DOI:
10.1039/C6RA28625A
(Paper)
RSC Adv., 2017,
7, 10266-10277
Antiviral activity and mechanism of gossypols: effects of the O2˙− production rate and the chirality†
Received
23rd December 2016
, Accepted 16th January 2017
First published on 7th February 2017
Abstract
(−)-Gossypol displayed an obviously higher antiviral activity against the tobacco mosaic virus (TMV) than (+)-gossypol, whereas the anti-TMV activity of (−)-gossypol Schiff bases is not significantly higher than (+)-gossypol Schiff bases. A mechanism study indicated that these gossypol compounds could neither inhibit the multiplication of TMV nor induce the systemic acquired resistance of tobacco plants. However, gossypol compounds with high anti-TMV activities could induce the accumulation of reactive oxygen species (ROS) in tobacco leaves, and this result is in accordance with the characteristics of extreme resistance featuring a necessary early and rapid ROS (O2˙− or H2O2) accumulation in plants. Further study showed that, the anti-TMV activities of the test compounds decreased synchronously when the O2˙− accumulation was reduced by superoxide dismutase (SOD). However, when the H2O2 accumulation was suppressed by hydrogen peroxidase (CAT), the anti-TMV activities did not change. Therefore, compared with the H2O2 accumulation in gossypol compounds-treated tobacco leaves, the O2˙− accumulation is a key factor for the development of the anti-TMV activities of those compounds, and their anti-TMV mechanism belonged to their extreme resistance. Further study showed that the O2˙− accumulation in the (−)-gossypol-treated tobacco leaves was mainly from the tobacco itself, whereas the same O2˙− accumulation in the gossypol Schiff base-treated tobacco leaves was at least partially related to the O2˙− produced by the compound. Accordingly, gossypol compounds achieved their anti-TMV activities by stimulating O2˙− accumulation in the tobacco, which was induced either by the O2˙− production or by the chirality of the gossypol compounds, and this result could also explain why (−)-gossypol or gossypol Schiff bases displayed higher anti-TMV activities than (+)-gossypol.
Introduction
Natural products are characterized by their chemical diversity, new mechanisms, and decomposing abilities, and they are a good source of a range of bioactive structures, including anti-virus compounds.1–3 Gossypol, a polyphenolic natural product, has displayed various anti-virus properties; however, most reports on gossypol's anti-virus ability are about its anti-virus abilities to enveloped viruses such as HIV-1,4 H5N1,4a HSV-2,5 and the influenza virus.6 Among the various research groups, Yang and Tien's groups demonstrated that gossypol compounds could target the enveloped protein of HIV-1 and H5N1 to prevent the viral entry.4a–c Some reports even speculated that gossypol was merely active against enveloped viruses rather than against non-enveloped viruses.4a However, recently, the tobacco mosaic virus (TMV), a typical non-enveloped virus, was reported to be an exception, whereby its typical necrosis symptom in tobacco leaves can be suppressed by racemic gossypol.7 Therefore, there is growing interest in understanding the mechanism of the anti-TMV activity of gossypol in the field of anti-virus research.
Our previous study7c indicated that the anti-TMV activities of (±)-gossypol Schiff bases with D-amino acids were positively related to their O2˙− production rates; however, the influence of chirality still needed further investigation. There are few reports in the literature about the anti-TMV activities of optical gossypol, and it is not yet clear which optical isomer in racemic gossypol plays a main role in its anti-TMV activity, and also the mechanism of anti-TMV activity is still unknown. Therefore, in this study, (+) and (−)-gossypol and a series of their derivatives were synthesized from (±)-gossypol, and their anti-TMV activities were tested, and the mechanism of their anti-TMV activities was explored.
Experimental
Materials and instruments
All reagents were purchased from commercial sources and were used as received. All anhydrous solvents were dried and purified by standard techniques just before use. Reaction progress was monitored by thin-layer chromatography (TLC) on silica gel GF-254, with detection by UV. 1H NMR and 13C NMR spectra were recorded on a Bruker Ascend 300/400 MHz spectrometer. Chiral HPLC analysis was performed on a Shimadzu LC20AT with a Phenomenex Lux 5 μm cellulose-1 analytical column (4.6 × 250 mm) Optical rotations were recorded on an Autopol IV instrument. The absorbance was obtained using a BioTek Synergy 4 enzyme-labeled instrument or Nanodrop 2000.
General procedure for the synthesis of L-Trp-OMe-(−)-gossypol or L-Trp-OMe-(+)-gossypol8a. A solution of L-tryptophan methyl ester hydrochloride (10.16 g, 40 mol), racemic gossypol (10.36 g, 20 mol), and NaOH (1.6 g, 40 mol) in ethanol (200 mL) was stirred at room temperature for 0.5 h. Then, the mixture was heated to reflux. The reaction was monitored by TLC. After 2 h, the reaction slurry was cooled to room temperature and a yellow precipitate was obtained via filtration. This yellow precipitate was then washed with ethanol, and dried under reduced pressure to afford 8.91 g (97%) of L-Trp-OMe-(−)-gossypol. The mother liquid was concentrated to dryness. The residue was extracted with chloroform by stirring the suspension, leaving behind a white insoluble inorganic salt. The chloroform extract was concentrated under reduced pressure to obtain 8.68 g (94%) of L-Trp-OMe-(+)-gossypol.
General procedure for the synthesis of (+) or (−)-gossypol8a. To a solution of L-Trp-OMe-(−)-gossypol or L-Trp-OMe-(+)-gossypol (6.0 g, 6.25 mmol) in ether (200 mL), acetic acid (55 mL) and 2.5 mL of hydrochloric acid (37%) were added in sequence. After refluxing for 4 h, the reaction mixture was cooled to room temperature and a white precipitate (2.4 g, L-tryptophan methyl ester hydrochloride) was filtered. The filtrate was washed with water several times until the water phase showed neutrality (pH ≈ 7), and then it was dried over anhydrous MgSO4 for 1 h. After filtration, this dried filtrate was concentrated to dryness under reduced pressure. The residue was dissolved in 100 mL solvent [Et2O/PE (8
:
1)]. This solution was then concentrated to 30 mL, and a yellow precipitate was formed. This yellow precipitate 2.54 g (75%) was (−)-gossypol or (+)-gossypol and could be obtained via filtration.
General procedure for the synthesis of (+) or (−)-gossypol Schiff bases8b. A mixture of (+)-gossypol or (−)-gossypol (0.446 g, 0.86 mmol) and an amino-containing compound [compounds 1–4, 9–10, 1.72 mmol] in ethanol (20 mL) was stirred and heated at reflux for 2–3 h, with the progress of the reaction being monitored by TLC. When the reaction reached the end, the mixture was cooled down to room temperature. The resulting solid was filtered and washed with ethanol (20 mL) three times to give the desired gossypol derivatives.A mixture of (+)-gossypol or (−)-gossypol (0.446 g, 0.86 mmol), NaOH (0.068 g, 1.72 mmol) and an amino-containing compound [compounds 5–8, 1.72 mmol] in ethanol (20 mL) was stirred and heated at reflux for 2–3 h, with the progress of the reaction being monitored by TLC. When the reaction reached the end, the mixture was cooled down to room temperature. The resulting solid was filtered and washed with ethanol (20 mL) three times to give the desired gossypol derivatives.
