DOI:
10.1039/C6RA28407H
(Paper)
RSC Adv., 2017,
7, 14981-14988
Corrosion behavior of Al film on uranium in salt spray test
Received
19th December 2016
, Accepted 23rd February 2017
First published on 7th March 2017
Abstract
The corrosion behavior of Al film coated uranium and bare uranium under neutral salt spray conditions are evaluated. Moreover, the corrosion process and electrochemical mechanism are investigated. Pits in uranium appear in the initial stage of the salt spray test, which link to each other to form a ring-like morphology along the machining trace. The corrosion product on the surface of uranium is a mixture of U3O8 and UO2. After salt spray for 1.5 h and sealed reservation for 1 month, some corrosion particles spalled off from the Al film. The Al film is eroded first as the sacrificial anode upon exposure to salt spray, which exhibits its excellent protection effect for uranium.
1 Introduction
Uranium is widely applied in the nuclear energy field, medical science, breeding, etc.1,2 Depleted uranium is a nuclear waste in the uranium enrichment process, and hence the 235U content of depleted uranium is about 0.2–0.4% less than that of natural uranium. Therefore, it is less radioactive and usually used as depleted uranium ammunition and depleted uranium-armored tanks. However, depleted uranium is prone to corrosion in the environment due to its high chemical reactivity. The corrosion products of uranium present environmental and health hazards.3–5 Surface coatings have been used to improve its corrosion performance. Protective coatings, such as zinc coatings,6 Al-based coatings,7–9 Ti-based coatings8,10 and Cr-based coatings,11,12 have been prepared to enhance the corrosion resistance of uranium.
Several methods have been used to investigate the corrosion resistance of uranium and its alloys. Research was conducted by observing changes in the atmosphere and the weight gain of samples.13–15 Electrochemical techniques were considered to be common and effective methods to evaluate the corrosion behavior of uranium and alloys.16–26 When exposed to an ocean atmosphere in service, active anions, such as Cl−, invade the matrix to corrode uranium. