DOI:
10.1039/C7RA00830A
(Paper)
RSC Adv., 2017,
7, 19385-19390
Fast microwave-assisted conjugation of magnetic nanoparticles with carboxylates of biological interest†
Received
19th January 2017
, Accepted 27th March 2017
First published on 31st March 2017
Abstract
A new technique of surface modification of nanoparticles (Fe3O4-NP) with carboxylates of biological interest has been developed with a microwave-assisted heating method. This two-step technique reduces the reaction time to 15 min (for each step) and increases the number of substituents to 2300 molecules compared to traditional methods with a maximum of 440 molecules.
Introduction
In the search of new functional materials, it is important to consider magnetic nanoparticles (Fe3O4-NP) as a valuable support for biological applications, such as magnetic fluid hyperthermia applications, magnetic resonance imaging (MRI), immunoassays, labelling for cells catalysis, and targeted drug delivery.
In most cases Fe3O4-NP are synthesized in the presence of surfactants, like oleic acid, to prevent aggregation among them. In order to improve the stability of the nanoparticles, the ligand molecules on the surface can be exchanged by others that can possibly provide new properties or functionality to the particles, with the incoming ligand molecule binding more strongly to the inorganic nanoparticle surface. Nanoparticles with better stability are those with strong covalent interactions with their ligands.1–4
An excellent proposal as a bifunctional linker is 3-aminopropyltriethoxysilane (APTES) which is able to make covalent binding at both sites, the first with inorganic nanoparticle surface and the second with organic molecules via amidation.5,6 It is important to conjugate the largest number of molecules on Fe3O4-NP surface to improve efficiency of materials. For that reason we propose a fast method assisted by microwave heating. Also, in this work we tested different carboxylates, namely, folic acid (FA), methotrexate (MTX), pemetrexed (PMX), raltitrexed (RTX), glutathione (GSH) and dimercaptosuccinic acid (DMSA) (Scheme 1), with biological applications like drugs for treatment and prevention of cancer and heavy metals chelation.2,5,7–10
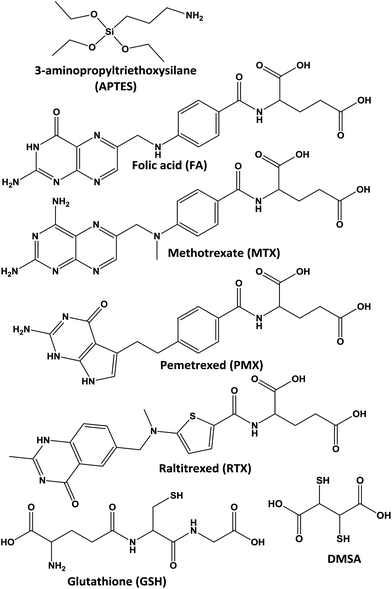 |
| Scheme 1 Linker and biological molecules used in this work to functionalize Fe3O4-NP. | |
In previous works,5–7,11–13 conjugation of Fe3O4-NP with APTES and similar linkers takes long reaction times and poor yields which does not allow Fe3O4-NP to be functionalized beyond 300–500 molecules per each nanoparticle (1.2 molecules per nm2).3,5 In general microwave processes are energy and time saving, reduce thermal gradient effects, thus leading to better results, and also can provide cleaner reactions.
In the present research, we exploit the potential of the microwave technique, on one hand, to improve the coupling processes with carboxylates on the Fe3O4-NP surface and, on the other hand, to reduce the reaction time from hours to few minutes.14 The amount of molecules loaded on magnetic nanoparticles was estimated by UV-Vis and TGA, and the results have been compared with those reported in the literature.
Results and discussion
Synthesis of Fe3O4-NP via thermal decomposition
Among different kinds of methodologies to synthesize Fe3O4-NP,15 the one that controls the NP size is the best option to get monodisperse Fe3O4-NP with 3 to 20 nm in diameter. The uniform shape and size distribution of Fe3O4-NP was analyzed by transmission electron microscopy (TEM) and showed an iron oxide core of about 8 nm in diameter on average. Fig. 1 shows FTIR with typical –CH2–CH2– absorption band at 2920 cm−1 and –CH2–CH3 at 2850 cm−1, C
O bond at 1590 cm−1, and Fe–O bond for magnetic nanoparticles at 630 cm−1.