General procedure for determination of the O2˙− production rate of (+) and (−)-gossypol and their Schiff bases. The O2˙− production rates of (+) and (−)-gossypol and their Schiff bases were measured by the method described in our previous work.7c
Confirmation of the influences of the gossypol compounds to TMV multiplication in tobacco. The TMV virus solution (5.5 × 10−2 μg mL−1 in water) was mixed with a gossypol compound solution [500 μg mL−1 in a mixed solvent (DMF
:
H2O = 1
:
10)] of the same volume for 50 min. Twenty fresh leaves from healthy tobacco plants (5–6 leaf stage) were cut into two halves along the main vein, and the left or right halves were separately collected and weighed. Then, the above TMV mixture was inoculated on the left halves of the tobacco leaves by the juice-leaf rubbing method, whereas the right halves of the leaves were inoculated with a mixture of one volume of solvent (DMF
:
H2O = 1
:
10) and one volume of TMV virus (5.5 × 10−2 μg mL−1 in water) for 50 min, as a control or mock. The leaves were then cultured at 25 °C under 100% humidity. After 72 h, the TMV was extracted separately from the left halves or right halves according to Leberman's method.9 The TMV extracted from per 10 g leaves was dissolved in 100 μL phosphate buffer (0.1 mol L−1, pH 7.0) to test the concentration. |
Virus concn = (A260 × dilution ratio)/E0.1%,260 nm1 cm
| (1) |
(A260-compd refers to the absorbance of TMV solution extracted from tobacco leaves treated by the compound at 260 nm.)The effects of those compounds to TMV multiplication can be reflected by the relative concentration of TMV, which can be obtained from eqn (2).
|
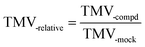 | (2) |
[TMV
-compd refers to the concentration of TMV extracted from the half tobacco leaf treated with a mixture of solvent (DMF
![[thin space (1/6-em)]](https://www.rsc.org/images/entities/char_2009.gif)
:
![[thin space (1/6-em)]](https://www.rsc.org/images/entities/char_2009.gif)
H
2O = 1
![[thin space (1/6-em)]](https://www.rsc.org/images/entities/char_2009.gif)
:
![[thin space (1/6-em)]](https://www.rsc.org/images/entities/char_2009.gif)
10), the compound, and TMV; TMV
-mock refers to the other half leaf treated by a mixture of one volume of solvent (DMF
![[thin space (1/6-em)]](https://www.rsc.org/images/entities/char_2009.gif)
:
![[thin space (1/6-em)]](https://www.rsc.org/images/entities/char_2009.gif)
H
2O = 1
![[thin space (1/6-em)]](https://www.rsc.org/images/entities/char_2009.gif)
:
![[thin space (1/6-em)]](https://www.rsc.org/images/entities/char_2009.gif)
10) and one volume of TMV solution (5.5 × 10
−2 μg mL
−1 in water)].
Because the TMV virus concentration can be calculated by eqn (1), eqn (2) can be converted into eqn (3).
|
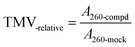 | (3) |
[
A260-compd refers to the absorbance of TMV solution at 260 nm, which was extracted from tobacco leaves treated with a mixture of solvent (DMF
![[thin space (1/6-em)]](https://www.rsc.org/images/entities/char_2009.gif)
:
![[thin space (1/6-em)]](https://www.rsc.org/images/entities/char_2009.gif)
H
2O = 1
![[thin space (1/6-em)]](https://www.rsc.org/images/entities/char_2009.gif)
:
![[thin space (1/6-em)]](https://www.rsc.org/images/entities/char_2009.gif)
10), compound, and TMV;
A260-blank refers to the absorbance of TMV solution at 260 nm, which was extracted from tobacco leaves treated by a mixture of one volume of solvent (DMF
![[thin space (1/6-em)]](https://www.rsc.org/images/entities/char_2009.gif)
:
![[thin space (1/6-em)]](https://www.rsc.org/images/entities/char_2009.gif)
H
2O = 1
![[thin space (1/6-em)]](https://www.rsc.org/images/entities/char_2009.gif)
:
![[thin space (1/6-em)]](https://www.rsc.org/images/entities/char_2009.gif)
10) and one volume of TMV solution (5.5 × 10
−2 μg mL
−1 in water)].
Determination of the influences of the gossypol compounds to the assembly of tobacco mosaic virus
TMV-coat protein (TMV-CP) was prepared by the acetic acid method;10 while TMV-RNA was isolated by the method reported by Zimmern.11 Before the reaction, the TMV-RNA (0.15 mg mL−1) was mixed with specific compounds (500 μg mL−1) for 15 s, then the fresh 20 s disk (1 mg mL−1) of TMV-CP [TMV-RNA/TMV-CP = 1
:
3 (wt/wt)] was added [the 20 s disk was prepared by incubating the TMV-CP (20 mg mL−1) in 0.1 M phosphate buffer (pH 7.0) at 20 °C for 12–15 h] to this solution. TMV assembly was carried out in the same buffer at 20 °C. After 8 h, micrococcal nuclease (1 K unit per mL) and CaCl2 (0.8 mmol L−1) were directly added into the assembled mixture. The unassembled RNA in the above solution could be decomposed by the micrococcal nuclease, but the RNA in the assembled TMV could not be affected, so after adding the micrococcal nuclease to this solution, the amount of RNA extracted from this solution could be positively correlated to the assembling ability, and negatively correlated to the interaction between the compound and TMV-CP or TMV-RNA. After incubating this mixture at 25 °C for 20 min, EDTA (5 mmol L−1) was added to terminate the nuclease reaction. RNA was then extracted from the above solution, followed by precipitation with ethanol three times at −20 °C. The RNA extracted from the assembled intermediates was analyzed by polyacrylamide gel electrophoresis.12
Influence of the compounds to tobacco
Determination of the influences of the compounds to ROS and its related enzymes in tobacco. To identify the influences of those compounds to tobacco, changes of the ROS (indicating O2˙− and H2O2 in this paper) or the related enzymes (SOD, CAT) activities in the tobacco leaves were determined. Because the primary data of ROS or the activities of enzymes in different tobacco leaves are different, it became a key issue in this experiment to eliminate this difference.We noticed that the anti-TMV activity of the compounds could be tested by the half-leaf method, whereby a piece of healthy tobacco leaf (5–6 leaves stage) was inoculated with TMV by the juice-leaf rubbing method, then half of this tobacco leaf was then treated by pure water or pure solvent (DMF
:
H2O = 1
:
10), while the other half was treated by the compound (500 μg mL−1). After 2–3 days, the anti-TMV activity data was acquired using eqn (4).13
|
 | (4) |
[The primary data refers to the number of necrotic lesions on the half leaf treated by the compound, while the mock refers to the number of necrotic lesions on the half leaf treated by pure water or pure solvent (DMF
![[thin space (1/6-em)]](https://www.rsc.org/images/entities/char_2009.gif)
:
![[thin space (1/6-em)]](https://www.rsc.org/images/entities/char_2009.gif)
H
2O = 1
![[thin space (1/6-em)]](https://www.rsc.org/images/entities/char_2009.gif)
:
![[thin space (1/6-em)]](https://www.rsc.org/images/entities/char_2009.gif)
10)].
