEKs, a class of engineering plastics having high Tg and high resistance to UV radiation exhibit high melting point and high melting viscosity.21,22 Hence, how to realize a balance between the processability and the high performance of the polymers is a challenging task.
In recent years, we are interested in developing the new materials with both high performance and good process-bility.12,23–26 It was found in our previous investigations that polymers or monomers with trifluorovinyl ether (TFVE) groups were more suitable for the preparation of the materials having both satisfactory physical properties and good processability. Those polymers or monomers usually showed good solubility in common organic solvents, as well as low melting point. Upon heating, they easily converted to the crosslinked networks containing perfluorocyclobutane (PFCB) structure via thermally induced [2 + 2] cyclodimerization of TFVE groups.27,28 The crosslinked networks exhibited good properties including high thermostability, good mechanical strength, low surface energy and dielectric constants, as well as highly visible transmittance.29–31 It is noted that spiro-centered polymers usually show high thermostability and good solubility owing to their rigid skeleton and distorted spiro-moiety.32,33 However, the dielectric properties of the spiro-centered polymers need to be improved.32,33 Based on the good dielectric properties of fluoropolymers, we have designed and synthesized a new functional monomer with spirophenol (S-1) as a core and thermocrosslinkable TFVE groups as the arms. The chemical structure of the monomer is depicted in Scheme 1. The monomer showed low melting point (93 °C) and good solubility in the common organic solvents. When heated at high temperature, the monomer transformed into a cross-linked network, which exhibited high Tg and low dielectric constant, as well as low water uptake. Those results indicate that the monomer can well realize the balance between the performance and processability of the polymers. Based on its good dielectric properties and thermostability, this monomer is very suitable as the encapsulation resin used in microelectronic industry, in which the high performance resins for sealing the devices are required. In particular, the resins with low dielectric constant, good processability, high thermostability and moisture-resistance are desirable.
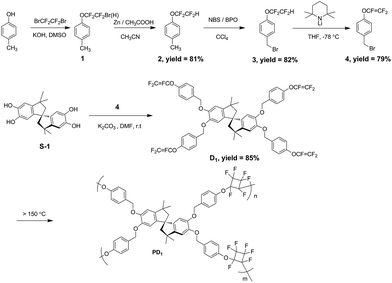 |
| Scheme 1 Procedure for the synthesis and conversion of the new monomer D1. | |
Results and discussion
Synthesis and characterization
The procedure for the synthesis of the new monomer is shown in Scheme 1. By using a simple Williamson reaction between a spirophenol (S-1) and 1-(bromomethyl)-4-(trifluoroethenyloxy)benzene (compound 4), the new monomer D1 was prepared in a high yield. Initially, we tried to synthesize compound 4 by using a general route. e.g., firstly, brominating compound 1 produced 1-(bromomethyl)-4-(bromotrifluoroethyloxy)benzene, which was then treated with zinc to yield the target product via an elimination reaction. However, the bromomethyl (–CH2Br) group also reacted with zinc. Thus, compound 4 can not be prepared by using the conventional route. It is noted that a previous report offered a different route for the synthesis of compound 4 by using 4-bromophenol as the feedstock.34 Such a five-step procedure gave the product in low overall yield. In particular, dangerous tert-butyl lithium was used for the preparation of an intermediate, suggesting the route can not provide the product in large scale.34 Fortunately, when we tried to employ a new procedure proposed by our group,35 using lithium 2,2,6,6-tetramethylpiperidine (LTMP) as base to transform –OCF2CF2 H into –OCF
CF2, compound 4 was obtained in an overall yield of about 52%, starting from 4-methylphenol. In that case, –CH2Br group in 3 did not reacted with LTMP. It must be pointed out that the reaction of spirophenol S-1 with compound 4 should be carried out in the presence of weaker bases at lower temperature because trifluorovinyl ether groups are sensitive to bases. We found that K2CO3 was a better base when the reaction was run at room temperature.
The new monomer D1 was soluble in common organic solvents such as toluene, ethyl acetate and chloroform. When heated to near 100 °C, the monomer melted and changed to a transparent liquid with very low viscosity, suggesting good processability of D1.