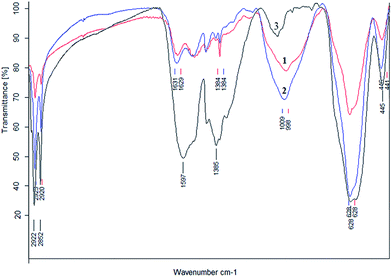 |
| Fig. 1 FTIR of Fe3O4-NP-APTES (1) traditional reaction, (2) microwave reaction and (3) Fe3O4-NP-oleic acid. | |
Functionalization of Fe3O4-NP-APTES via microwave and characterization
In the search of better hybrid nanomaterials, the key is a correct functionalization of the particle surface. As the first step to improve traditional methods it is important to choose the appropriate solvent for microwave heating, to increase temperatures and decrease reaction times. For instance, in previous works,5 the reaction is undertaken in toluene anhydride, sonicating for 4 hours at 60 °C and finally washed with EtOH. According to the bibliography, we used the same reagents proportions and tested different organic solvents to enhance the insertion of APTES on Fe3O4-NP surface. Water, EtOH and MeOH were not used because they can hydrolyze APTES. The final product was magnetically separated and washed with EtOH to remove rests of APTES in the reaction medium. From the results of these reactions gathered in Table 1 it can be inferred that toluene is the best option. Accordingly, we determined the best conditions for microwave heating at 120 °C, 2 bar, and 15 minutes (P = 400 W) with toluene as solvent. Other solvents (acetone, acetonitrile) reached higher pressures under the same reaction conditions, but were not good candidates because they are not able to promote the exchange of substituents. In other cases, solvents with higher boiling points than toluene make difficult to clean the final product. APTES coating was confirmed by FTIR (Fig. 1) with typical Si–O bond at 1000 cm−1. There are also bands at 628 and 446 cm−1 which are typical for Fe–O. By inspecting the FTIR results, obtained from 1 mg of dried product and 100 mg of KBr (in both cases), a high functionalization for the microwave-assisted process is observed.
Table 1 List of results of different solvents used for the Fe3O4-NP-APTES functionalization
Solvent |
Boiling point (°C) |
Pressure (bar) |
Results |
Acetone |
56.0 |
4 |
No reaction |
Acetonitrile |
82.0 |
3 |
No reaction |
CH2Cl2 |
39.6 |
7 |
Precipitate |
DMF |
153.0 |
0 |
No reaction |
DMSO |
189.0 |
0 |
No reaction |
Hexane |
68.0 |
2 |
Precipitate |
Toluene |
110.6 |
2 |
Precipitate |
Xylene |
137.0 |
1 |
Precipitate |
Amidation on Fe3O4-NP surface and characterization
In order to study fast covalent bonding of antifolates and other organic molecules, we prepared six different hybrid nanoparticles: Fe3O4-NP-APTES-FA, Fe3O4-NP-APTES-MTX, Fe3O4-NP-APTES-PMX, Fe3O4-NP-APTES-RTX, Fe3O4-NP-APTES-GSH, and Fe3O4-NP-APTES-DMSA. Analogously to the previous chapter, the microwave treatment and the classical heating mode for the Fe3O4-NP functionalization were compared. The free –NH2 groups present on APTES react with antifolates via activation of the carboxylic groups by the carbodiimide/N-hydroxysuccinimide method using the microwave technique. Traditional processes7,16 perform amidation reaction on the Fe3O4-NP surface at 37 °C overnight with DMSO as solvent. Here we propose a fast method, adapted from the literature,17–19 using microwave heating (P = 400 W) and MeOH/H2O as solvent at 120 °C. All experiments reached 4 bar of pressure in 15 minutes of reaction. Size distribution of different Fe3O4-NP was analyzed by TEM (Fig. 2) showing particles of 8 nm in average. For a better view, TEM complete microphotographs are shown in the ESI (Fig. S12 and S13†).