In this half-leaf method, the mock was used as a reference to eliminate the system error caused by different leaves. On the basis of the half-leaf method, we designed a method to determine the influence of the gossypol compounds on the ROS and of their related enzymes (SOD, CAT) in tobacco leaves.
A piece of tobacco leaf was treated one half by pure solvent (DMF
:
H2O = 1
:
10) or pure double-distilled water [our experimental results showed that the pure solvent (DMF
:
H2O = 1
:
10) and the pure double-distilled water showed no distinction in this experiment] and the other half by a specific gossypol Schiff base solution (DMF
:
H2O = 1
:
10, 500 μg mL−1). After set times (2, 5, 8, 12, 18, 24 h), each half of the tobacco leaf was washed by saline, and the tissues were homogenated (9 mL saline to 1 g fresh tissue), centrifuged, and then the supernatant was taken for measurement of the protein concentration, ROS, and ROS-related dismutase. The data acquired from the half leaf treated with the pure solvent was regarded as a reference. The relative data used in this paper were obtained by dividing the primary data by the reference.
|
 | (5) |
[The primary data refers to the ROS or related dismutase in the compound-treated half leaf, while the reference refers to that on the pure solvent (DMF
![[thin space (1/6-em)]](https://www.rsc.org/images/entities/char_2009.gif)
:
![[thin space (1/6-em)]](https://www.rsc.org/images/entities/char_2009.gif)
H
2O = 1
![[thin space (1/6-em)]](https://www.rsc.org/images/entities/char_2009.gif)
:
![[thin space (1/6-em)]](https://www.rsc.org/images/entities/char_2009.gif)
10)-treated half leaf. At least three leaves per pot were used to acquire those data at each specified point-in-time].
The results showed that although the primary data of ROS or related dismutase in different leaves varied greatly (ESI, Table S1†), the relative data obtained from eqn (5) were almost around the same level, indicating that the relative data eliminated the influence of different leaves and thus this could be a reasonable index to reflect the influences of compounds to ROS and their related enzymes in tobacco.
Determination of the influences of the compounds, SOD, or CAT to ROS and the related enzymes in TMV-inoculated tobacco leaves. The middle leaves of the tobacco were chosen for this experiment. The right half of a tobacco leaf was treated by pure solvent (DMF
:
H2O = 1
:
10) or pure double-distilled water, while the left half was treated by the solution of a specific gossypol Schiff base (DMF
:
H2O = 1
:
10, 500 μg mL−1). Then, at 24 h, both the right half and left half of the tobacco leaves were inoculated by the TMV. At 26, 28, 32, 40, 48, 56, 64, and 72 h (with at least three leaves used to acquire those data at each specified point-in-time), each half of the tobacco leaves was washed by saline, and the tissue homogenate was prepared in a specific proportion (9 mL saline to 1 g fresh tissue). After homogenizing and centrifuging, the supernatant was taken for measurement of the protein concentration, ROS, and ROS-related dismutases. The relative data used in the paper were obtained according to eqn (5).
Anti-TMV assay
The anti-TMV activity of the compounds was tested by our previously reported method14 and confirmed by use of the half-leaf method.
Results and discussion
Synthesis of L-Trp-OMe-(−)-gossypol and L-Trp-OMe-(+)-gossypol
The diastereomeric excess (dr) of the L-Trp-OMe-(−)-gossypol or L-Trp-OMe-(+)-gossypol were identified by 1H NMR analysis, as clearly described in our previously reported article.7c In order to obtain the desirable high dr products, several elements which may influence their dr were investigated. We found that during the preparation of L-Trp-OMe-(−)-gossypol, it was favorable to use methanol or ethanol as the reaction solvent. Using other solvents, such as isopropanol or tetrahydrofuran, could not generate the ideal product with a high dr. It was also essential to keep the amount of NaOH between 2 and 2.1 equiv. (refers to 1 equiv. gossypol). The temperature and reaction time did not affect the dr of the product. We also found that the dr of L-Trp-OMe-(−)-gossypol could also be improved by beating the crude product in ethanol. The L-Trp-OMe-(+)-gossypol could be further purified by recrystallization from chloroform.
Optimization of the synthesis of (−)-gossypol or (+)-gossypol
During the preparation of (+) or (−)-gossypol, it is important to keep the acetic acid and hydrochloric acid in a specific proportion [acetic acid
:
hydrochloric acid (37%) = 22
:
1 mL]. The results showed that if their ratios were too far from this, L-tryptophan methyl ester would mix in (−)-gossypol. We also found that, compared with pure Et2O or PE, using Et2O/PE (8
:
1) as the recrystallization solvent was more favorable to improve the enantiomeric excess (ee) of (+) or (−)-gossypol.
The influence of chirality on the anti-TMV activities of optical gossypol compounds
Our previous study7c indicated that the O2˙− production rates of D-amino acids (±)-gossypol Schiff bases were positively related to their anti-TMV activities. If the O2˙− production rate was the only influencing factor to the anti-TMV activities of gossypol compounds, (−) and (+)-gossypol, with the same O2˙− production rate, should display the same anti-TMV activity. However, as shown in Table 1, (−)-gossypol displayed a higher anti-TMV activity than (+)-gossypol and ribavirin.