The chemical structure of D1 was characterized by its 1H NMR, 19F NMR and 13C NMR spectra, as well as by its elemental analysis results. The detailed data are listed in ESI.† As depicted in the ESI,† the signals, attributed to the H of –CH2O– groups, appear at 4.83–5.09 ppm in the 1H NMR spectrum. Furthermore, the 19F NMR signals of D1 appearing at −119.35 to −134.15 ppm, are ascribed to the –OCF
CF2 groups. Thus, all detected data are accordance with the proposed structure.
Thermo-crosslinking reaction
Upon heating, –OCF
CF2 groups have a tendency to form a crosslinking network via a [2 + 2] cyclodimerization. In our case, the cyclodimerization process was monitored by differential scanning calorimetry (DSC), and the results are shown in Fig. 1. As depicted in Fig. 1, D1 exhibits a melting point at 93 °C, and a curing onset temperature of about 150 °C. With increasing temperature, the monomer gives a wide exothermic peak at a range of temperatures from 200 to 300 °C, implying that D1 has wide process windows. At second scan, no obvious exothermic peak is observed, meaning that the monomer has been completely converted.
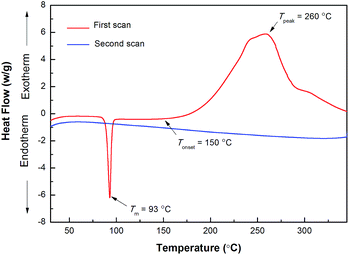 |
| Fig. 1 DSC traces of D1 at a heating rate of 10 °C min−1 in N2 atmosphere. | |
The thermo-crossing degree of D1 was characterized by FT-IR spectroscopy. Fig. 2 depicts the difference of FT-IR spectra between D1 and thermo-crosslinked D1 (PD1). As can be seen form Fig. 2, a characteristic peak at 1835 cm−1 for TFVE disappears and a characteristic peak at 960 cm−1 for perfluorocyclobutane (PFCB) appears in PD1.
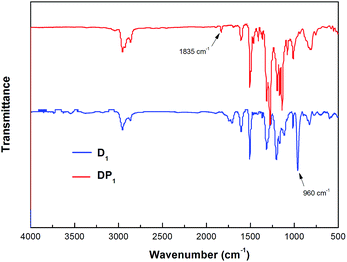 |
| Fig. 2 FT-IR spectra of D1 before (up) and after (down) thermo-crosslinking. | |
Thermostability and dielectric properties
In many cases, thermo-crosslinking can effectively improve the heat-resistance of polymers. In our work, the thermostability of thermo-crosslinked D1 (PD1) was investigated by thermal gravimetric analysis (TGA), and the results are shown in Fig. 3. As exhibited in Fig. 3, PD1 has 5 wt% loss temperature of 417 °C and a weight residue of 53% at 1000 °C (in N2), respectively. These data are higher than many commercial materials such as epoxy and phenolic resins.36,37 It is noted that coefficient of thermal expansion (CTE) reflects the dimensional stability of the materials upon the temperature changes. Fig. 4 depicts the CTE curves of PD1 at a range of temperatures from 30 to 350 °C. It is seen that PD1 has an average linear CTE of 59.5 ppm °C−1 varying from 30 to 150 °C, whereas such a value increases to 91.2 ppm °C−1 when the temperature is up to 150 °C. Fig. 4 also gives a Tg value of PD1 (near 316 °C), which is obviously higher than those of most phenolic and epoxy resins.36,37
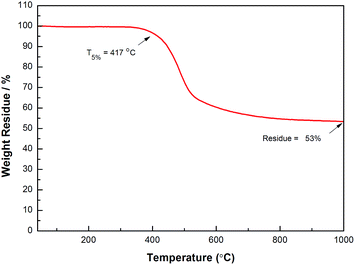 |
| Fig. 3 TGA curves of PD1. | |
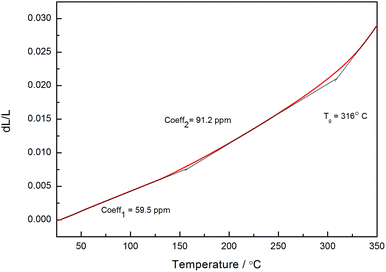 |
| Fig. 4 Relative length change (dL/L) of PD1 versus temperature (T). | |
