DOI:
10.1039/C7RA00701A
(Paper)
RSC Adv., 2017,
7, 14330-14336
High conversion of glucose to 5-hydroxymethylfurfural using hydrochloric acid as a catalyst and sodium chloride as a promoter in a water/γ-valerolactone system
Received
17th January 2017
, Accepted 18th February 2017
First published on 3rd March 2017
Abstract
Biomass derived 5-hydroxymethylfurfural (HMF) is regarded as an important platform molecule for the synthesis of value-added chemicals and fuels, but the high production cost has always been a bottleneck for the industrial scale use of HMF. Different mineral acids (HCl and H2SO4) being used as the catalyst and different salts being used as the reaction promoter were evaluated. It was found that HCl, in combination with NaCl, in a water/γ-valerolactone system showed high selectivity and impressive efficiency for the synthesis of HMF from glucose. The optimal conditions to obtain the best HMF yield (62.45%) were 0.2 M HCl and 0.1 M NaCl at 140 °C with a residence time of 60 minutes. An 18.22% molar yield of LA was obtained as a by-product. The effect of different anions was also investigated, and it was determined that not only the hydrogen ions, but also the nature of the acid and the type of salt played a joint role in improving the HMF yield. In addition, a possible synthesis pathway was proposed for large scale production of HMF.
1. Introduction
The increasing concerns about carbon footprints in the environment indicate that the need for renewable energy will be urgent in the near future, and one of the most promising solutions is to cost-effectively convert sustainable carbohydrates into value-added chemicals and biofuels.1–3 Glucose, which is the monomer unit of cellulose in biomass, has gained increasing attention due to its abundance, environmental friendliness and wide distribution in nature.4–6 Among all the derivatives derived from glucose, 5-hydroxymethylfurfural (HMF) is considered to be one of the most important and versatile platform molecules. It can serve as a precursor for many fine chemicals and biofuel additives, such as levulinic acid, 2,5-furandicarboxylic acid, 2,5-diformylfuran, dihydroxymethylfuran, etc.,7–9 hence, its efficient and economical production from abundant and low-cost glucose has received considerable interest recently.
Most efforts to obtain HMF from glucose have utilized one of the following two routes, (a) combining the isomerization of glucose to fructose together with the subsequent dehydration of fructose to HMF and (b) direct dehydration of glucose to HMF.10,45 The former method requires the participation of both Brønsted acids and Lewis acids, mainly because the Lewis acid is responsible for the isomerization of glucose to fructose and the Brønsted acid favors the dehydration of fructose to HMF.11 However, the latter approach requires only a relatively high concentration of a Brønsted acid.12 It is also worth noting that the addition of salts, such as alkaline earth metal salts and metal halide salts, can effectively enhance the yield of glucose conversion to HMF in ionic liquids and in a biphasic system.13–15,46 Dumesic and co-workers introduced the extracting ratio R (the ratio of HMF in the organic layer to that in the aqueous layer), indicating that the addition of salts increases the R value by means of the salting-out effect, thus increasing the HMF yield and restraining the undesired side reactions.16,17
The reaction medium is deemed to be one of the most significant variables in HMF production.18 To date, the highest HMF yield reported was using ionic liquids such as [BMIM]Cl19 and [EMIM]Cl20 as the solvent. Nevertheless, the high costs associated with product purification and the sensitivity of ionic liquids to water mean they are not feasible for large scale production.21 Another alternative method is a water–organic biphasic system, for which various organic solvents, including dimethylsulfoxide (DMSO),22 tetrahydrofuran (THF)23,24,31 methyl isobutyl ketone (MIBK),14 N,N-dimethylacetamide (DMA),25 γ-valerolactone (GVL),26,27 etc. were reported. Compared to a monophasic reaction system, a biphasic system can remarkably enhance the target product yield. Among all these solvents, it is worth noting that GVL is regarded as a non-toxic and eco-friendly organic solvent.28,29 It is also an important derivative of levulinic acid (LA), which is the degradation product of HMF. Herein, GVL is selected as the reaction solvent for glucose conversion to HMF.
