DOI:
10.1039/C7RA00509A
(Paper)
RSC Adv., 2017,
7, 16836-16842
Hollow Au loaded with kanamycin for pharmacological and laser-triggered photothermal sterilization †
Received
12th January 2017
, Accepted 3rd March 2017
First published on 17th March 2017
Abstract
Effective and appropriate use of existing antibiotics is imperative because of the problem of antibiotic resistance. Driven by this need, a platform of hollow Au loaded with kanamycin (hAu-kana) was produced. This platform has the ability to target drug delivery and facilitate photothermal sterilization. Hollow Au was synthesized by galvanic replacement reaction. We then covalently attached anti-E. coli to hollow Au modified with the ligands SH-PEG and L-cysteine. SH-PEG prevents the aggregation of AuNPs, and the carboxyl group of the L-cysteine further links with IgG. The dual-ligand hollow Au can be targeted to deliver the drug after modification with the anti-E. coli, thus improving the sterilization efficiency. This was followed by loading the model drug kanamycin (hAuNPs-anti-E. coli-kana) to facilitate photothermal treatment. The bactericidal effect on the bacterial strain was investigated by incubating E. coli with the hybrid and eventually subjecting it to laser irradiation (650 nm, 200 mW cm−2, diameter 2 mm, 40 min). Compared with E. coli treated with hAuNPs-anti-E. coli-kana alone, irradiation of E. coli treated with hAuNPs-anti-E. coli-kana with laser has obvious synergistic effect and made the sterilization more effective. Taken together, this hybrid suggests a new method for achieving synergistic bactericidal effect.
Introduction
The problem of antibiotic resistance is not only man-made; resistance genes have been present in the bacterial population long before humans started to use antibiotics to fight bacterial infections.1,2 Despite an armamentarium of antibacterial, antifungal and antiviral agents for the treatment of most infectious diseases, the biggest threats today are resistant pathogens, especially those in hospitals.3–6 In order to meet the growing problem of antibiotic resistance among pathogens, the development of new treatments for infectious diseases that will ensure effective and appropriate use of existing antibiotics is imperative.
Hollow Au nanoparticles (hAuNPs) have the unique characteristics of small size (outer diameter, ∼50 nm), spherical shape, a hollow interior and, especially, good biocompatibility, and they present promising application prospects in various fields.7–11 As a substitute for complex processing with special equipment requirements, a more simple and effective method can allow chloroauric acid (HAuCl4) to undergo spontaneous transformation in sodium borohydride (NaBH4) solution and produce hollow structures.12,13 The obtained hAuNPs are most suitable for serving as drug carriers with hollow structure.14 Meanwhile, the characteristics of the hAuNP surface present the possibility of covalent modification with other biomolecules. Taking into account the biocompatibility, load and target problems of nanomaterials in application, in this work, we chose the hAuNPs and antibody modification of hAuNPs. At the same time, under irradiation conditions, the hAuNPs containing chemical drugs can provide the usual chemical and physical sterilization functions.
It is very important to improve the stability of hAuNPs in this system. O-[2-(3-Mercaptopropionylamino)ethyl]-O′-methylpolyethylene glycol (SH-PEG) is a commonly used ligand to stabilize metal nanoparticles (NPs) in different pH and salt solutions.15–18 However, due to the SH-PEG surface not having functional groups, it cannot connect with antibodies, enzymes or others, limiting its application. Furthermore, carboxyl functionalization seems to attenuate toxicity.19 Because of this, we used the method of dual-ligand modification of hAuNPs, in which the surface gains carboxyl groups to react with the antibody, and SH-PEG coating, which greatly improved its stability in the medium.20,21 HAuNPs with a mixed monolayer of SH-PEG and L-cysteine (1
:
10 volume ratio) covalently conjugated to IgG through Au–NH2. HAuNPs modified with the antibody had targeted drug transport property, which could selectively reduce the drug's side effects.
Nanomaterial-mediated photothermal therapy (PTT) is a promising strategy for sterilization. Its principle is mainly via damage to the integrity of the cell structure by degrading the nuclear membrane and endoplasmic reticulum.22–26 This type of light-triggered treatment modality has improved selectivity and lowered side effects. Various kinds of NPs have been reported to be able to enter cells by endocytic pathways.27–29 AuNPs are known to be nontoxic and nonimmunogenic. AuNPs also have the characteristics of light absorption.30 Here, we used the photothermal effect of hAuNPs to reach a synergistic bactericidal effect with the drug.