The dielectric constant and dielectric loss of PD1 were measured according to standard capacitance method,25 and the results are illustrated in Fig. 5. As can be seen from Fig. 5, the average dielectric constant of PD1 is less than 2.58, along with dissipation factor of below 0.01 varying from 1 to 30 Hz. Such a low dielectric constant is attributed to the incorporation of C–F bond, which decreases the polarity of the molecule. On the other hand, the formation of perfluorocyclobutane units during the curing of D1 can lower the crosslinking density, also resulting in the decreasing of dielectric constant.12 These results are comparable with that of commercial low-k materials, such as SILK (2.65–2.75),38 poly(aryl ether) (2.7–3.0),39 benzoxazine resins (2.81),40 and benzocyclobutene (BCB)-based polymers (2.65).41
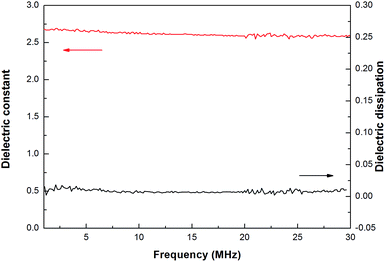 |
| Fig. 5 Dielectric constant and dissipation factor of PD1 at different frequency at room temperature. | |
Water uptake and film uniformity and mechanical properties
It is very important to have low water absorption for the application of the materials in the microelectronic industry. The water uptake test of PD1 was carried out in boiling water. After kept at the water for 72 h, PD1 showed water absorption of about 0.45%. Such result is better than that of Kapton, a famous commercial polyimide used as the insulating coating in industry, which shows water absorption of 0.74%.42 In order to investigate the reason why PD1 showed low water uptake, hydrophobicity of a PD1 film also was surveyed by the water contact angle test. To obtain a film for the test, a solution of D1 in 1,2,4-trimethylbenzene was heated at 180 °C for 4 h to give a pre-polymer. Spin-coating a solution of the pre-polymer on a silicon wafer gave a smooth and transparent film. The wafer was then moved to an oven, slowly heated to 220 °C and maintained at the temperature for 4 h in N2. Thus, a full cued PD1 film was obtained. On the surface of the film, the water contact angle was measured as 98.6° (Fig. 6), indicating that the PD1 film has good hydrophobicity. Thus, the low water uptake of PD1 is attributed to the high hydrophobicity of the polymer.
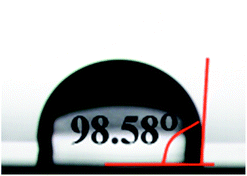 |
| Fig. 6 Contact angle of water on a PD1 film. | |
The film uniformity of PD1 was characterized by means of atomic force microscopy (AFM). Fig. 7 exhibits the AFM image of the surface of a PD1 film on a silicon wafer. In a 3.0 × 3.0 μm area, the average surface roughness (Ra) of PD1 film is about 0.34 nm. Such a low Ra value suggests that PD1 film have good uniformity.
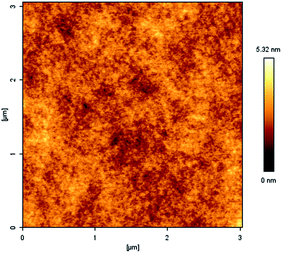 |
| Fig. 7 AFM image of PD1. | |
The nano indenter/scratch system was used to measure the mechanical properties of PD1, (as shown in Fig. 8 and Tables S1, S2†). The results indicated that a PD1 film had an average hardness of 0.41 GPa, a Young's modulus of 14.82 GPa and a bonding strength to the silicon wafer of 0.23 GPa, respectively. These data exhibit that PD1 is suitable as a high performance coating utilized in electronic/electrical devices.
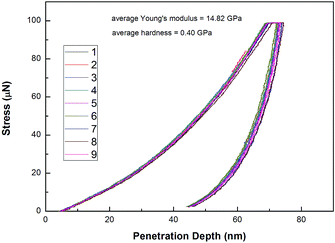 |
| Fig. 8 The load-displacement curves of nanoindentation tests for a PD1 film. | |
Conclusions
In summary, we have successfully synthesized a new tetra-functional macromonomer by using a facile procedure. This macromonomer shows low melting point (93 °C) and good solubility in the common organic solvents. The monomer can be easily thermally transformed into a cross-linked network, showing high Tg, low dielectric constant, good film uniformity and low water absorption. These good properties suggest the monomer is very suitable as the encapsulation resins or the coatings utilized in microelectronic industry.