Several groups have reported their explorations about glucose conversion to HMF. Some Lewis acids, such as CrCl2, SnCl4, AlCl3 and FeCl3,30,31 various liquid Brønsted acids such as HCl, H2SO4 and H3PO4,32 the combination of both Lewis acids and Brønsted acids,33–35,46 and also solid acid catalysts such as Sn-Mont,36 and CMK-3,37 were tested in different systems with applying various organic solvents. However, in these studies, solid acids modified with Brønsted acid or Lewis acid sites usually tend to result in only moderate HMF yields. Strong liquid Brønsted acids lead to relatively high HMF yields, but also give rise to undesired degradation of HMF to LA and humins. Other studies include adding sulfate salts and chloride salts into the reaction system,17,38,47 or combining the different salts as co-catalysts to enhance the HMF yield.39 However, the anion effect of Cl− and SO42− on glucose conversion remains to be further explored, and the appropriate type of salt applied to enhance the HMF yield still remains to be decided in the water/GVL system.
In our previous research, it was reported that H2SO4 shows a much higher selectivity on cellulose conversion to LA than HCl with corn stover used as the substrate, while HCl performs better than H2SO4 in HMF production.40 In this study, the different catalytic effects of HCl and H2SO4 to transform glucose to HMF were further assessed in the water/GVL system, and different types of alkali metal salts were tested to decide the suitable additive used to further enhance the HMF yield. In addition, various reaction parameters, including reaction temperature, reaction time, acid concentration and salt dosage were investigated to determine the optimal conditions. Finally, real biomass was also tested in this reaction system and an industrial production model was established.
2. Experimental
2.1 Materials
The corn stover was harvested in the Northern Anhui Province of China and was ground using a high-speed rotary cutting mill, passed through a 40-mesh screen, washed with tap water to get rid of the dust, and oven-dried at 333 K to a constant weight. The corn stover powder was stored in a tightly closed plastic bottle at ambient temperature.
D-Glucose, γ-valerolactone (GVL, 98%), 5-hydroxymethylfurfural (HMF, 98%), and furfural (99%) were purchased from Aladdin Industrial Inc. (Shanghai, China). Sulfuric acid (H2SO4, 96–98%), hydrochloric acid (HCl, 37%), nitric acid (HNO3, 65%) NaCl, Na2SO4, NaHSO4, NaNO3, KCl, K2SO4, LiCl and MgSO4 were purchased from Sinopharm Chemical Reagent Co., Ltd (Shanghai, China). The Aminex HPX-87H column was purchased from Bio-Rad Laboratories Inc. (Hercules, CA, USA). The Waters Symmetry®-C18 column was purchased from Waters Corporation (Milford, MA, USA). All chemicals in this work were commercially available and were used without further purification.
2.2 One-pot conversion of glucose to HMF
The catalytic conversion of glucose to HMF was performed in a stainless steel reactor equipped with mechanical agitation. In a typical experiment, 1.5 g of glucose, 10 mL of water and 40 mL of GVL, together with the 0.2 M acid catalyst and 0.1 M salt, were mixed and loaded into the reactor. The reactor was electrically heated to the target temperature, and after the reaction, the reactor was immediately removed from the heating jacket and quenched in tap water. Samples were diluted with distilled water and filtered with a 0.45 μm syringe filter, prior to HPLC analysis.
2.3 Analysis methods
The quantities of products were determined using a high-performance liquid chromatography (HPLC) system (Waters 515 pump, Waters 2489 UV/Visible Detector, Waters 2414 refractive index detector). Specifically, the quantities of HMF and furfural were determined using a Waters Symmetry®-C18 column (5 μm, 4.6 × 150 mm) and an Ultraviolet Detector (Waters 2489) at 280 nm. The temperature of the column oven was maintained at 30 °C, and water/methanol (3
:
2, v/v), with a flow rate of 0.4 mL min−1, was chosen as the mobile phase. The quantity of levulinic acid was determined using an Aminex HPX-87H column (9 μm, 7.8 × 300 mm) and a Refractive Index Detector (Waters 2414), with the column oven and detector temperature set at 63 °C and 50 °C respectively, and 0.005 M H2SO4 at a flow rate of 0.6 mL min−1 was used as the mobile phase. The HMF, furfural, and levulinic acid detected in both steps were counted together as the final yields.