In this study, we report a hybrid bactericidal material, hAuNPs that are dual-ligand modified, anti-E. coli conjugated and kanamycin-loaded (hAuNPs-anti-E. coli-kana), which could selectively target bacteria and kill them by combining the effects of kanamycin and photothermal therapy. Meanwhile, the hybrid could reduce the risk of abuse of broad-spectrum antibiotics. In addition, the synergistic effect of the chemical drug and physical sterilization of hAuNPs-anti-E. coli-kana is an improvement on the bactericidal effect.
Experimental section
Instrumentation
UV-vis absorption spectra are obtained with a spectrophotometer (Thermo Scientific, Nanodrop 1000, USA). Magnetic stirrer is used in material synthesis (Shanghai Sile instrument Co. Ltd., S21-3, China). The concentration of bacteria in the suspension is measured using a cell density meter (Amersham Biosciences, Ultrospec 10, USA). Zetasizer is used to measure the size and the zeta potential of the NPs (Malvern, Nano ZS-90, UK).
Other apparatus used also includes the following: air clean bench (Aetna Corp, SW-CJ-1FD, China), the classical multi-amplitude orbital shaker (Zhicheng Analytical Instrument Manufacturing Co., Ltd., ZWY-100H, China), digital thermometer (Wanda, TP 101, China), low-temperature incubator (Shellab, SRI3-2, USA), Labo autoclave (Sanyo, MLS-3780, Japan), confocal fluorescence microscope (Nikon, Eclipse 80i, Japan), laser head (Diamond Electronics Co., Ltd., 650 nm, 200 mW cm−2, China), pH meter (Leici, PHS-3C, China), scanning electron microscopy (SEM) (Hitachi, S-3000 N, Japan), transmission electron microscopy (TEM) (Hitachi, 7500, Japan) for characterization of NPs, and TEM (JEOL, JEM-1400Plus, Japan) for the study of bacterial slices.
Reagents
Cobalt chloride, sodium borohydride, O-[2-(3-mercaptopropionylamino)ethyl]-O′-methylpolyethylene glycol (SH-PEG), and N-hydroxysuccinimide (NHS) were purchased from Sigma-Aldrich. Sodium citrate, chloroauric acid, kanamycin, culture dish, crystal violet staining solution, Gram's iodine, and Safranin O were purchased from Sangon Biotech. L-Cysteine was purchased from Bio Basic, Inc. 1-Ethyl-3-(3-dimethylaminopropyl) carbodiimide (EDC) was purchased from Damas-Beta. Escherichia coli was purchased from American Type Culture Collection (ATCC) (Fig. S1†). Antibody to E. coli (anti-E. coli) was purchased from Abcam.
The Luria–Bertani (LB) nutrient solution was prepared by dissolving sodium chloride, tryptone and yeast extract in deionized (DI) water. LB solid medium was obtained by adding agar. Phosphate-buffered saline (PBS) was prepared by mixing the solutions of NaH2PO4 and Na2HPO4. DI water purified through a Millipore system (≥18 MΩ cm) was employed in all runs.
Preparation of the hAuNPs
HAuNPs were synthesized according to a previously reported method with a little modification.31 Briefly, cobalt nanoparticles (CoNPs) were first synthesized by deoxygenating 100 mL DI water containing 400 μL of 0.1 M sodium citrate and 100 μL of 0.4 M cobalt chloride, and bubbling the solution with nitrogen (∼20–30 min). Sodium borohydride (100 μL, 1 M) was then added. The slightly pink solution turned light grey and then brown on the addition of sodium borohydride, indicating the reduction of Co(II) and the formation of CoNPs. The solution was allowed to react for 45 min under constant nitrogen flow before the addition of gold. Thereafter, 30 mL of the CoNP solution was transferred immediately to a vortexing solution of 10 mL DI containing 15 to 35 μL of 0.1 M chloroauric acid. The cobalt immediately reduced the gold ions onto the surface of CoNPs, while at the same time, it was oxidized to cobalt oxide. Any remaining cobalt core was further oxidized by air, resulting in the final product, hAuNPs.
Preparation of hAuNPs-anti-E. coli
SH-PEG (10 μL) and L-cysteine (100 μL) were added to the hAuNP solution, stirred for 30 s, and then stored at 4 °C for further use.
After the activation of carboxylic acid group with 2 mM of EDC and 5 mM of NHS in 0.1 M of PBS, 10 μL of the antibody was added to the pre-modified hAuNP dispersion. The mixture was shaken for 2 h at room temperature. After centrifugation (5000 rpm s−1, 15 min, 4 °C) to remove excess antibodies, the precipitate was resuspended in PBS solution and stored at 4 °C, to be used within a week.