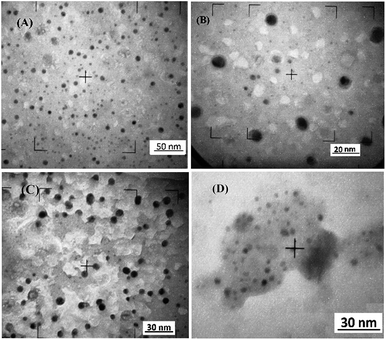 |
| Fig. 2 TEM microphotographs (A) Fe3O4-NP-oleic acid. (B) Fe3O4-NP-APTES-. (C) Fe3O4-NP-APTES-GSH. (D) Fe3O4-NP-APTES-FA. | |
FTIR shows the correct functionalization of Fe3O4-NP in all cases. The presence of C
O stretching bands at 1630 cm−1 are typical of monosubstituted amide groups, confirmed with the presence of an N–H band at 1550 cm−1; Si–O bond is still present at 1000 cm−1, and Fe–O bond at 630 cm−1 (Fig. 3). The general procedure of modification via microwave is shown in Scheme 2. The FTIR spectra performed for the free carboxylates are shown in the ESI (Fig. S1–S5†). In addition, the MS spectrum data are included in the Experimental section. These results confirm that the reaction was successfully performed.
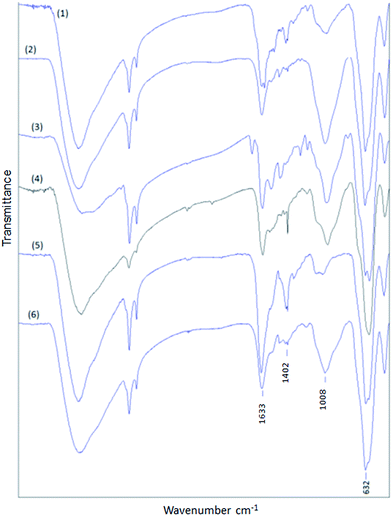 |
| Fig. 3 FTIR of Fe3O4-NP-APTES- (1) FA, (2) MTX, (3) PMX, (4) RTX, (5) GSH and (6) DMSA. | |
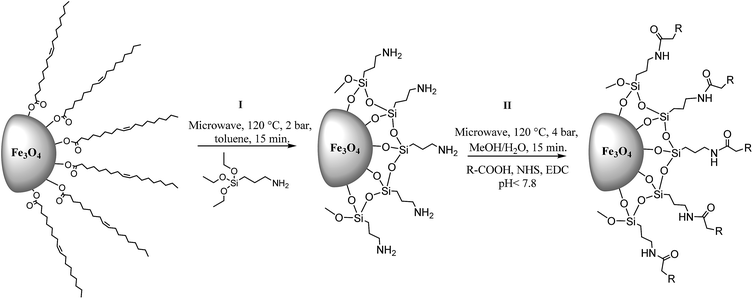 |
| Scheme 2 General procedure for Fe3O4-NP functionalization. | |
Determination of number of molecules on Fe3O4-NP surface
It is important to have a precise control on the number and orientation of the immobilized molecules on nanoparticles because carboxylates coordination occurs via APTES free amine groups. In previous works, the concentration of APTES and carboxylates (MTX, PMX and RTX) covalently bounded on the Fe3O4-NP surface was estimated by the standard addition method by 1H-NMR and UV-VIS through the residues of the reaction applying the following equations:
where 1/N refers to the number of Fe3O4-NP for each mol of Fe3O4. D is the average diameter of Fe3O4-NP in cm (provided by TEM microphotographs), ρ is Fe3O4 density (5.196 g cm−3) and mw is the molecular weight of Fe3O4 (231.53 g mol−1).20 In order to improve and clarify the number of substituents, we chose TGA method to determine the conjugation rate by measuring the mass loss due to decomposition. Experiments were performed with a constant heating rate of 10 °C min−1 from room temperature (25 °C) to 1000 °C. The mass lost from 25 °C to 100 °C has not been taken into account in the calculations, because it corresponds to the loss of water and/or solvents that may contain the sample. The TGA curves show weight loss in both cases (Fig. 4). Using weight loss percentage values it is possible to quantify the number of molecules on Fe3O4-NP surface by applying previous equations. Our main results together with bibliographic data are summarized in Table 2.