The yields of HMF and levulinic acid derived from glucose were defined as follows:
|
Glucose conversion (%) = (molars of converted glucose)/(molars of starting glucose) × 100%
| (1) |
|
HMF yield (%) = (molars of HMF produced)/(molars of starting glucose) × 100%
| (2) |
|
HMF selectivity (%) = (HMF yield)/(glucose conversion) × 100%
| (3) |
|
LA yield (%) = (molars of LA produced)/(molars of starting glucose) × 100%
| (4) |
And the yields of HMF, furfural and levulinic acid derived from corn stover were defined as follows:
|
HMF yield (%) = (moles of HMF)/(moles of glucose in corn stover) × 100%
| (5) |
|
Furfural yield (%) = (moles of furfural)/(moles of xylose in corn stover) × 100%
| (6) |
|
LA yield (%) = (moles of LA)/(moles of glucose in corn stover) × 100%
| (7) |
The corn stover used in this experiment was identical to that in our previous research.40
3. Results and discussion
3.1 The catalytic effect of HCl and H2SO4 on HMF yield
The conversion of glucose to HMF may proceed through two routes, via fructose as an intermediate or by direct dehydration. J. Pagan-Torres et al. reported that Lewis acids (AlCl3, SnCl4, VCl3, InCl3 etc.) were principally responsible for the isomerization of glucose,41 and Roman-Leshkov et al. reported that Brønsted acids could not catalyze the isomerization process.42 Kuster and Temmink reported that no glucose to fructose was observed at pH < 4.5 when only Brønsted acids were used as the catalysts.12 In the present work, no fructose was detected during the experiments, hence, the conversion of glucose to HMF most likely proceeds through direct dehydration of glucose when HCl or H2SO4 were chosen as the catalyst.
The different catalytic effects of HCl and H2SO4 with an equimolar amount of hydrogen ions (0.2 mol L−1) are shown in Fig. 1. H2SO4 showed a much higher selectivity towards LA, whilst HCl being used as the catalyst resulted in a fairly high HMF yield of 52.99% at 140 °C with a residence time of 60 min. As a contrast, the highest yield using H2SO4 was 22.06%. It is widely accepted that the generation of HMF requires relatively moderate reaction conditions, and with the increase of temperature, HMF tended to further degrade to LA and other by-products. Marcotullio and Jong reported that Cl− facilitates furfural formation from xylose in acidic solutions,43 Combs et al. claimed that SO42− had a stronger effect on activating the glucose ring leading to a higher HMF production in the water/MIBK system,17 Tyrlik et al. reported that cations like Mg2+ could promote glucose conversion to LA and that Cl− should be completely avoided in HMF production in order to prevent the formation of humins in non-acidic systems.44 However, the conclusions in the above literature present some contradictions with the present work, therefore the competing effect of the cations and anions in the water/GVL system still calls for further in-depth exploration.
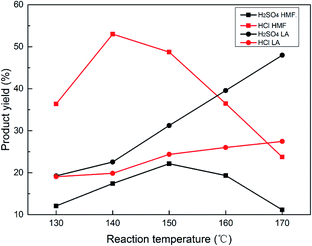 |
| Fig. 1 The catalytic effect of HCl and H2SO4 under different temperatures. Reaction conditions: 0.2 M HCl, 0.1 M H2SO4, VH2O : VGVL = 1 : 4, 60 min. | |
3.2 The effect of adding different salts as promoters on HMF yield
In order to gain a better perspective of the different catalytic effects of HCl and H2SO4 with equimolar H+ but different amounts and types of anions, a series of salts were added into the reaction system to obtain the same amount of cations and anions in the solution. The various cations and anions were also introduced to test their effects on HMF production. Table 1 summarizes the performance of different acids combined with different salts used to convert glucose to HMF. As is shown in entry 1 and entry 2, with identical amounts and types of cations and anions in the reaction solution, HCl and H2SO4 show almost the same catalytic effect on HMF and LA. However, when adding the same type and amount of salts, HCl still performs better than H2SO4 in HMF production (entry 1 vs. entry 4, entry 2 vs. entry 3 and entry 7 vs. entry 6). As the HMF yield in the reaction at 150 °C, using HCl as catalyst and without salt has reached 48.74% (from Fig. 1), adding most types of salts to the HCl solution seems to make no difference to the HMF yield, except with NaCl and KCl (entry 3 and entry 7), while adding salts to H2SO4 resulted in a great enhancement in the HMF yield (entries 2, 4, 5 and 6 with H2SO4 being used as the catalyst, without salt only obtains a 22.12% HMF yield).