Table 1 The anti-TMV activities of (+) and (−)-gossypol and their Schiff bases
Compd |
Concn (μg mL−1) |
In vitro curative effecta (%) |
In vivo activity |
Inactivation effecta (%) |
Curative effecta (%) |
Protection effecta (%) |
Average of three replicates. All the results are expressed as mean ± SD. Compound 3* refers to adding 0.3 equiv. of NaOH to the solution (DMF : H2O = 1 : 10) of compound 3. Compound 4* refers to adding 0.3 equiv. of NaOH to the solution (DMF : H2O = 1 : 10) of compound 4. |
(−)-Gossypol |
500 |
48 ± 4 |
63 ± 5 |
55 ± 6 |
60 ± 4 |
100 |
16 ± 5 |
20 ± 4 |
30 ± 6 |
28 ± 4 |
(+)-Gossypol |
500 |
32 ± 5 |
23 ± 5 |
19 ± 4 |
28 ± 6 |
100 |
0 |
9 ± 5 |
6 ± 5 |
12 ± 4 |
1 |
500 |
55 ± 6 |
55 ± 2 |
61 ± 3 |
48 ± 2 |
100 |
26 ± 6 |
22 ± 5 |
28 ± 4 |
18 ± 1 |
2 |
500 |
38 ± 5 |
47 ± 4 |
43 ± 5 |
45 ± 8 |
100 |
15 ± 4 |
13 ± 8 |
11 ± 7 |
9 ± 6 |
3 |
500 |
40 ± 8 |
43 ± 5 |
47 ± 4 |
37 ± 6 |
100 |
16 ± 5 |
20 ± 2 |
9 ± 4 |
12 ± 4 |
3*b |
500 |
49 ± 6 |
58 ± 5 |
63 ± 4 |
51 ± 8 |
100 |
36 ± 5 |
32 ± 4 |
28 ± 3 |
25 ± 3 |
4 |
500 |
22 ± 8 |
35 ± 4 |
37 ± 6 |
32 ± 4 |
100 |
0 |
10 ± 5 |
0 |
8.8 ± 5 |
4*b |
500 |
45 ± 7 |
55 ± 3 |
48 ± 8 |
61 ± 4 |
100 |
28 ± 3 |
29 ± 4 |
31 ± 3 |
35 ± 8 |
5 |
500 |
61 ± 5 |
62 ± 3 |
59 ± 1 |
69 ± 3 |
100 |
27 ± 2 |
42 ± 3 |
37 ± 2 |
29 ± 3 |
6 |
500 |
58 ± 4 |
68 ± 2 |
51 ± 6 |
64 ± 4 |
100 |
30 ± 5 |
35 ± 3 |
12 ± 4 |
22 ± 7 |
7 |
500 |
46 ± 5 |
57 ± 2 |
53 ± 3 |
55 ± 3 |
100 |
20 ± 3 |
23 ± 2 |
26 ± 2 |
19 ± 3 |
8 |
500 |
43 ± 6 |
52 ± 2 |
50 ± 5 |
47 ± 6 |
100 |
13 ± 3 |
17 ± 3 |
15 ± 3 |
13 ± 4 |
9 |
500 |
35 ± 5 |
43 ± 3 |
38 ± 3 |
47 ± 5 |
100 |
0 |
9 ± 4 |
14 ± 2 |
6 ± 4 |
10 |
500 |
30 ± 5 |
36 ± 6 |
32 ± 5 |
40 ± 4 |
100 |
0 |
0 |
0 |
7.5 ± 5 |
Ribavirin |
500 |
38 ± 5 |
39 ± 8 |
40 ± 5 |
36 ± 8 |
100 |
12 ± 5 |
14 ± 3 |
10 ± 2 |
10 ± 4 |
Antofine |
500 |
59 ± 5 |
65 ± 5 |
62 ± 4 |
58 ± 8 |
100 |
36 ± 5 |
32 ± 5 |
28 ± 5 |
34 ± 5 |
The only difference between (+) and (−)-gossypol was their chirality, so the chirality of the gossypol should be another influencing factor to their anti-TMV activities besides their O2˙− production rates, and it was found that (−)-gossypol in (±)-gossypol was the main contributor to the anti-TMV activity of (±)-gossypol.
The anti-TMV activities of (±)-gossypol Schiff bases were higher than those of (±)-gossypol,7 so if (−)-gossypol Schiff bases were also a main contributor to the anti-TMV activity of (±)-gossypol Schiff base, the (−)-gossypol Schiff bases should display higher anti-TMV activities than (−)-gossypol. However, the (−)-gossypol Schiff bases (compounds 1, 3, 5, 7, and 9), which showed higher O2˙− production rates than (−)-gossypol (ESI, Fig. S13†), did not show obviously higher anti-TMV activities than (−)-gossypol (Table 1). These results indicated that, though changing (−)-gossypol to the corresponding Schiff bases improved their O2˙− production rates, this change did not lead to a combined effect of chirality and O2˙− production, which we thought might offer a higher anti-TMV activity than that of (−)-gossypol.
On the contrary, as it is also shown in Table 1, the anti-TMV activities of (+)-gossypol Schiff bases (compounds 2, 4, 6, 8, and 10) were higher than that of (+)-gossypol. These results demonstrate that changing (+)-gossypol to its Schiff bases can not only enhance their O2˙− production rates, but also improve their anti-TMV activities.
So according to these results, we suspected that, for the anti-TMV activity of optical gossypols, the chirality is an influencing factor, but for those of optical gossypol Schiff bases, the chirality is not so important as the O2˙− production rates.
To better understand these results, the anti-TMV mechanism was further explored (Fig. 1).
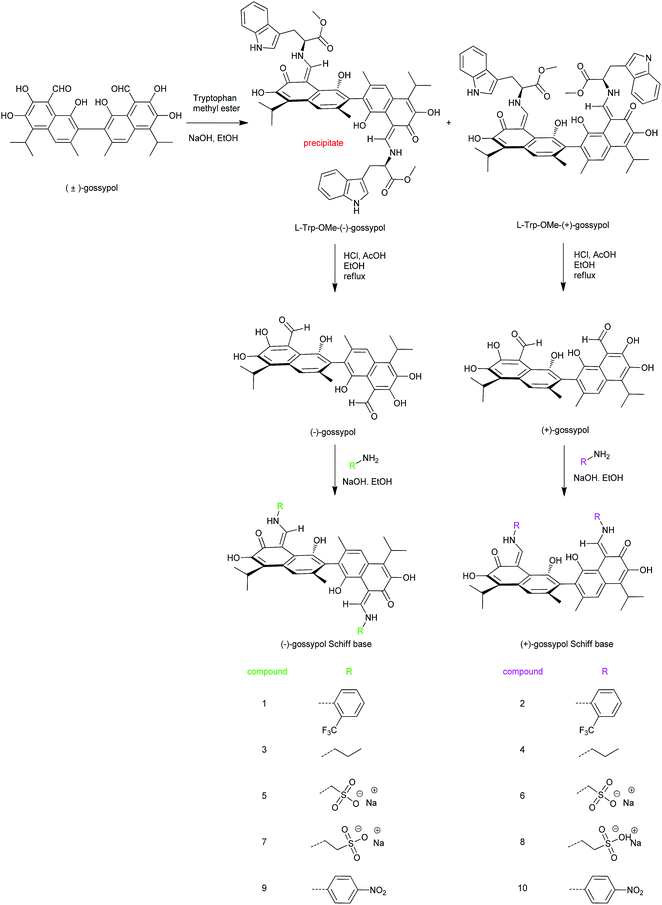 |
| Fig. 1 General procedure for the synthesis of (+) and (−)-gossypol and some of their Schiff bases and their structures. | |
Anti-TMV mechanism study
The influence of the gossypol compounds on TMV multiplication. One potential explanation for the above-described phenomenon was that (−)-gossypol and optical gossypol Schiff bases might have higher ability in inhibiting the TMV multiplication than (+)-gossypol. To justify this assumption, further experiments on the influence of (+), (−)gossypol and their related Schiff bases on TMV multiplication in tobacco were conducted. However, it turned out that the multiplication of TMV in tobacco leaves was not be affected by these gossypol compounds, but could be obviously inhibited by antofine (Fig. 2), an alkaloid which has been demonstrated to inhibit the TMV multiplication via targeting TMV-RNA.15 If a compound could interact with either the TMV-CP or TMV-RNA, their assembly would be inhibited. To further confirm this result, the self-assemblies of TMV-CP (20 s disk) and TMV-RNA in the presence of (+)-gossypol or (−)-gossypol, compound 1 or compound 2, were performed. As expected, the results showed that the self-assembly of TMV could not be inhibited by these gossypol compounds (ESI, Fig. S14†).