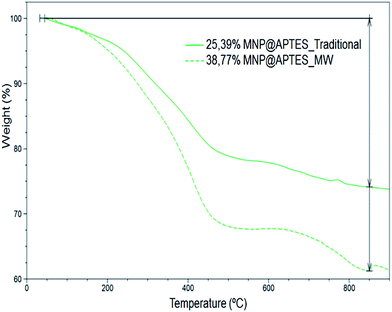 |
| Fig. 4 Thermogravimetric analysis for Fe3O4-NP-APTES. | |
Table 2 Thermogravimetric and particle size values of Fe3O4-NP functionalized by the two methods
|
Technique |
Analysis method |
θ (nm) |
Weight loss (%) |
Molecules/Fe3O4-NP |
ξ (molecules per nm3) |
F. Benyettou et al.3 N. Kohler et al.7 K. López et al.5 |
Fe3O4-NP-oleic acid |
— |
TGA |
8 |
42.01 |
2142 |
4.18 |
Fe3O4-NP-APTES |
Traditional |
TGA |
8 |
25.39 |
1284 |
2.51 |
Fe3O4-NP-APTES |
Microwave |
TGA |
8 |
38.72 |
2380 |
4.65 |
Fe3O4-NP-APTESa |
Traditional |
31P NMR titration |
10 |
— |
1200 |
1.20 |
Fe3O4-NP-APTES-MTXb |
Traditional |
Standard addition |
10 |
— |
419 |
0.42 |
Fe3O4-NP-APTES-MTX |
Microwave |
TGA |
8 |
52.69 |
2046 |
4.00 |
Fe3O4-NP-APTES-RTXc |
Traditional |
Standard addition |
6 |
— |
363 |
1.70 |
Fe3O4-NPAPTES-RTX |
Traditional |
TGA |
8 |
9.39 |
1142 |
0.54 |
Fe3O4-NPAPTES-RTX |
Microwave |
TGA |
8 |
38.55 |
1142 |
2.23 |
Fe3O4-NP-APTES-PMXc |
Traditional |
Standard addition |
6 |
— |
164 |
0.85 |
Fe3O4-NP-APTES-PMX |
Microwave |
Standard addition |
8 |
— |
2113 |
4.13 |
The microwave-assisted functionalization and conjugation reported herein clearly shows a substantial improvement in most cases over other reported methods. For APTES conjugation, the density of molecules per nm3 (ξ) is doubled, thereby increasing the number of –NH2 groups available for further amidation. The same result is observed for the amidation process, which suggest two ideas; first, a low number of linkers results in a low concentration of carboxylates and, second, the reaction conditions for the amidation reaction reported in the literature produces very low reaction yields if compared with our results.
Experimental section
Materials
All commercially available reagents: oleylamine (≥98% (primary amine)), oleic acid (≥99% (GC)), dibenzyl ether (purum, ≥98.0% (GC)), were supplied by Sigma-Aldrich. All the solvents were purchased from Scharlau (all solvents have superior to 98% purity). Ferric acetylacetonate (purum, ≥97.0% (RT)) was supplied by Fluka.
Instrumentation
Infrared (IR) was obtained on Bruker Tensor 27 instrument in solid state. Ultraviolet-visible (UV-Vis) was obtained on a Shimadzu UV-2401PC Spectrometer. Matrix-assisted laser desorption/ionization mass spectra (MALDI), was recorded with a Autoflex III MALDI TOF/TOF mass spectrometer provided with an Smart beam Laser at 200 Hz. Functionalization of Fe3O4-NP was performed on a Biotage Initiator Classic Microwave Synthesizer at 400 W. TGA were performed on a SDT Q-600 instrument.
Methods
FTIR. For IR experiments, 100 mg of anhydrous KBr were mixed with 1 mg of each product (perfectly dried) and grounded in a mortar to make a tablet.
TGA. To perform the analysis, all the samples were perfectly dried with vacuum before weighing them.
TEM. The samples of nanoparticles were dissolved in ethanol to support them in grids. The micrographics were prepared in ultrathin carbon type A400 mesh (pkg/50) grids.
Mass analysis. All the samples of nanoparticles were prepared with 1 mg of dried sample, digested for 1 hour with HCl (37%) filtered, vacuum dried and redissolved in 1 mL of Milli-Q water.
Synthesis and characterization of Fe3O4-NP
For the synthesis of Fe3O4 nanoparticles, an adapted procedure of S. Sun et al.21 was used. Fe(acac)3 (2 mmol), oleic acid (6 mmol), oleylamine (6 mmol) were mixed into 20 mL of dibenzyl ether and magnetically stirred under a flow of argon. The solution was heated firstly at 200 °C for 1 h and then at 300 °C for 2 h with vigorous magnetic stirring with reflux under Ar atmosphere. The solution was cooled at room temperature. Nanoparticles were precipitated by adding 25 mL of ethanol to the mixture. The precipitated was collected by centrifugation at 8000 rpm, washed with ethanol (3 mL × 15 mL) and redispersed in 25 mL of hexane or acetonitrile (depending on the type of reaction that will take place later), to a final concentration of 0.025 mmol mL−1. FTIR (KBr): 3406, 2921, 2851, 1591, 1384, and 632 cm−1.