Table 1 The performance of different acid and salt combinations on the HMF yielda
Entry |
Catalyst |
Salt |
HMF yield (molar%) |
LA yield (molar%) |
Reaction conditions: 1.5 g glucose, 10 mL H2O, 40 mL GVL, 150 °C, 60 min. |
1 |
0.2 M HCl |
0.1 M Na2SO4 |
49.28 |
16.23 |
2 |
0.1 M H2SO4 |
0.2 M NaCl |
48.56 |
17.56 |
3 |
0.2 M HCl |
0.2 M NaCl |
58.97 |
21.36 |
4 |
0.1 M H2SO4 |
0.1 M Na2SO4 |
48.20 |
14.91 |
5 |
0.1 M H2SO4 |
0.1 M K2SO4 |
29.39 |
14.03 |
6 |
0.1 M H2SO4 |
0.2 M KCl |
48.49 |
18.20 |
7 |
0.2 M HCl |
0.2 M KCl |
59.06 |
16.16 |
8 |
0.2 M HCl |
0.1 M K2SO4 |
32.48 |
14.60 |
9 |
0.2 M HCl |
0.1 M NaNO3 |
1.95 |
10.16 |
10 |
0.1 M H2SO4 |
0.1 M NaNO3 |
3.20 |
32.17 |
11 |
0.1 M HNO3 |
0.1 M Na2SO4 |
0.62 |
33.21 |
12 |
0.1 M HNO3 |
0.1 M NaCl |
2.20 |
17.01 |
14 |
0.2 M HCl |
0.1 M LiCl |
45.40 |
14.78 |
15 |
0.2 M HCl |
0.1 M MgSO4 |
12.78 |
34.58 |
It should be mentioned that some types of salt combined with HCl can also jeopardize the HMF yield, for example, the existence of NO3− was detrimental to HMF generation (entries 9, 10, 11 and 12), and the addition of LiCl and MgSO4 also both had negative effects on the HMF yield (entries 14 and 15). Even with the same type of salt, different cations can cause diverse results. Entries 1 and 8 and also entries 4 and 5 demonstrate that Na2SO4 is superior to K2SO4 in terms of enhancing the HMF yield. These phenomena indicate that the hydrogen ions are not the only factor affecting the glucose conversion to HMF, and that the anions also play a significant role in the dehydration process.
From the aforementioned results, it can be concluded that adding the suitable type of salt into the HCl and H2SO4 solutions will facilitate the conversion of glucose to HMF, and a sodium salt can be one of the best choices due to its abundance and low price, thus various sodium salts were tested; the results are shown in Fig. 2. It is obvious that NaCl performed much better than NaHSO4 and Na2SO4 in enhancing the HMF yield when the catalyst was H2SO4, while when HCl was used as the catalyst, adding NaHSO4 and Na2SO4 had a negligible effect on the system, however adding NaCl enhanced the HMF yield from 48.74% to 56.46%. NaNO3 decreased the HMF yield when either HCl or H2SO4 was used as the catalyst. Thus NaCl can be considered as the salt additive which functions as the reaction promoter in this study.
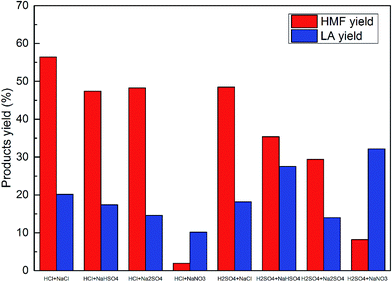 |
| Fig. 2 Effects of different sodium salts used as additives on the HMF and LA yields. Reaction conditions: 1.5 g of glucose, 10 mL of H2O, 40 mL of GVL, 150 °C and 60 min. All salts added in the solution were of 0.1 M concentration. | |
3.3 Effect of NaCl as the promoter on the HMF yield
Further experiments were performed to probe the role of NaCl in the glucose conversion to HMF under different temperatures, using HCl and H2SO4 as the catalyst.
Fig. 3a–c depict the glucose conversion, the HMF yield and the LA yield under the reaction temperature range of 130 °C to 170 °C, with and without NaCl. Fig. 3a shows that the glucose conversion surprisingly increased with temperature, whilst adding NaCl dramatically promoted the glucose conversion both with HCl and H2SO4 under relatively low temperature conditions. For example, H2SO4 without NaCl at 140 °C resulted in 76.1% glucose conversion, while after adding NaCl, the glucose conversion reached 93.2%, and the glucose conversion using HCl at 130 °C was 92.34% vs. 76.21% with and without NaCl, respectively. Fig. 3b shows that the optimal temperature for HCl was 140 °C, for which the highest HMF yield was 62.45%, while the suitable temperature for H2SO4 was 150 °C for which the highest HMF yield was 53.85%. Whether with or without NaCl as the additive, the optimal temperature for HCl and H2SO4 was 140 °C and 150 °C, respectively. Fig. 3c indicates that HMF degrades to LA with an increase in temperature, however, the addition of NaCl suppressed such a tendency. Thus, it can be concluded that the combination of HCl and NaCl is superior to H2SO4 combined with NaCl, in terms of achieving a high yield of HMF from glucose. HCl was therefore selected for further studies because of its higher selectivity towards HMF.