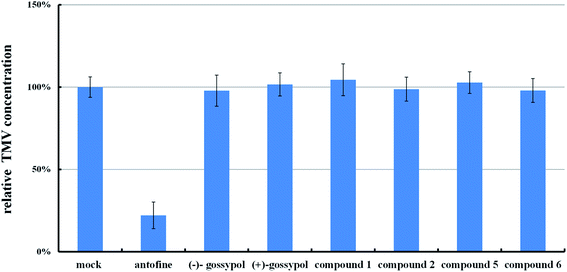 |
| Fig. 2 Relative TMV amount in tobacco leaves treated by a mixed solvent (DMF : H2O = 1 : 10), antofine [500 μg mL−1, in solution (DMF : H2O = 1 : 10)] or specific compounds [500 μg mL−1, in solution (DMF : H2O = 1 : 10)]. The relative TMV amount in tobacco treated by the mixed solvent was referred to as the mock. The means of three independent experiments ± SD are shown. | |
From those results, we came to the conclusion that the suppression of the typical necrotic symptom induced by TMV in gossypol-treated tobacco leaves was not related to the suppression of TMV multiplication.
Systemic acquired resistance vs. extreme resistance. Another possible explanation for their anti-TMV activities was that (+) and (−)-gossypol and their corresponding Schiff bases may have different abilities in stimulating the defense system of tobacco.However, our study confirmed that these compounds could not elicit the traditional systemic acquired resistance of tobacco. Therefore, their mechanism should be different with that of a systemic acquired resistance. In 1989, Doke's group reported that the generation of O2˙− during TMV infection might correlate to the necrotic lesion in tobacco, and adding merely SOD could suppress the necrotic lesion.16 Recently, Király's group reported that applying a low concentration of H2O2 to tobacco leaf could suppress necrotic disease symptom caused by TMV, without the inhibition of TMV multiplication.17 They further demonstrated that this mechanism might belong to the extreme resistance17c (refers to a rapid symptomless plant resistance to virus infections), and that during the development of extreme resistance,18 an early and rapid ROS (O2˙−, H2O2) accumulation in the plant seemed crucial. Because (+) and (−)-gossypol and their Schiff bases cannot suppress the TMV multiplication, we speculate that their mechanism of anti-TMV activities may also belong to one of extreme resistance.
Influence of optical gossypol and their Schiff bases to the ROS and their related enzymes in TMV-inoculated tobacco leaves. To verify this hypothesis, investigations about the relationship of ROS (O2˙− and H2O2), their related dismutases, and anti-TMV activities were conducted.As shown in Fig. 3, S15a and b (ESI†), (+)-gossypol with a lower anti-TMV activity could neither stimulate the production of ROS, nor increase the activities of SOD or CAT in the tobacco leaves. After TMV inoculation, (+)-gossypol-treated tobacco leaves underwent an O2˙− burst (a dramatic increase in a short time), the same as that treated by mixed solvent [mock, (DMF
:
H2O = 1
:
10)], which has no anti-TMV activity.
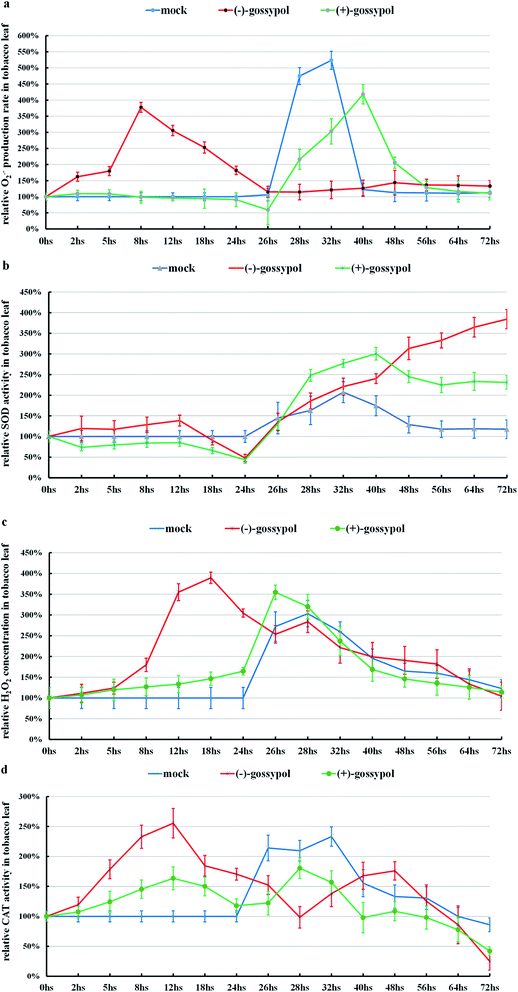 |
| Fig. 3 (a) The change of O2˙− production rates in tobacco leaves. (b) The change of SOD activities in tobacco leaves. (c) The change of H2O2 concentrations in tobacco leaves. (d) The change of CAT activities in tobacco leaves. A mixed solvent [mock, (DMF : H2O = 1 : 10)], (−)-gossypol or (+)-gossypol was sprayed onto the tobacco leaves at the beginning, separately, and then TMV was inoculated on these tobacco leaves at the time of 24 h. The means of three independent experiments ± SD are shown. | |
In contrast, (−)-gossypol with a high anti-TMV activity (Fig. 3) could stimulate the ROS production in the tobacco leaves, accompanied by increased activities of SOD and CAT. Also, (−)-gossypol-pretreated tobacco leaves showed an obviously higher SOD activity and had no obvious O2˙− burst after TMV inoculation.
Further studies of the Schiff bases of (−) and (+)-gossypol, which showed high anti-TMV activities, were similarly performed. The response of tobacco to (+)-gossypol derivatives (compound 2 and compound 6) was much different from that of (+)-gossypol (Fig. 4, S15c and d in ESI†), but similar to that of (−)-gossypol. This finding coincides with the fact that (+)-gossypol Schiff bases and (−)-gossypol had much higher anti-TMV activities than (+)-gossypol.