Functionalization of Fe3O4-NP with APTES via microwave
For APTES functionalization procedure has been adapted from Zhang et al.7,11 to microwave reactor. 0.1 mL of APTES was added to 1.7 mL of Fe3O4-NP-oleic acid colloidal suspension in a 5 mL flask with 3 mL of toluene and hermetically closed. The mixture was heated in the microwave reactor under magnetic stirring for 15 min at 120 °C and 2 bar. Fe3O4-NP-APTES was magnetically precipitated and washed with EtOH (3× 5 mL) and finally dispersed in MeOH for the next step. FTIR (KBr): 3384, 2920, 2651, 1629, 1384, 998 and 628 cm−1.
Covalent conjugation via microwave: amidation reaction
Amidation reaction procedure has been adapted from bibliography3,11,22,23 to microwave reactor. 0.025 mmol of carboxylic acid (FA = 11.04 mg, MTX = 11.36 mg, PMX = 10.69, RTX = 11.46 mg, GSH = 7.68 mg, DMSA = 4.56 mg) is dissolved in distillated water. Moreover 34.8 mg of EDC and 5.81 mg of NHS was dissolved in 1 mL of distillated water, both dissolutions were mixed and pH was adjusted to 8, finally Fe3O4-NP-APTES dispersed in MeOH is added to the mixture, hermetically closed and heated in the microwave reactor under magnetic stirring for 15 min at 120 °C and 2 bar. Fe3O4-NP-APTES-R was magnetically precipitated and washed with distillated H2O (3 × 5 mL). To preserve the product, Fe3O4-NP-APTES-R was dissolved on a NH4OH solution of 1%.
FTIR (KBr): Fe3O4-NP-APTES-FA. 3385, 2921, 2852, 1631, 1385, 993, 630, 590 and 445 cm−1. Fe3O4-NP-APTES-MTX: 3385, 2921, 2852, 1625, 1555, 1446, 1384, 1199, 990, 590 and 442 cm−1. Fe3O4-NP-APTES-PMX: 3380, 2921, 2851, 1729, 1628, 1546, 1459, 1260, 1011, 631 and 591 cm−1. Fe3O4-NP-APTES-RTX: 3385, 2912, 2854, 1626, 1558, 1448, 1384, 997, 596 and 444 cm−1. Fe3O4-NP-APTES-GHS: 3419, 2922, 2852, 1635, 1556, 1385, 998, 59 and 443 cm−1. Fe3O4-NP-APTES-DMSA: 3385, 2921, 2852, 1631, 1385, 993, 630, 590 and 445 cm−1.
MALDI. APTES-FA: found m/z 624.844 [M], [C25H33N8NaO8Si] requires 624.209. APTES-MTX (+): found m/z 701.501 [M]+, [C25H33FeN9Na2O7Si+] requires 701.502. APTES-PMX: found m/z 475.342 [M]+, [C20H25N6Na2O5+] requires 475.168. APTES-RTX: found m/z 440.950 [M]−, [C21H21N4O5S−] requires 441.123. APTES-GSH: found m/z 510.880 [M], [C15H31FeN4O8SSi] requires 511.098. APTES-DMSA found m/z 383.967 [M], [C9H18FeNO6S2Si] requires 383.968.
Conclusions
In summary, we have developed a new and fast microwave-assisted technique for the functionalization of Fe3O4-NP with a high yield of conjugation and short reaction times, and demonstrated that the grafting rate was increased by using the microwave technique. A significant difference was encountered when comparing the nanoparticles functionalized via microwave with those obtained in a traditional way.
The number of molecules on Fe3O4-NP surface has been determined by thermogravimetric analysis and compared with standard addition method, the former showing better results. All the carboxylates used in this work showed similar results for the amidation reaction.
Acknowledgements
This work was financially supported by Ministerio de Economía y Competitividad ref. CTQ2014-57393-C2-1-P M. Susana Gutiérrez acknowledges her Ph.D. scholarship from CONACyT (Consejo Nacional de Ciencia y Tecnología de México).