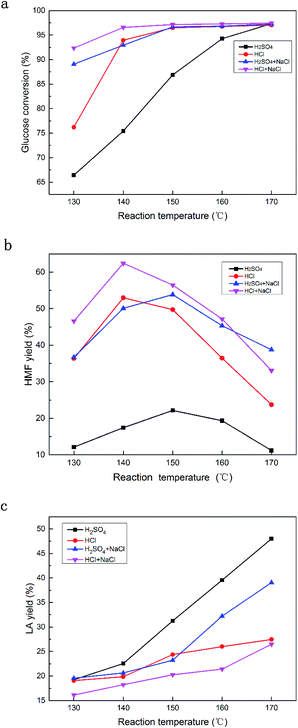 |
| Fig. 3 The effect of adding NaCl to HCl and H2SO4 in catalyzing the glucose conversion to HMF and LA. Reaction conditions: 1.5 g of glucose, 10 mL of H2O, 40 mL of GVL, 0.2 M HCl, 0.1 M H2SO4, 0.1 M NaCl and residence time of 60 min. | |
3.4 Effect of HCl concentration on HMF yield
The effect of the concentration of HCl on the HMF yield was also investigated. The results of 0.1–0.3 M HCl in combination with 0.1 M NaCl, in the temperature range of 130 °C to 170 °C are shown in Fig. 4a and b. It was found that the reaction temperature and acid concentration played a joint role in achieving a good yield of HMF; a lower acid concentration required a higher reaction temperature and vice versa. More specifically, with 0.1 M HCl being used as the catalyst, the highest HMF yield of 53.69% was achieved at 150 °C, while with 0.2 M or 0.3 M HCl, the highest HMF yields of 62.45% and 59.87% were achieved at 140 °C. A higher temperature will cause the HMF conversion to LA. According to Fig. 4a and b, a high acid concentration (0.3 M) led to a much faster degradation rate than for a low acid concentration (0.1 M) above 150 °C, which favored the generation of LA. In summary, a moderate HCl concentration results in a satisfactory HMF yield, while a low acid concentration is not sufficient for the high yield of HMF, and a further increase of HCl concentration does not seem to improve the HMF yield much.
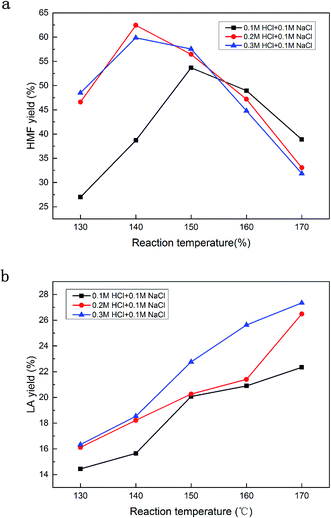 |
| Fig. 4 The effect of acid concentration on the HMF and LA yields. Reaction conditions: 1.5 g of glucose, 10 mL of H2O, 40 mL of GVL, 0.1 M NaCl and a residence time of 60 min. | |
3.5 Effect of NaCl dosage on HMF yield
Fig. 5 shows the effect of the amount of NaCl on the HMF and LA yields. 0.2 M HCl was applied as the catalyst, and 0.05 M, 0.1 M, 0.2 M, 0.3 M concentrations of NaCl were added as the reaction promoter at different temperatures. As with the aforementioned result, the highest HMF yield was achieved at 140 °C. A low amount of NaCl (0.05 M) did not sufficiently promote HMF production, however, a very high amount of NaCl (0.2 M or 0.3 M) also did not result in a higher HMF yield than that obtained using 0.1 M. A slightly improved yield of LA was observed with the increased amount of NaCl.