Loading of kanamycin on hAuNPs-anti-E. coli
Kanamycin was loaded into hAuNPs-anti-E. coli (1 mg mL−1) by mixing the NPs with a kanamycin solution (1 mg mL−1) in PBS (0.1 M, 1 mL). The mixture was gently shaken at room temperature for 24 h in the dark. Free kanamycin was removed by washing with PBS followed by centrifugation, done several times.
Characterization of hAuNPs
UV-vis absorption spectra were used for analysis of sample composition. The zeta potentials of the samples were determined on a Zetasizer at 25 °C. The structure and morphology of samples were observed with TEM.
Verification of photothermal properties of hAuNPs
To verify the photothermal effect of hAuNPs, 10 mL of SH-PEGylated hAuNPs (0.5 mM) in a glass vial were irradiated by 650 nm laser with an intensity of 200 mW cm−2 (diameter 2 mm) for 40 min, and the temperature changes were measured by a digital thermometer.
Cytotoxicity assay
E. coli were inoculated in LB broth and grown at 37 °C for 16 h, then stored at 4 °C for use. E. coli (0.5 MCF) were diluted with LB broth to 1 × 105 cfu mL−1 and stored as the original bacterial solution. The as-prepared E. coli solution (50 μL) was mixed with diverse concentrations of hAuNPs (0.3 mM, 0.5 mM, 1.0 mM, 2.0 mM, 30 μL, respectively) and diluted with LB broth to 500 μL. Then, 100 μL of the bacterial solutions were placed on LB solid medium and cultured at 37 °C for 16 h. The bacterial toxicity was evaluated by counting the number of bacterial colonies.
Photothermal treatment of bacteria by the hAuNPs-anti-E. coli-kana nanocapsules
To evaluate the bactericidal property of the hAuNPs-anti-E. coli-kana nanocapsules, E. coli (0.5 MCF) solution was diluted with broth to 1 × 105 cfu mL−1 and stored as original bacterial solution. The as-prepared E. coli solution (50 μL) was mixed with hAuNPs-anti-E. coli-kana nanocapsules (30 μL) and diluted with LB broth to 500 μL. Three samples of the same solution were prepared in parallel. The obtained bacterial mixture was irradiated for 40 min under laser, then shaken for 10 min. Bacteria from the above solution (100 μL) was coated on the LB solid medium, and the number of bacterial colonies was counted after 37 °C culture for 16 h. Control experiments were performed in parallel, i.e., the bacteria diluted with LB broth in the absence of hAuNPs-anti-E. coli-kana nanocapsules. To illustrate that the combination of chemical drug and physical photothermal bactericidal effect caused bacterial death, the presence of kanamycin-only and bare hAuNPs under laser irradiation were investigated in accordance with the steps described in the previous procedures.
TEM images of hAuNPs-anti-E. coli-kana-treated bacteria
The prepared hAuNPs-anti-E. coli-kana was added into the bacterial solution in a flask and incubated for 4 h at 37 °C to induce receptor-mediated combination of NPs. Untreated bacterial solution was prepared as a control. Then, TEM samples were prepared. The bacteria were fixed in a fixing solution overnight at 4 °C. The fixed bacteria were dehydrated in serially diluted ethanol (30, 50, 70, 80, 90 and 100%). After dehydration, the bacteria were infiltrated at 70 °C overnight. The samples were cut into ultrathin sections and mounted on a copper grid. Then, the sections were stained and observed with a JEM-1400Plus TEM.
Results and discussion
Fabrication of the hAuNPs-anti-E. coli-kana
The overall schematic diagram for the synthesis of target-specific hAuNPs-anti-E. coli-kana is shown in Scheme 1. First, hAuNPs (∼50 nm in diameter) were prepared by galvanic replacement reaction. During the galvanic replacement reaction, the CoNPs were galvanically replaced by gold ions, accompanying the generation of a hollow interior, resulting in an increase of drug loading capacity. As-prepared hAuNPs were coated with SH-PEG/L-cysteine (molar ratio 1
:
10) to provide biocompatibility and bio-conjugation sites. After modification, anti-E. coli was conjugated to the carboxyl group of pre-modified hAuNPs by EDC/NHS coupling method. Lastly, kanamycin was introduced into the hAuNPs-anti-E. coli carrier. Kanamycin was loaded by external (DO, “drug outside” on the exterior surface area of hAuNPs) and internal loading (DI, “drug inside” the cavity of hAuNPs) methods to the hAuNPs.