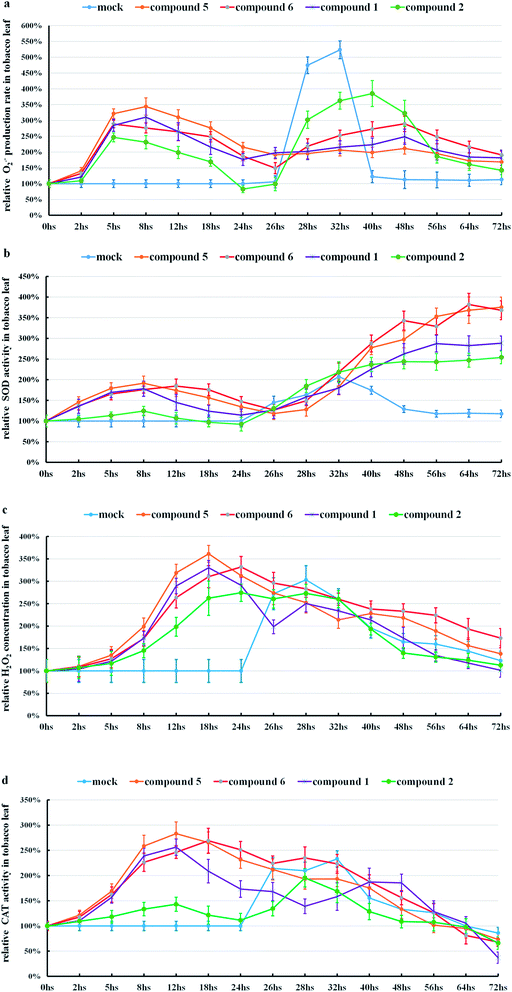 |
| Fig. 4 (a) The change of O2˙− production rates in tobacco leaves. (b) The change of SOD activities in tobacco leaves. (c) The change of H2O2 concentrations in tobacco leaves. (d) The change of CAT activities in tobacco leaves. A mixed solvent [mock, (DMF : H2O = 1 : 10)], compound 1 (500 μg mL−1), compound 2 (500 μg mL−1), compound 5 (500 μg mL−1), or compound 6 (500 μg mL−1) was sprayed onto the tobacco leaves, separately, and then TMV was inoculated onto these tobacco leaves at the time of 24 h. The means of three independent experiments ± SD are shown. | |
Comparing those results, we found that those gossypol compounds with high anti-TMV activities can stimulate the ROS production of tobacco leaves, accompanied by enhanced SOD or CAT activities in tobacco leaves. Those changes seem related to the development of the anti-TMV ability of tobacco leaves (suppression of the typical necrotic symptom induced by TMV).
On the contrary, the O2˙− burst in (+)-gossypol compounds or the mixed solvent (DMF
:
H2O = 1
:
10)-treated tobacco leaves, after TMV inoculation, was not favorable but rather was harmful to their anti-TMV activity, and this O2˙− burst should be classified as an oxidative stress, which is related to the typical necrotic symptom induced by TMV.16 After TMV inoculation, the SOD activities in those tobacco leaves treated by high anti-TMV gossypol compounds were higher than those treated by low anti-TMV gossypol compounds or by double-distilled water.
Confirmation of the importance of ROS accumulation. What happened in those tobacco leave when gossypol compounds were applied to those tobacco? Is the ROS (O2˙− or H2O2) accumulation in the tobacco leaves crucial to the development of the anti-TMV activity? If so, which of ROS, O2˙−, or H2O2, is more important?To answer those questions, we speculated that the addition of (−)-gossypol can perturb redox homeostasis in tobacco leaves. This perturbation could lead to an oxidative stress in the tobacco. In order to reduce the hurt of oxidative stress, tobacco would produce more ROS dismutase to reduce the ROS. This change could also improve their abilities to respond to an emergency, for example, TMV infection. So, before the TMV inoculation, the ROS accumulation in tobacco leaves could be crucial to the development of their anti-TMV activities.
If this speculation was correct, for instance, when additional ROS dismutase was added before applying (−)-gossypol to the tobacco leaves, the ROS accumulation induced by (−)-gossypol could be suppressed, and as a result, their anti-TMV activities should be reduced. But, if additional ROS dismutase was added after TMV inoculation, their ROS accumulation induced by (−)-gossypol should not be suppressed, and its anti-TMV activity might be the sum of (−)-gossypol and ROS dismutase. Since SOD and CAT themselves also have anti-TMV activity,4c,16 on this condition, its anti-TMV activity should increase. But for (+)-gossypol, because the (+)-gossypol itself could not induce the ROS accumulation in tobacco leaves, adding the ROS dismutase (before TMV inoculation or after inoculation) should not reduce but rather should increase its anti-TMV activity.
However, as shown in Fig. 5a, adding CAT (480 unit per mL) to (−) or (+)-gossypol-treated tobacco leaves did not obviously influence their anti-TMV activities. The same trend happened when CAT (480 unit per mL) was added to Schiff base-treated tobacco leaves (ESI, Fig. S15†). So the H2O2 accumulation in tobacco leaves before TMV inoculation (CAT-1) is not a key factor to the formation of their anti-TMV activities.
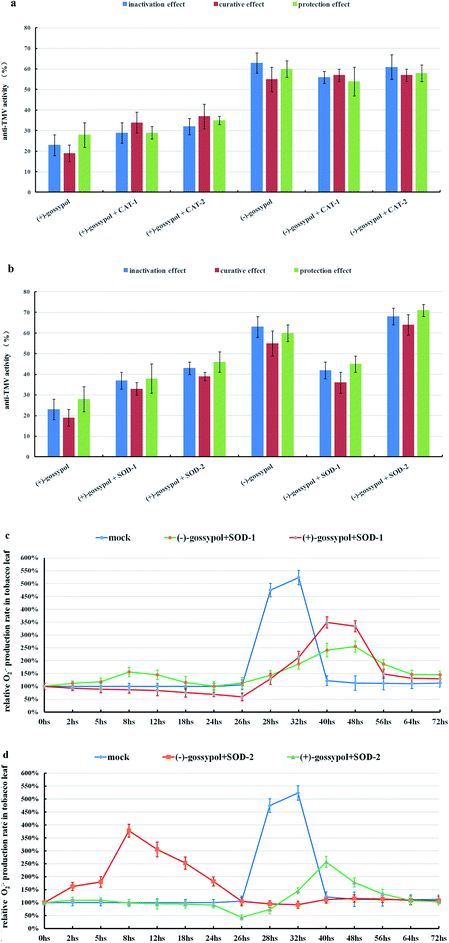 |
| Fig. 5 (a) The anti-TMV activities of (−)-gossypol (500 μg mL−1), (−)-gossypol (500 μg mL−1) + CAT-1 (480 unit per mL), (−)-gossypol (500 μg mL−1) + CAT-2 (480 unit per mL), (+)-gossypol (500 μg mL−1), (+)-gossypol (500 μg mL−1) + CAT-1 (480 unit per mL), and (+)-gossypol (500 μg mL−1) + CAT-2 (480 unit per mL). (b) The anti-TMV activities of (−)-gossypol (500 μg mL−1), (−)-gossypol (500 μg mL−1) + SOD-1 (360 unit per mL), (−)-gossypol (500 μg mL−1) + SOD-2 (360 unit per mL), (+)-gossypol (500 μg mL−1), (+)-gossypol (500 μg mL−1) + SOD-1 (360 unit per mL), and (+)-gossypol (500 μg mL−1) + SOD-2. (c) The change of O2˙− production rates in tobacco leaves treated by a mixed solvent [mock, (DMF : H2O = 1 : 10)], (−)-gossypol + SOD-1, or (+)-gossypol + SOD-1. (d) The change of O2˙− production rates in tobacco leaves treated by a mixed solvent [mock, (DMF : H2O = 1 : 10)], (−)-gossypol + SOD-2, or (+)-gossypol + SOD-2. Compound + SOD-1 or compound + CAT-1 refer to adding SOD or CAT 10 min before the compound was applied to the tobacco leaves, and compound + SOD-2 or compound + CAT-2 refer to adding SOD or CAT 10 min after inoculation of the tobacco leaves, which were treated by the compounds for 24 h, with TMV. The means of three independent experiments ± SD are shown. | |
In contrast, adding SOD (360 unit per mL) to tobacco leaves before TMV inoculation (SOD-1) can not only suppress the O2˙− accumulation caused by (−)-gossypol (Fig. 5b) or gossypol Schiff bases (ESI, Fig. S16†), but can also reduce their anti-TMV and SOD activities (ESI, Fig. S17†). Also, when SOD was added after TMV inoculation to (−)-gossypol-treated tobacco leaves (SOD-2), the anti-TMV activity of (−)-gossypol was improved (Fig. 5b). This result indicates that the O2˙− accumulation in the tobacco leaves before TMV inoculation is a key factor for the development of tobacco's anti-TMV activity. It should also be noted that the anti-TMV activity of (+)-gossypol was improved irrespective of whether the SOD (360 unit per mL) was added to the tobacco leaves before or after TMV inoculation. This fact demonstrated that the increase of SOD activity after TMV inoculation was favored to suppress necrotic lesion in tobacco leaves, and this fact also further confirmed our view that the O2˙− burst in (+)-gossypol-treated tobacco leaves, after TMV inoculation, was not favorable but rather was harmful to their anti-TMV activity.