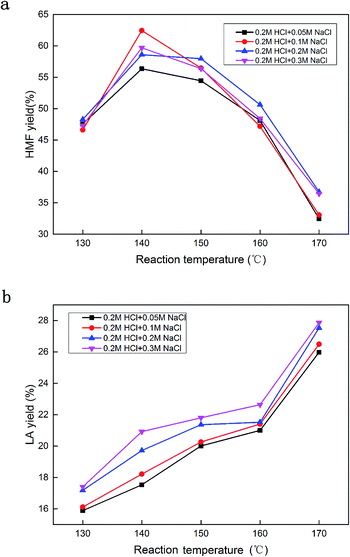 |
| Fig. 5 The effect of NaCl dosage on the HMF and LA yields. Reaction conditions: 1.5 g of glucose, 10 mL of H2O, 40 mL of GVL, 0.2 M HCl and a residence time of 60 min. | |
It is probable that an excessive amount of NaCl not only promotes the glucose conversion to HMF, but also accelerates the rehydration of HMF to LA. From an application point of view, reducing the additive amount is also cost-effective, thus a suitable amount of NaCl as an additive is vital in the glucose conversion to the HMF process.
3.6 Effect of reaction time on the HMF yield
Other reaction parameters such as residence time were also investigated to obtain a better understanding of the conversion process. As the optimal temperature, acid concentration and NaCl amount have been decided, a series of experiments were conducted with the reaction time range of 10 min to 90 min and the results are shown in Fig. 6. It was found that within the first 30 min, the glucose conversion and the HMF yield increased rapidly, and after 50 min, the HMF yield reached approximately 60% and became steady. The maximum yield of 62.45% was obtained at 60 min and a longer reaction time even caused a slight decrease in the HMF yield and an increase in the LA yield. The LA yield slowly increased with time, and after 50 min it reached a plateau.
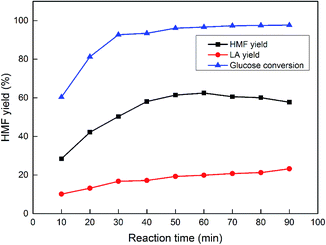 |
| Fig. 6 The effect of the reaction time on the HMF and LA yields. Reaction conditions: 1.5 g of glucose, 10 mL of H2O, 40 mL of GVL, 0.2 M HCl, 0.1 M NaCl and a reaction temperature of 140 °C. | |
3.7 The catalytic performance on corn stover as a substrate
As discussed above, the combination of HCl and NaCl exhibits great performance in converting glucose to HMF in the water/GVL system. As one of the most abundant biomass resources in nature, corn stover was also tested to verify the potential of the present reaction system. According to our previous work, the decomposition of corn stover required a higher temperature and longer reaction time, thus the conditions of 170 °C and 90 min were applied herein. The results are demonstrated in Fig. 7.
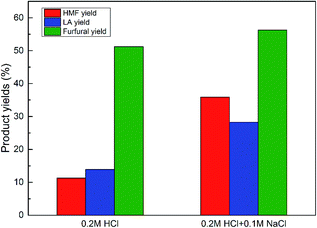 |
| Fig. 7 The catalytic effect of HCl–NaCl on corn stover. Reaction conditions: 3.5 g of corn stover, 10 mL of H2O, 40 mL of GVL, 0.2 M HCl, 0.1 M NaCl, 170 °C, 90 min. | |
Interestingly, the addition of NaCl into the reaction system not only remarkably enhanced the HMF yield from 10.5% to 35.87%, but also enhanced the LA yield from 11.8% to 27.6%, and slightly enhanced the furfural yield, showing the great potential of such a reaction system in industrial applications.
3.8 A proposed large-scale application model
One possible large-scale production route is shown in Fig. 8. The most suitable reaction conditions (residence time, temperature, HCl concentration and NaCl amount etc.) are applied in the reactor, and after the reaction is completed, the reaction liquid is transferred to an evaporator to distill the HMF generated. After that the remaining H2O/GVL mixture, which is rich in LA, can be recycled for a new reaction. It should be noted that HMF readily degrades to LA in acidic solution, and as LA can be much more steady than HMF under such an environment, each new reaction could enrich the concentration of LA in the H2O/GVL reaction system. After several cycles, the LA concentration in the reaction liquid will reach a relatively high level, and then the LA can be separated from the system as a by-product, or directly converted to GVL to compensate for the GVL loss in the reaction process. In this way, it is desirable that HMF can be produced from glucose in a cost-efficient, energy-saving way and on a commercial scale.