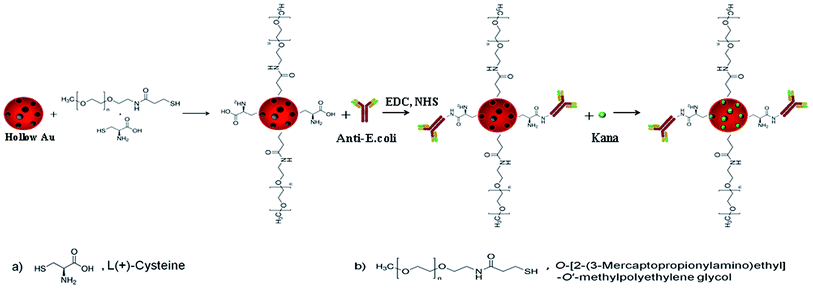 |
| Scheme 1 Schematic illustration for the preparation of hAuNPs-anti-E. coli-kana. After the synthesis of hAuNPs by galvanic replacement reaction, hAuNPs were modified with SH-PEG and L-cysteine to allow conjugation of the E. coli antibody and increase biocompatibility. Next, E. coli antibody was conjugated through activation of the carboxyl groups at the surface of pre-modified hAuNPs, followed by kanamycin loading. | |
Characterization of the hAuNPs-anti-E. coli-kana
Homogeneous distribution of the prepared hAuNPs dispersed in DI water was observed in the TEM image (Fig. 1A). Fig. 1B is an amplification of the hAuNP image, and obvious hollow structure is seen. The hAuNPs were uniformly dispersed in the solution without obvious aggregation, mainly due to the negative charge on the surface of the particles. Particle diameter analysis was measured at 25 °C with the Zetasizer Nano ZS90, as shown in Fig. 1C. Zetasizer Nano ZS90 uses dynamic light scattering (DLS) to measure particle size with a 90 degree scattering angle. The prepared hAuNPs had relatively good stability, as shown in Fig. S2.†
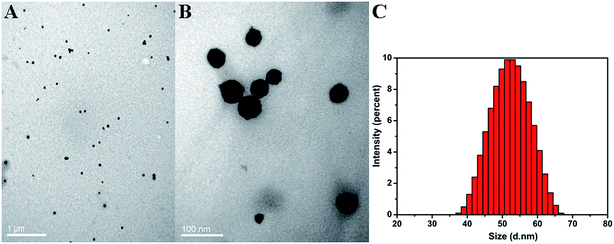 |
| Fig. 1 (A) TEM and (B) amplification images, and (C) particle diameter analysis of hAuNPs at 25 °C. | |
The plasmonic spectra of the hAuNPs were measured by UV-vis spectrometry, as shown in Fig. 2A. The plasmonic band of the hAuNPs formed a peak at 630 nm, which was attributed to the hAuNPs, in comparison with that of the CoNPs, due to galvanic replacement. Strong absorbance of hAuNPs could be related to a high potential for heat generation upon irradiation with laser.32,33 The antibody covalent-bonding and kanamycin-loading properties of hAuNPs-anti-E. coli-kana were evaluated. UV-vis spectra revealed a newly appeared peak at around 280 nm, corresponding to anti-E. coli, after antibody bonding. After kanamycin loading, the curve changed to (c) (Fig. 2B). The hollow structure of hAuNPs promoted kanamycin loading inside its interior spaces.
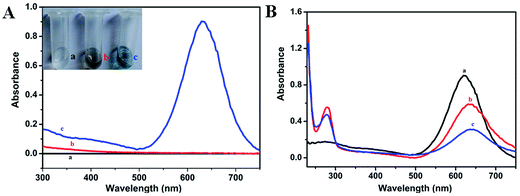 |
| Fig. 2 (A) UV-vis spectra of (a) DI water, (b) CoNPs and (c) hAuNPs. Illustration is the solution color of the corresponding curve. (B) UV-vis spectra of (a) hAuNPs and (b) hAuNPs conjugated with anti-E. coli through dual-ligand and (c) hAuNPs-anti-E. coli loaded with kanamycin. | |
To further verify the successful synthesis, the surface charges of these NPs at different stages were determined by zeta potential measurement.34–36 The zeta potential of the samples after incubation are provided in Fig. 3. A significant transition of the zeta potential from negative −25.5 mV to −10.8 mV was observed after each step of modification, confirming the successful preparation of hAuNPs-anti-E. coli-kana.