From the results above, it was concluded that the gossypol compounds-induced O2˙− accumulation, rather than H2O2 accumulation, in gossypol-treated tobacco leaves is essential to the development of their anti-TMV activity. SOD rather than CAT seems related to the reduction of the necrotic lesion in TMV-inoculated tobacco leaves.
Two sources of O2˙− accumulation in those gossypol compounds-treated tobacco. The (+) and (−)-gossypols have the same O2˙− producing activity in solution (DMF
:
H2O = 1
:
10), so if the O2˙− accumulation is produced by the compound itself, the O2˙− accumulation in the tobacco leaves treated by (+) and (−)-gossypol should be at the same level. However, as we mentioned above, this is not the case. So we naturally come to the conclusion that the O2˙− accumulation in the tobacco leaves treated by (−)-gossypol should not be generated by the (−)-gossypol itself but rather by the tobacco leaves during the interaction between (−)-gossypol and the tobacco leaves, and furthermore that it was endogenous.However, the unexpected results that the gaps between O2˙− accumulation in the tobacco leaves treated by compounds 1 and 2, or compounds 5 and 6, are smaller than that between (+) and (−)-gossypol, led us to believe that the source of O2˙− accumulations in those gossypol Schiff bases-treated tobacco leaves might not be exactly the same as that in (−)-gossypol-treated tobacco leaves.
To confirm this point of view, several additional experiments were designed and performed. NaOH (0.26 mmol L−1) solution (DMF
:
H2O = 1
:
10), which had no anti-TMV activity, could not generate O2˙− or stimulate the O2˙− accumulation in tobacco leaves. As we can see in Fig. 6, compound 3* [compound 3(500 μg mL−1) with 0.3 equiv. (0.26 mmol L−1) NaOH] and compound 4* [compound 4 (500 μg mL−1) with 0.3 equiv. (0.26 mmol L−1) NaOH] displayed higher anti-TMV activities and higher O2˙− production rates in the solution (DMF
:
H2O = 1
:
10) than compound 3 (500 μg mL−1) and compound 4 (500 μg mL−1), respectively.
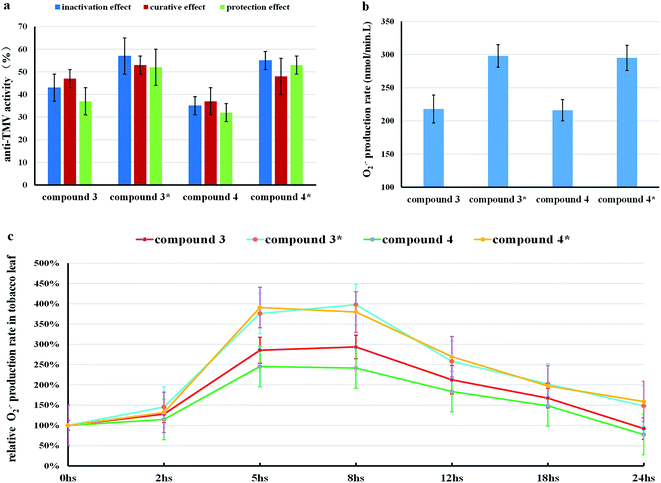 |
| Fig. 6 (a) The anti-TMV activities of compounds 3, 3*, 4, and 4* at a concentration of 500 μg mL−1. (b) The O2˙− production rate of compound 3, 3*, 4, and 4* at a concentration of 500 μg mL−1 and time of 5 h in the solution (DMF : H2O = 1 : 10). (c) The change of O2˙− production rates in tobacco leaves treated by compounds 3, 3*, 4, and 4* at a concentration of 500 μg mL−1. Compound 3* refers to adding 0.3 equiv. of NaOH to the solution (DMF : H2O = 1 : 10) of compound 3 (500 μg mL−1). Compound 4* refers to adding 0.3 equiv. of NaOH to the solution (DMF : H2O = 1 : 10) of compound 4 (500 μg mL−1). The means of three independent experiments ± SD are shown. | |
Assuming the O2˙− producing abilities of (+) or (−)-gossypol Schiff bases in solution (DMF
:
H2O = 1
:
10) were related to the ROS accumulations in tobacco leaves, then compounds 3* and 4* should display higher levels of O2˙− accumulation than that of compounds 3 and 4, separately. This hypothesis was then proven by the fact that both compounds 3* and 4* could induce higher ROS accumulations in tobacco leaves than compounds 3 and 4, respectively (Fig. 6c). So these results confirmed our speculation that the source of O2˙− accumulation in gossypol Schiff base-treated tobacco leaves is different from that in (−)-gossypol-treated tobacco leaves, and this O2˙− accumulation in tobacco is related with the O2˙− producing abilities of (+) or (−)-gossypol Schiff bases, and furthermore that this O2˙− accumulation may be exogenous.
Conclusions
The chirality of gossypols was found to be an influencing factor for the anti-TMV activity of optical gossypols. However, for optical gossypol Schiff bases, their O2˙− producing ability seems more important than their chirality. Detailed studies revealed that the phenomenon was not because they can inhibit the multiplication or assembly of TMV, but rather because they can induce an extreme resistance in the tobacco plant. Further study demonstrated that it is the O2˙− accumulation rather than the H2O2 accumulation in gossypol-treated tobacco leaves that contributes to the development of the anti-TMV activity. Further study of the sources of O2˙− accumulation in tobacco leaves suggested that the O2˙− accumulation in (−)-gossypol-treated tobacco leaves was derived from the interaction between (−)-gossypol and tobacco, and it was related to the chirality of (−)-gossypol. However, the O2˙− accumulation in (+) or (−)-gossypol Schiff bases-treated tobacco leaves was related to their O2˙− production rates. Therefore, by stimulating the O2˙− accumulation in tobacco leaves, both the chirality and the O2˙− production rate of gossypol compounds can influence their anti-TMV activity. Because most research on gossypol's anti-virus activities are usually about the interaction between the virus and specific compounds, this unique anti-virus mechanism reminds us that it is also worthwhile paying attention to the interaction between the compound and its host.