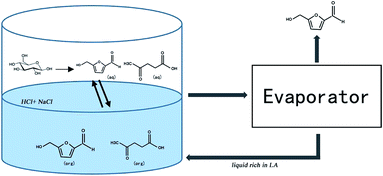 |
| Fig. 8 One possible pathway of HMF production from glucose in a H2O/GVL–HCl system. | |
4. Conclusions
In this study, the catalytic activity of HCl and H2SO4 to convert glucose to HMF in H2O/GVL was systematically investigated. HCl was proved to show high selectivity towards HMF generation, and H2SO4 was beneficial for LA production. A simple modification of the reaction system by adding salt was shown to greatly improve the HMF yield and the glucose conversion when also using H2SO4 as catalyst, however only certain kinds of chloride salt were shown to improve the HMF yield when using HCl as the catalyst. HNO3 and nitrate ions seemed to have a negative effect on the HMF yield, thus it is concluded that the pH or hydrogen ions are not the only catalytic factors influencing the glucose conversion to HMF, but also the nature of the acid and the addition of certain salts and the existence of certain kinds of anions play a joint role in improving the HMF yield.
HCl and NaCl were selected as the catalyst and reaction promoter respectively in the present study, due to the high selectivity and low price. An impressively high yield of 62.45% of HMF and 18.22% of LA were obtained at 140 °C and in 60 min in a H2O/GVL system, in which 0.2 M HCl was used as the catalyst and 0.1 M NaCl was used as the reaction promoter. A possible model for industrial applications was proposed, showing the high potential of such a system in the glucose conversion to HMF.
Acknowledgements
This study was financially supported by the National Key Technology R&D Program of China (No. 2015BAD15B06), Science and Technological Fund of Anhui Province for Outstanding Youth (1508085J01) and the international technology cooperation plan of Anhui (No. 1503062030).
Notes and references
- S. Dutta and S. Pal, Biomass Bioenergy, 2014, 62, 182–197 CrossRef CAS.
- F. Cherubini, Energy Convers. Manage., 2010, 51, 1412–1421 CrossRef CAS.
- S. K. Maity, Renewable Sustainable Energy Rev., 2015, 43, 1446–1466 CrossRef CAS.
- R. J. Van Putten, J. C. van der Waal, E. D. De Jong, C. B. Rasrendra, H. J. Heeres and J. G. de Vries, Chem. Rev., 2013, 113, 1499–1597 CrossRef CAS PubMed.
- P. Zhou and Z. Zhang, Catal. Sci. Technol., 2016, 6, 3694–3712 CAS.
- Z. Xue, M. G. Ma, Z. Li and T. Mu, RSC Adv., 2016, 6, 98874–98892 RSC.
- A. Mukherjee, M. J. Dumont and V. Raghavan, Biomass Bioenergy, 2015, 72, 143–183 CrossRef CAS.
- B. Saha and M. M. Abu-Omar, Green Chem., 2014, 16, 24–38 RSC.
- X. Tong, Y. Ma and Y. Li, Appl. Catal., A, 2010, 385, 1–13 CrossRef CAS.
- V. Choudhary, S. H. Mushrif, C. Ho, A. Anderko, V. Nikolakis, N. S. Marinkovic and D. G. Vlachos, J. Am. Chem. Soc., 2013, 135, 3997–4006 CrossRef CAS PubMed.
- Y. Zhang, J. Wang, X. Li, X. Liu, Y. Xia, B. Hu and Y. Wang, Fuel, 2015, 139, 301–307 CrossRef CAS.
- B. F. Kuster and H. M Temmink, Carbohydr. Res., 1977, 54, 185–191 CrossRef CAS.
- W. Mamo, Y. Chebude, C. Márquez-Álvarez, I. Díaz and E. Sastre, Catal. Sci. Technol., 2016, 6, 2766–2774 CAS.
- T. S. Hansen, J. Mielby and A. Riisager, Green Chem., 2011, 13, 109–114 RSC.
- Y. Román-Leshkov and J. A. Dumesic, Top. Catal., 2009, 52, 297–303 CrossRef.
- Y. Román-Leshkov, C. J. Barrett, Z. Y. Liu and J. A. Dumesic, Nature, 2007, 447, 982–985 CrossRef PubMed.
- E. Combs, B. Cinlar, Y. Pagan-Torres, J. A. Dumesic and B. H. Shanks, Catal. Commun., 2013, 30, 1–4 CrossRef CAS.
- V. Vasudevan and S. H. Mushrif, RSC Adv., 2015, 5, 20756–20763 RSC.
- S. J. Dee and A. T. Bell, ChemSusChem, 2011, 4, 1166–1173 CrossRef CAS PubMed.
- X. Qi, M. Watanabe, T. M. Aida and R. L. Smith Jr, Cellulose, 2011, 18, 1327–1333 CrossRef CAS.