Notes and references
- Y.-J. Liang, Y. Zhang, Z. Guo, J. Xie, T. Bai, J. Zou and N. Gu, Chem.–Eur. J., 2016, 22, 1–10 CrossRef CAS.
- Y. Pan, M. J. C. Long, X. Li, J. Shi, L. Hedstrom and B. Xu, Chem. Sci., 2011, 2, 945–948 RSC.
- F. Benyettou, E. Guenin, Y. Lalatonne and L. Motte, Nanotechnology, 2011, 22, 55102 CrossRef CAS PubMed.
- R. A. Sperling and W. J. Parak, Philos. Trans. R. Soc., A, 2010, 368, 1333–1383 CrossRef CAS PubMed.
- K. A. López, M. N. Piña, R. Alemany, O. Vögler, F. Barceló and J. Morey, RSC Adv., 2014, 4, 19196 RSC.
- M. Ma, Y. Zhang and W. Yu, Colloids Surf., A, 2003, 212, 219–226 CrossRef CAS.
- N. Kohler, C. Sun, J. Wang and M. Zhang, Langmuir, 2005, 21, 8858–8864 CrossRef CAS PubMed.
- C. Chen, J. Ke, X. E. Zhou, W. Yi, J. S. Brunzelle, J. Li, E.-L. Yong, H. E. Xu and K. Melcher, Nature, 2013, 500, 486–489 CrossRef CAS PubMed.
- H.-H. S. Chow, I. A. Hakim, D. R. Vining, J. A. Crowell, M. E. Tome, J. Ranger-Moore, C. A. Cordova, D. M. Mikhael, M. M. Briehl and D. S. Alberts, Cancer Epidemiol., Biomarkers Prev., 2007, 16, 1662–1666 CAS.
- P.-J. Chen, S.-H. Hu, C.-S. Hsiao, Y.-Y. Chen, D.-M. Liu and S.-Y. Chen, J. Mater. Chem., 2011, 21, 2535 RSC.
- K. A. López, M. N. Piña and J. Morey, Sens. Actuators, B, 2013, 181, 267–273 CrossRef.
- A. M. Demin, V. P. Krasnov and V. N. Charushin, Mendeleev Commun., 2013, 23, 14–16 CrossRef CAS.
- M. De, P. S. Ghosh and V. M. Rotello, Adv. Mater., 2008, 20, 4225–4241 CrossRef CAS.
- R. Cecilia, U. Kunz and T. Turek, Chem. Eng. Process., 2007, 46, 870–881 CrossRef CAS.
- R. G. Chaudhuri and S. Paria, Chem. Rev., 2012, 112, 2373–2433 CrossRef PubMed.
- C. J. Zhou, S. H. Wang, Y. Zhou, P. F. Rong, Z. Z. Chen, J. Y. Liu and J. Da Zhou, Trans. Nonferrous Met. Soc. China, 2013, 23, 2079–2084 CrossRef CAS.
- M. Milošević, I. Šterbal, U. Feguš, J. Baškovč, B. Prek, U. Grošelj, B. Stanovnik and J. Svete, J. Heterocycl. Chem., 2015, 52, 556–561 CrossRef.
- N.-S. Cho, H.-J. Jeon and D.-U. Heo, J. Korean Chem. Soc., 2012, 56, 658–660 CrossRef CAS.
- P. Sharma, N. Kaur, P. Sharma, R. Sirohi and D. Kishore, Bull. Chem. Soc. Ethiop., 2013, 27, 301–307 CAS.
- L. Wang, J. Luo, Q. Fan, M. Suzuki, I. S. Suzuki, M. H. Engelhard, Y. Lin, N. Kim, J. Q. Wang and C.-J. Zhong, J. Phys. Chem. B, 2005, 109, 21593–21601 CrossRef CAS PubMed.
- S. Sun and H. Zeng, J. Am. Chem. Soc., 2002, 124, 8204–8205 CrossRef CAS PubMed.
- P. Lidström, J. Tierney, B. Wathey and J. Westman, Tetrahedron, 2001, 57, 9225–9283 CrossRef.
- N. Nakajima and Y. Ikada, Bioconjugate Chem., 1995, 6, 123–130 CrossRef CAS PubMed.
Footnote |
† Electronic supplementary information (ESI) available. See DOI: 10.1039/c7ra00830a |
|
This journal is © The Royal Society of Chemistry 2017 |
Click here to see how this site uses Cookies. View our privacy policy here.