Acknowledgements
Financial supports from Ministry of Science and Technology of China (2015CB931900) and the Natural Science Foundation of China (NSFC No. 21574146 and No. 21504103) and the Science and Technology Commission of Shanghai Municipality (15ZR1449200 and 16JC1403800) are gratefully acknowledged. The authors are also grateful to the Strategic Priority Research Program of the Chinese Academy of Sciences (Grant XDB 20020000).
Notes and references
- F. Herold and A. Schneller, High-Performance Polymers, Adv. Mater., 1992, 4, 143–152 CrossRef CAS.
- X. Guo, R. P. Ortiz, Y. Zheng, M.-G. Kim, S. Zhang, Y. Hu, G. Lu, A. Facchetti and T. J. Marks, Thieno[3,4-c]pyrrole-4,6-dione-based Polymer Semiconductors: Toward High-Performance, Air-Stable Organic Thin-Film Transistors, J. Am. Chem. Soc., 2011, 133, 13685–13697 CrossRef CAS PubMed.
- J. K. Fink, High performance polymers, William Andrew Inc., New York, 2008 Search PubMed.
- M. G. Dhara and S. Banerjee, Fluorinated High-Performance Polymers: poly(arylene ether)s and Aromatic Polyimides Containing Trifluoromethyl Groups, Prog. Polym. Sci., 2010, 35, 1022–1077 CrossRef CAS.
- T. M. Swager, Iptycenes in the Design of High Performance Polymers, Acc. Chem. Res., 1994, 41, 1181–1189 CrossRef PubMed.
- R. B. Seymour, Advances in High-Performance Polymers, in The Effects of Hostile Environments on Coatings and Plastics, ACS Symp Ser, 229, ed. D. P. Garner1 and G. Allan Stahl, American Chemical Society, Washington, DC, 1983, pp. 87–98 Search PubMed.
- D. K. Dei, B. R. Lund, J. Wu, D. Simon, T. Ware, W. E. Voit, D. MacFarlane, S. M. Liff and D. W. Smith, High Performance and Multipurpose Triarylamine-Enchained Semifluorinated Polymers, ACS Macro Lett., 1989, 2, 35–39 CrossRef.
- T. Lei, Y. Cao, Y. Fan, C.-J. Liu, S.-C. Yuan and J. Pei, High-Performance Air-Stable Organic Field-Effect Transistors: Isoindigo-Based Conjugated Polymers, J. Am. Chem. Soc., 1989, 133, 6099–6101 CrossRef PubMed.
- H. Hatakeyama, S. Hirose and T. Hatakeyama, High-Performance Polymers from Lignin Degradation Products, in Lignin: properties and materials, ACS Symp Ser, 397, ed. W. G. Glasser and S. Sarkane, American Chemical Society, Washington, DC, 1989, pp. 205–218 Search PubMed.
- T. Shimomura and T. Namba, Preparation and Application of High-Performance Superabsorbent Polymers, in Superabsorbent Polymers, ACS Symp Ser, 573, ed. F. L. Buchholz1 and N. A. Peppas, American Chemical Society, Washington, DC, 1994, pp. 112–127 Search PubMed.
- H.-C. Liou, P. S. Ho and A. McKerrow, The effect of crosslinking on aromatic ether polymers, J. Polym. Sci., Part B: Polym. Phys., 1998, 36, 1383–1392 CrossRef CAS.
- C. Yuan, K. Jin, K. Li, S. Diao, J. Tong and Q. Fang, Non-Porous Low-k Dielectric Films Based on a New Structural Amorphous Fluoropolymer, Adv. Mater., 2013, 25, 4875–4878 CrossRef CAS PubMed.
- Y. Luo, J. Sun, K. Jin, J. Wang, G. Huang and Q. Fang, Propargyl ether-functionalized poly(m-phenylene): a new precursor for the preparation of polymers with high modulus and high Tg, RSC Adv., 2015, 5, 23009–23014 RSC.