- C. Moreau, A. Finiels and L. Vanoye, J. Mol. Catal. A: Chem., 2006, 253, 165–169 CrossRef CAS.
- J. N. Chheda, Y. Román-Leshkov and J. A. Dumesic, Green Chem., 2007, 9, 342–350 RSC.
- X. Li, Q. Xia, K. Peng, X. Liu, N. Essayem and Y. Wang, Catal. Sci. Technol., 2016, 6, 7586–7596 CAS.
- J. Sun, X. Yuan, Y. Shen, Y. Yi, B. Wang, F. Xu and R. Sun, Ind. Crops Prod., 2015, 70, 266–271 CrossRef CAS.
- C. Wang, L. Fu, X. Tong, Q. Yang and W. Zhang, Carbohydr.Res., 2012, 347, 182–185 CrossRef CAS PubMed.
- L. Zhang, H. Yu, P. Wang and Y. Li, Bioresour. Technol., 2014, 151, 355–360 CrossRef CAS PubMed.
- L. Qi, Y. F. Mui, S. W. Lo, M. Y. Lui, G. R. Akien and I. T. Horváth, ACS Catal., 2014, 4, 1470–1477 CrossRef CAS.
- D. M. Alonso, S. G. Wettstein and J. A. Dumesic, Green Chem., 2013, 15, 584–595 RSC.
- I. T. Horváth, H. Mehdi, V. Fábos, L. Boda and L. T. Mika, Green Chem., 2008, 10, 238–242 RSC.
- S. Hu, Z. Zhang, J. Song, Y. Zhou and B. Han, Green Chem., 2009, 11, 1746–1749 RSC.
- Y. Shen, J. Sun, Y. Yi, M. Li, B. Wang, F. Xu and R. Sun, Bioresour. Technol., 2014, 172, 457–460 CrossRef CAS PubMed.
- F. Salak Asghari and H. Yoshida, Ind. Eng. Chem. Res., 2006, 45, 2163–2173 CrossRef.
- M. Moreno-Recio, J. Santamaría-González and P. Maireles-Torres, Chem. Eng. J., 2016, 303, 22–30 CrossRef CAS.
- H. Xia, S. Xu and L. Yang, RSC Adv., 2017, 7, 1200–1205 RSC.
- Y. Yang, C. Hu and M. M. Abu-Omar, J. Mol. Catal. A: Chem., 2013, 376, 98–102 CrossRef CAS.
- J. Wang, J. Ren, X. Liu, J. Xi, Q. Xia, Y. Zu and Y. Wang, Green Chem., 2012, 14, 2506–2512 RSC.
- J. M. R. Gallo, R. Alamillo and J. A. Dumesic, J. Mol. Catal. A: Chem., 2016, 422, 13–17 CrossRef CAS.
- X. Wu, J. Fu and X. Lu, Bioresour. Technol., 2012, 119, 48–54 CrossRef CAS PubMed.
- N. Shi, Q. Liu, Q. Zhang, T. Wang and L. Ma, Green Chem., 2013, 15, 1967–1974 RSC.
- M. Li, W. Li, Q. Liu, H. Jameel, H. M. Chang, S. An and L. Ma, BioResources, 2016, 11, 8239–8256 CAS.
- Y. J. Pagan-Torres, T. Wang, J. M. R. Gallo, B. H. Shanks and J. A. Dumesic, ACS Catal., 2012, 2, 930–934 CrossRef CAS.
- Y. Román-Leshkov, M. Moliner, J. A. Labinger and M. E. Davis, Angew. Chem., Int. Ed., 2010, 49, 8954–8957 CrossRef PubMed.
- G. Marcotullio and W. De Jong, Green Chem., 2010, 12, 1739–1746 RSC.
- S. K. Tyrlik, D. Szerszen, M. Olejnik and W. Danikiewicz, J. Mol. Catal. A: Chem., 1996, 106, 223–233 CrossRef CAS.
- J. Tang, L. Zhu, X. Fu, J. Dai, X. Guo and C. Hu, ACS Catal., 2016, 7, 256–266 CrossRef.
- J. Li, Z. Jiang, L. Hu and C. Hu, ChemSusChem, 2014, 7, 2482–2488 CrossRef CAS PubMed.
- Z. Jiang, J. Yi, J. Li, T. He and C. Hu, ChemSusChem, 2015, 8, 1901–1907 CrossRef CAS PubMed.
|
This journal is © The Royal Society of Chemistry 2017 |
Click here to see how this site uses Cookies. View our privacy policy here.