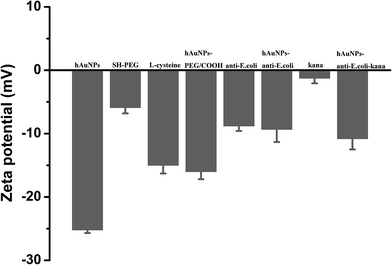 |
| Fig. 3 The corresponding zeta potential measured at each step of the preparation process. The error bars represent the standard deviation of three measurements. | |
Condition optimization
In order to prove that the hAuNPs-anti-E. coli-kana was suitable for the bactericidal effect, we evaluated the bactericidal properties of hAuNPs and kanamycin separately. Fig. 4A shows that at a hAuNP concentration of 2.0 mM, about 80% of E. coli was still viable. On the other hand, the increasing kanamycin concentration from 0–10 mg mL−1 led to improvement of the bactericidal effect, and the survival rate of E. coli decreased to 6% at 5 mg mL−1 (Fig. 4B). For the purpose of studying the bactericidal ability of the hAuNPs-anti-E. coli-kana hybrid, it is necessary to maintain the survival of E. coli. In this respect, the concentration of hAuNPs and kanamycin was set to 0.5 mM and 1 mg mL−1, respectively.
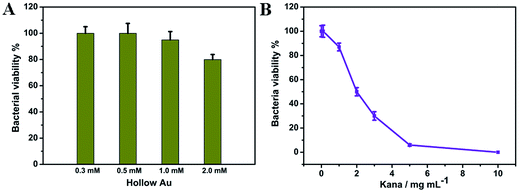 |
| Fig. 4 The viability of E. coli with the concentration of (A) hAuNPs and (B) kanamycin. The concentration of E. coli: 1 × 105 cfu mL−1; hAuNPs: 0.3 mM, 0.5 mM, 1.0 mM, 2.0 mM; kanamycin: 0, 0.1, 1.0, 2.0, 3.0, 5.0, 10.0 mg mL−1. The data were averaged from three parallel measurements. | |
Photothermal property of hAuNPs
HAuNPs-anti-E. coli-kana has three functions: targeting specific bacteria via the anti-E. coli, photothermal treatment by laser irradiation, and drug delivery using the hAuNPs (Scheme 2). Receptor-mediated delivery for target cells is more effective than non-specific.37–40 After targeting, the bacteria were exposed to the laser. Meanwhile, the targeted bacteria were subjected to photothermal effects and the drug.
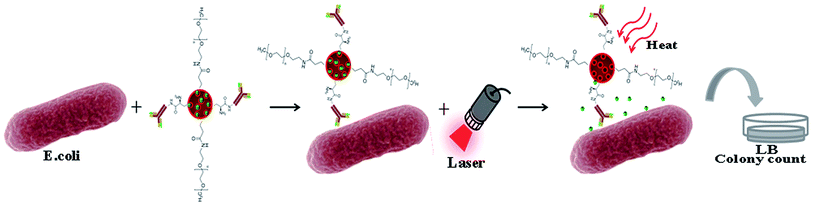 |
| Scheme 2 The hAuNPs-anti-E. coli-kana complexes effectively lead to specific bacterial death through the synergetic effects of kanamycin release and heat generation. | |
To confirm the photothermal effect, temperature changes of the hAuNP dispersion in DI water were measured upon irradiation using a laser with a wavelength of 650 nm. As shown in Fig. 5, the temperature of the hAuNP dispersion (0.5 mM) gradually increased from 29.1 °C to 43.0 °C (ΔT = 13.9 °C) upon irradiation (650 mW cm−2, 40 min), whereas the temperature of DI water rose by less than 2 °C after irradiation. This elevation in temperature could induce bacterial death and thus the combined effects on bacteria of radiation and drug sensitivity.