- M. Pantouvaki, C. Huffman, L. Zhao, N. Heylen, Y. Ono, M. Nakajima, K. Nakatani, G. P. Beyer and M. R. Baklanov, Jpn. Advanced Organic Polymer for the Aggressive Scaling of Low-k Materials, J. Appl. Phys., 2011, 50, 584–587 Search PubMed.
- D. Shamiryan, T. Abell, F. Iacopi and K. Maex, Low-k dielectric materials, Mater. Today, 2004, 7, 34–39 CrossRef CAS.
- R. Souzy, B. Ameduri and B. Boutevin, Synthesis and co-polymerization of monofluoro, difluoro, trifluorostyrene and ((trifluorovinyl)oxy)benzene, Prog. Polym. Sci., 2004, 29, 75–106 CrossRef CAS.
- C. A. Corley, A. J. Guenthner, C. M. Sahagun and K. R. Lamison, Di(cyanate Ester) Networks Based on Alternative Fluorinated Bisphenols with Extremely Low Water Uptake, ACS Macro Lett., 2014, 3, 105–109 CrossRef CAS.
- J. M. García, F. C. García, F. Serna and J. L. de la Peña, High-performance aromatic polyamides, Prog. Polym. Sci., 2010, 35, 623–686 CrossRef.
- D.-J. Liaw, K.-L. Wang, Y.-C. Huang, K.-R. Lee, J.-Y. Lai and C.-S. Ha, Advanced polyimide materials: Syntheses, physical properties and applications, Prog. Polym. Sci., 2012, 37, 907–974 CrossRef CAS.
- M. G. Dhara and S. Banerjee, Fluorinated high-performance polymers: Poly(arylene ether)s and aromatic polyimides containing trifluoromethyl groups, Prog. Polym. Sci., 2010, 35, 1022–1077 CrossRef CAS.
- H. X. Guyen and H. Ishida, Poly(aryl-ether-ether-ketone) and its advanced composites: a review, Polym. Compos., 1987, 8, 57–73 CrossRef.
- S. Pramanik and K. K. Kar, Functionalized poly(ether ether ketone): improved mechanical property and acellular bioactivity, J. Appl. Polym. Sci., 2012, 123, 1100–1111 CrossRef CAS.
- J. Wang, K. Li, C. Yuan, K. Jin, S. Tian, J. Sun and Q. Fang, Variable Polymer Properties Driven by Substituent Groups: Investigation on a Trifluorovinylether- Functionalized Polyfluorene at the C-9 Position, Macromol. Chem. Phys., 2015, 216, 742–748 CrossRef CAS.
- C. Yuan, J. Wang, K. Jin, S. Diao, J. Sun, J. Tong and Q. Fang, Postpolymerization of Functional Organosiloxanes: An Efficient Strategy for Preparation of Low-k Material with Enhanced Thermostability and Mechanical Properties, Macromolecules, 2014, 47, 6311–6315 CrossRef CAS.
- F. He, Y. Gao, K. Jin, J. Wang, J. Sun and Q. Fang, Conversion of a Biorenewable Plant Oil (Anethole) to a New Fluoropolymer with Both Low Dielectric Constant and Low Water Uptake, ACS Sustainable Chem. Eng., 2016, 4, 4451–4456 CrossRef CAS.
- J. Zhou, L. Fang, J. Wang, J. Sun, K. Jin and Q. Fang, Post-functionalization of novolac resins by introducing thermo-crosslinkable –OCF
CF2 groups as the side chains: a new strategy for production of thermosetting polymers without releasing volatiles, Polym. Chem., 2016, 7, 4313–4316 RSC. - S. T. Iacono, S. M. Budy, J. Jin and D. W. Smith Jr, Science and technology of perfluorocyclobutyl aryl ether polymers, J. Polym. Sci., Part A: Polym. Chem., 2007, 45, 5705–5721 CrossRef CAS.
- D. A. Babb, B. R. Ezzell, K. S. Clement, W. F. Richey and A. P. Kennedy, Perfluorocyclobutane aromatic ether polymers, J. Polym. Sci., Part A: Polym. Chem., 1993, 31, 3465–3477 CrossRef CAS.