Acknowledgements
We are grateful to the National Natural Science Foundation of China (21372131, 21421062, 21672117) and the Specialized Research Fund for the Doctoral Program of Higher Education (20130031110017) and the Tianjin Natural Science Foundation (16JCZDJC32400) for generous financial support for our programs.
Notes and references
- X. H. Qian, P. W. Lee and S. Cao, J. Agric. Food Chem., 2010, 58, 2613–2623 CrossRef CAS PubMed.
- J. N. Seiber, J. Agric. Food Chem., 2011, 59, 1–21 CrossRef CAS PubMed.
- Y. M. Li, L. H. Wang, S. L. Li, X. Y. Chen, Y. M. Shen, Z. K. Zhang, H. P. He, W. B. Xu, Y. L. Shu, G. D. Liang, R. X. Fang and X. J. Hao, Proc. Natl. Acad. Sci. U. S. A., 2007, 104, 8083–8088 CrossRef CAS PubMed.
-
(a) J. Yang, G. Chen, L. L. Li, W. Pan, F. Zhang, J. Yang, S. Wu and P. Tien, Bioorg. Med. Chem. Lett., 2013, 23, 2619–2623 CrossRef CAS PubMed;
(b) J. Yang, F. Zhang, J. R. Li, G. Chen, S. W. Wu, W. J. Ouyang, W. Pan, R. Yu, J. X. Yang and P. Tien, Bioorg. Med. Chem. Lett., 2012, 22, 1415–1420 CrossRef CAS PubMed;
(c) T. An, W. J. Ouyang, W. Pan, D. Y. Guo, J. R. Li, L. L. Li, G. Yang, J. Chen, S. W. Wu and P. Tien, Antiviral Res., 2012, 94, 276–287 CrossRef CAS PubMed;
(d) R. E. Royer, L. M. Deck, T. J. Vander Jagt, F. J. Martinez, R. G. Mills, S. A. Young and D. L. Vander Jagt, J. Med. Chem., 1995, 38, 2427–2432 CrossRef CAS PubMed;
(e) T. S. Lin, R. Schinazi, B. P. Griffith, E. M. August, B. F. H. Eriksson, D. K. Zheng, L. Huang and W. H. Prusoff, Antimicrob. Agents Chemother., 1989, 33, 2149–2151 CrossRef CAS PubMed;
(f) K. Dodou, Expert Opin. Invest. Drugs, 2005, 14, 1419–1434 CrossRef CAS PubMed.
-
(a) K. Wichmann, A. Vaheri and T. Luukkainen, Am. J. Obstet. Gynecol., 1982, 142, 593–594 CrossRef CAS PubMed;
(b) R. J. Radloff, L. M. Deck, R. E. Royer and D. L. Vander Jagt, Pharmacol. Res. Commun., 1986, 18, 1063–1067 CrossRef CAS PubMed.
- S. A. Vichkanova, A. I. Oifa and L. V. Goryunova, Antibiotiki, 1970, 15, 1071–1073 CAS.
-
(a) L. Li, Z. Li and Q. M. Wang, J. Agric. Food Chem., 2014, 62, 11080–11088 CrossRef CAS PubMed;
(b) L. Li, Z. Li, K. L. Wang, Y. X. Liu and Q. M. Wang, Bioorg. Med. Chem., 2016, 24, 474–483 CrossRef CAS PubMed;
(c) B. Zhang, L. Li, Y. X. Liu and Q. M. Wang, RSC Adv., 2016, 6, 87637–87648 RSC.
-
(a) H. X. Jiang, X. X. Cao, H. Huang and B. Jiang, Tetrahedron: Asymmetry, 2007, 18, 2437–2441 CrossRef CAS;
(b) I. Effenberger, B. Zhang, L. Li, Q. Wang, Y. X. Liu, I. Klaiber, J. Pfannstiel, Q. M. Wang and A. Schaller, Angew. Chem., Int. Ed., 2015, 54, 14660–14663 CrossRef CAS PubMed.
- R. Leberman, Virology, 1966, 30, 341–347 CrossRef CAS PubMed.
- H. Fraenkel-Conrat, Virology, 1957, 4, 1–4 CrossRef CAS PubMed.
- D. Zimmern, Nucleic Acids Res., 1975, 2, 1189–1202 CrossRef CAS PubMed.
-
(a) P. J. G. Butler and A. Klug, Nature, 1971, 229, 47–50 CrossRef CAS;
(b) Y. Okada, Mol. Gen. Genet., 1972, 114, 205–213 CrossRef CAS;
(c) K. E. Richards and R. C. Williams, Proc. Natl. Acad. Sci. U. S. A., 1972, 69, 1121–1124 CrossRef CAS PubMed;
(d) M. Fukuda and Y. Okada, Proc. Natl. Acad. Sci. U. S. A., 1985, 82, 3631–3634 CrossRef CAS PubMed.
-
(a) Z. J. Fan, Z. G. Shi, H. K. Zhang, X. F. Liu, L. L. Bao, L. Ma, X. Zuo, Q. X. Zheng and N. Mi, J. Agric. Food Chem., 2009, 57, 4279–4286 CrossRef CAS PubMed;
(b) H. Jin, D. Y. Hu, L. L. Pang and W. Xue, J. Agric. Food Chem., 2005, 53, 7886–7891 CrossRef PubMed.
- K. L. Wang, B. Su, Z. W. Wang, M. Wu, Z. Li, Y. N. Hu, Z. J. Fan, N. Mi and Q. M. Wang, J. Agric. Food Chem., 2010, 58, 2703–2709 CrossRef CAS PubMed.
- S. Gao, R. Y. Zhang, Z. H. Yu and Z. Xi, ChemBioChem, 2012, 13, 1622–1627 CrossRef CAS PubMed.
- N. Doke and Y. Ohashi, Physiol. Mol. Plant Pathol., 1988, 32, 63–175 Search PubMed.
-
(a) R. Bacsó, Y. Hafez, Z. Király and L. Király, Acta Phytopathol. Entomol. Hung., 2011, 46, 1–10 CrossRef;
(b) Y. M. Hafez, R. Bacsó, Z. Király, A. Künstler and L. Király, Phytopathology, 2012, 102, 848–856 CrossRef CAS PubMed;
(c) A. Künstler, R. Bacsó, Y. M. Hafez and L. Király, Reactive Oxygen Species and Plant Disease Resistance, ed. D. K. Gupta, et al., Springer International Publishing, Switzerland, 2015, DOI:10.1007/978-3-319-20421-5_11.
-
(a) A. Bendahmane, K. Kanyuka and D. C. Baulcombe, Plant Cell, 1999, 11, 781–791 CrossRef CAS PubMed;
(b) M. B. Cooley, S. Pathirana, H. J. Wu, P. Kachroo and D. F. Klessig, Plant Cell, 2000, 12, 663–676 CrossRef CAS PubMed;
(c) M. R. Hajimorad and J. H. Hill, Mol. Plant-Microbe Interact., 2001, 14, 587–598 CrossRef CAS PubMed.
Footnote |
† Electronic supplementary information (ESI) available: 1H NMR, 13C NMR, and HRMS spectra of compounds. See |
|
This journal is © The Royal Society of Chemistry 2017 |