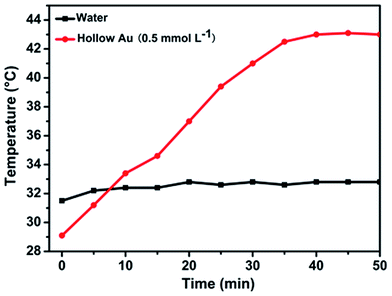 |
| Fig. 5 Temperature change of hAuNP suspension (0.5 mmol L−1) under laser irradiation (650 nm, 200 mW cm−2) for 40 min. DI water was used as control under the same conditions. | |
Bactericidal properties of hAuNPs-anti-E. coli-kana in vitro
The bactericidal effect of hAuNPs-anti-E. coli-kana incubated with E. coli, after laser irradiation, was measured by the number of colonies. Next, we did a control test under the same experimental conditions, measuring the viability of bacteria (blank), bacteria exposed to physical sterilization with hAuNPs under laser irradiation, the chemical drug sterilization by kanamycin, and the viability of bacteria with the hollow hybrid loaded with kanamycin under laser irradiation. The survival rate of the bacteria after treatment and the images of E. coli colony growth on the solid medium are shown in Table 1, with the corresponding histogram shown in Fig. 6. The viabilities of bare E. coli, those after exposure to hAuNPs under irradiation, after incubation with bare kanamycin, or after incubation with the hollow hybrid carrying kanamycin, were 100.00 ± 2.90%, 90.18 ± 2.80%, 87.27 ± 1.83%, and 50.36 ± 1.76%, respectively. The bacterial viability was significantly decreased to 50.36 ± 1.76% after incubating with the hAuNPs-anti-E. coli-kana and exposure to irradiation (650 nm, 200 mW cm−2, diameter 2 mm, 40 min). This result showed that a sterilizing rate of 49.64% was achieved for the hAuNPs-anti-E. coli-kana under irradiation. This clearly shows that there was a synergistic effect between hAuNPs-anti-E. coli-kana for each component of the bactericidal effect, producing greatly improved bactericidal properties against E. coli. In the case of the same bactericidal effect, owing to this synergistic effect, the amount of kanamycin was significantly reduced, which could reduce the possibility of drug resistance.
Table 1 The survival rate of E. coli in the presence of various conditions (irradiation at 650 nm laser, 40 min)
|
Bacteria (blank) |
Hollow Au (laser) |
Kanamycin |
Hollow Au-kana (laser) |
Culture medium |
LB |
LB |
LB |
LB |
Culture temperature |
37 °C |
37 °C |
37 °C |
37 °C |
Culture time |
20 h |
20 h |
20 h |
20 h |
Bacteria viability (%) |
100.00 ± 2.90 |
90.18 ± 2.80 |
87.27 ± 1.83 |
50.36 ± 1.76 |
Colony images |
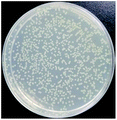 |
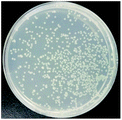 |
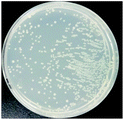 |
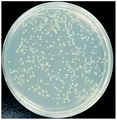 |
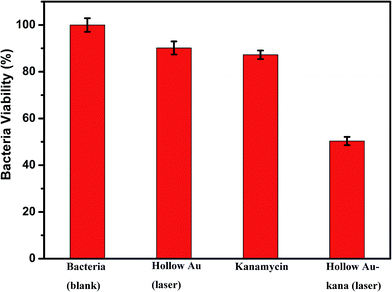 |
| Fig. 6 The corresponding histogram of the survival rate of E. coli under various conditions. | |
Here, using E. coli as a model, hAuNPs-anti-E. coli-kana with laser caused damage to the E. coli structures. After incubating E. coli with hAuNPs-anti-E. coli-kana for 4 h, the loss of interior structures and the formation of a large-scale, light-colored area were observed (Fig. 7B). The TEM images indicate that hAuNPs-anti-E. coli-kana could be internalized and located inside the cell wall of bacteria (red arrow in Fig. 7B), which seriously destroyed the cell wall and resulted in significant leakage of the cell contents (blue arrows in Fig. 7B).
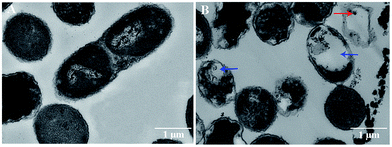 |
| Fig. 7 Morphology of E. coli without and with hAuNPs-anti-E. coli-kana treatment. TEM images of E. coli without (A), and with (B). | |
Conclusion
In this study, anti-E. coli-conjugated and kanamycin-loaded hAuNPs (hAuNPs-anti-E. coli-kana) were prepared for bacterial targeting and drug delivery. The system has multiple characteristics: dual-ligand modified hAuNPs targeting specific bacteria via the anti-E. coli, and synergistic bactericidal effects of the chemical drug and physical sterilization. In particular, the hollow interior of these NPs allows a significant increase in kanamycin loading. Compared with drug and photothermal treatment alone, the bactericidal rate of the combined treatment with the hAuNPs-anti-E. coli-kana was much higher. Thus, hAuNP-derived nanocarriers are a promising drug-targeting delivery system.
Acknowledgements
This work was financially supported by the National Natural Science Foundation of China (81171415) and the Chongqing Municipal Education Commission Science and Technology Research Project Foundation of China (KJ1400211).
Notes and references
- J. Davies, EMBO Rep., 2008, 9, S18–S21 CrossRef CAS PubMed.
- Y. Feng, W. Chen, Y. Jia, Y. Tian, Y. Zhao, F. Long, Y. Rui and X. Jiang, Nanoscale, 2016, 8, 13223–13227 RSC.