- M. Mujkic, S. T. Iacono, A. R. Neilson and D. W. Smith Jr, Recent Optical Applications of Perfluorocyclobutyl Aryl Ether Polymers, Macromol. Symp., 2009, 283–284, 326–335 CrossRef CAS.
- R. Verma, S. Creager, J. Ballato and D. W. Smith Jr, Optimized statically non-wetting hydrophobic electrospun surface of perfluorocyclobutyl aryl ether polymer, Polym. Int., 2013, 62, 1152–1158 CrossRef CAS.
- H. Pu, Fluoropolymers for Proton Exchange Membranes, in Polymers for PEM Fuel Cells, John Wiley & Sons, Inc., 2014, pp. 50–101 Search PubMed.
- N. K. Sini, M. Azechi and T. Endo, Synthesis and Properties of Spiro-Centered Benzoxazines, Macromolecules, 2015, 48, 7466–7472 CrossRef.
- C. R. Mason, L. Maynard-Atem, K. W. J. Heard, B. Satilmis, P. M. Budd, K. Friess, M. Lanc, P. Bernardo, G. Clarizia and J. C. Jansen, Enhancement of CO2 Affinity in a Polymer of Intrinsic Microporosity by Amine Modification, Macromolecules, 2014, 47, 1021–1029 CrossRef CAS PubMed.
- B. K. Spraul, S. Suresh, J. Jin and D. W. Smith Jr, Synthesis and Electronic Factors in Thermal Cyclodimerization of Functionalized Aromatic Trifluorovinyl Ethers, J. Am. Chem. Soc., 2006, 128, 7055–7064 CrossRef CAS PubMed.
- Y. Luo, K. Jin, C. He, J. Wang, J. Sun, F. He, J. Zhou, Y. Wang and Q. Fang, An Intrinsically Microporous Network Polymer with Good Dielectric Properties at High Frequency, Macromolecules, 2016, 49, 7314–7321 CrossRef CAS.
- H. J. Hwang, S. W. Hsu, C. L. Chung and C.-S. Wang, Low dielectric epoxy resins from dicyclopentadiene-containing poly(phenylene oxide) novolac cured with dicyclopentadiene containing epoxy, React. Funct. Polym., 2008, 68, 1185–1193 CrossRef CAS.
- A. Knop and L. A. Pilato, Phenolic Resins: Chemistry, Applications and Performance, Springer-Verlag, Berlin Heidelberg, 1985 Search PubMed.
- S. J. Martin, J. P. Godschalx, M. E. Mills, E. O. Shaffer II and P. H. Townsend, Development of a low-dielectric-constant polymer for the fabrication of integrated circuit interconnect, Adv. Mater., 2000, 12, 1769–1778 CrossRef CAS.
- C. Wang, C. Xu, Q. Li, W. Chen and X. Zhao, Synthesis of new fluorene-based poly(aryl ether) containing pendant tert-butyl groups for low dielectric materials, Colloid Polym. Sci., 2015, 293, 313–318 CAS.
- J. Wu, Y. Xi, G. T. McCandless, Y. Xie, R. Menon, Y. Patel, D. J. Yang, S. T. Iacono and B. M. Novak, Synthesis and Characterization of Partially Fluorinated Polybenzoxazine Resins Utilizing Octafluorocyclopentene as a Versatile Building Block, Macromolecules, 2015, 48, 6087–6095 CrossRef CAS.
- J. Tong, S. Diao, K. Jin, C. Yuan, J. Wang, J. Sun and Q. Fang, Benzocyclobutene-functionalized poly(m-phenylene): a novel polymer with low dielectric constant and high thermostability, Polymer, 2014, 55, 3628–3633 CrossRef CAS.
- F. Bellucci and L. Nicodemo, Water transport in organic coatings, Corros. Sci., 1993, 49, 235–247 CrossRef CAS.
Footnote |
† Electronic supplementary information (ESI) available: Experimental routes, NMR spectra of the monomers and polymers. See DOI: 10.1039/c7ra01146f |
|
This journal is © The Royal Society of Chemistry 2017 |