- P. Chairatana, T. Zheng and E. M. Nolan, Chem. Sci., 2015, 6, 4458–4471 RSC.
- H. K. Pedersen, V. Gudmundsdottir, H. B. Nielsen, T. Hyotylainen, T. Nielsen, B. A. Jensen, K. Forslund, F. Hildebrand, E. Prifti, G. Falony, E. Le Chatelier, F. Levenez, J. Dore, I. Mattila, D. R. Plichta, P. Poho, L. I. Hellgren, M. Arumugam, S. Sunagawa, S. Vieira-Silva, T. Jorgensen, J. B. Holm, K. Trost, H. I. T. C. Meta, K. Kristiansen, S. Brix, J. Raes, J. Wang, T. Hansen, P. Bork, S. Brunak, M. Oresic, S. D. Ehrlich and O. Pedersen, Nature, 2016, 535, 376–381 CrossRef CAS PubMed.
- K. Lewis and P. Strandwitz, Nature, 2016, 535, 501–502 CrossRef CAS PubMed.
- B. Hu, L. P. Zhang, X. W. Chen and J. H. Wang, Nanoscale, 2013, 5, 246–252 RSC.
- A. M. Schwartzberg, T. Y. Olson, C. E. Talley and J. Z. Zhang, J. Phys. Chem. B, 2006, 110, 19935–19944 CrossRef CAS PubMed.
- J. You, R. Zhang, G. Zhang, M. Zhong, Y. Liu, C. S. Van Pelt, D. Liang, W. Wei, A. K. Sood and C. Li, J. Controlled Release, 2012, 158, 319–328 CrossRef CAS PubMed.
- W. Lu, Q. Huang, G. Ku, X. Wen, M. Zhou, D. Guzatov, P. Brecht, R. Su, A. Oraevsky, L. V. Wang and C. Li, Biomaterials, 2010, 31, 2617–2626 CrossRef CAS PubMed.
- Z. V. Feng, I. L. Gunsolus, T. A. Qiu, K. R. Hurley, L. H. Nyberg, H. Frew, K. P. Johnson, A. M. Vartanian, L. M. Jacob, S. E. Lohse, M. D. Torelli, R. J. Hamers, C. J. Murphy and C. L. Haynes, Chem. Sci., 2015, 6, 5186–5196 RSC.
- I. H. El-Sayed, X. Huang and M. A. El-Sayed, Nano Lett., 2005, 5, 829–834 CrossRef CAS PubMed.
- I. H. Li, H. J. Zhou, J. X. Wang, D. H. Wang, R. X. Shen, X. L. Zhang, P. Jin and X. Y. Liu, Nanoscale, 2016, 8, 11907–11923 RSC.
- I. Yang, H. Shen, X. Zhang, Y. Tao, H. Xiang and G. Xie, Biosens. Bioelectron., 2016, 77, 1119–1125 CrossRef PubMed.
- I. C. Wu, A. R. Deokar, J. H. Liao, P. Y. Shih and Y. C. Ling, ACS Nano, 2013, 7, 1281–1290 CrossRef PubMed.
- I. Dai, X. Cao, K. F. Liu, C. X. Li, G. F. Zhang, L. H. Deng, C. L. Si, J. He and J. D. Lei, J. Mater. Chem. B, 2015, 3, 3754–3766 RSC.
- D. H. Tsai, S. Elzey, F. W. Delrio, A. M. Keene, K. M. Tyner, J. D. Clogston, R. I. Maccuspie, S. Guha, M. R. Zachariah and V. A. Hackley, Nanoscale, 2012, 4, 3208–3217 RSC.
- T. Huang, P. D. Nallathamby, D. Gillet and X. H. Xu, Anal. Chem., 2007, 79, 7708–7718 CrossRef CAS PubMed.
- J. Nicolas, S. Mura, D. Brambilla, N. Mackiewicz and P. Couvreur, Chem. Soc. Rev., 2013, 42, 1147–1235 RSC.
- E. Tegou, M. Magana, A. E. Katsogridaki, A. Ioannidis, V. Raptis, S. Jordan, S. Chatzipanagiotou, S. Chatzandroulis, C. Ornelas and G. P. Tegos, Biomaterials, 2016, 89, 38–55 CrossRef CAS PubMed.
- N. S. Noh, S. Lee, H. Kang, J. K. Yang, H. Lee, D. Hwang, J. W. Lee, S. Jeong, Y. Jang, B. H. Jun, D. H. Jeong, S. K. Kim, Y. S. Lee and M. H. Cho, Biomaterials, 2015, 45, 81–92 CrossRef PubMed.
- A. K. Singh, D. Senapati, S. Wang, J. Griffin, A. Neely, P. Candice, K. M. Naylor, B. Varisli, J. R. Kalluri and P. C. Ray, ACS Nano, 2009, 3, 1906–1912 CrossRef CAS PubMed.
- J. L. Tang, X. M. Jiang, L. M. Wang, H. Zhang, Z. J. Hu, Y. Liu, X. C. Wu and C. Y. Chen, Nanoscale, 2014, 6, 3670–3678 RSC.
- Z. B. Li, H. Huang, S. Y. Tang, Y. Li, X. F. Yu, H. Y. Wang, P. H. Li, Z. B. Sun, H. Zhang, C. L. Liu and P. K. Chu, Biomaterials, 2016, 74, 144–154 CrossRef CAS PubMed.
- B. K. Wang, X. F. Yu, J. H. Wang, Z. B. Li, P. H. Li, H. Wang, L. Song, P. K. Chu and C. Li, Biomaterials, 2016, 78, 27–39 CrossRef CAS PubMed.
- R. R. Xing, K. Liu, T. F. Jiao, N. Zhang, K. Ma, R. Y. Zhang, Q. L. Zou, G. H. Ma and X. H. Yan, Adv. Mater., 2016, 28, 3669–3676 CrossRef CAS PubMed.
- J. B. Qin, Z. Y. Peng, B. Li, K. C. Ye, Y. X. Zhang, F. K. Yuan, X. R. Yang, L. J. Huang, J. Q. Hu and X. W. Lu, Nanoscale, 2015, 7, 13991–14001 RSC.
- X. Y. Zhang, C. Y. Yang, J. P. Zhou and M. R. Huo, Small, 2016, 12, 3578–3590 CrossRef CAS PubMed.
- L. M. Wang, Q. Sun, X. Wang, T. Wen, J. J. Yin, P. Y. Wang, R. Bai, X. Q. Zhang, L. H. Zhang, A. H. Lu and C. Y. Chen, J. Am. Chem. Soc., 2015, 137, 1947–1955 CrossRef CAS PubMed.
- H. Q. Chen, B. Wang, D. Gao, M. Guan, L. N. Zheng, H. Ouyang, Z. F. Chai, Y. L. Zhao and W. Y. Feng, Small, 2013, 9, 2735–2746 CrossRef CAS PubMed.
- Y. Y. Zhao and X. Y. Jiang, Nanoscale, 2013, 5, 8340–8350 RSC.
- K. L. Knappenberger-J, A. M. Schwartzberg, A. M. Dowgiallo and C. A. Lowman, J. Am. Chem. Soc., 2009, 131, 13892–13893 CrossRef PubMed.
- C. Loo, A. Lowery, N. Halas, J. West and R. Drezek, Nano Lett., 2005, 5, 709–711 CrossRef CAS PubMed.
- K. J. Prashant, S. L. Kyeong, H. E. S. Ivan and A. E. S. Mostafa, J. Phys. Chem. B, 2006, 110, 7238–7248 CrossRef PubMed.
- L. M. Pan, J. N. Liu, Q. J. He and J. L. Shi, Adv. Mater., 2014, 26, 6742–6748 Search PubMed.
- Z. Z. Wang, Z. W. Chen, N. Gao, J. S. Ren and X. G. Qu, Small, 2015, 11, 4970–4975 CrossRef CAS PubMed.
- C. Chelsea, W. T. Alexander and C. B. Guillermo, Chem. Sci., 2016, 7, 2023–2029 RSC.
- F. Wang, Y. C. Wang, S. Dou, M. H. Xiong, T. M. Sun and J. Wang, ACS Nano, 2011, 5, 3679–3692 CrossRef CAS PubMed.
- H. Hillaireau and P. Couvreur, Cell. Mol. Life Sci., 2009, 66, 2873–2896 CrossRef CAS PubMed.
- G. Sahay, D. Y. Alakhova and A. V. Kabanov, J. Controlled Release, 2010, 145, 182–195 CrossRef CAS PubMed.
- X. K. Zhang, L. J. Meng, Q. H. Lu, Z. F. Fei and P. J. Dyson, Biomaterials, 2009, 30, 6041–6047 CrossRef CAS PubMed.
Footnote |
† Electronic supplementary information (ESI) available. See DOI: 10.1039/c7ra00509a |
|
This journal is © The Royal Society of Chemistry 2017 |
Click here to see how this site uses Cookies. View our privacy policy here.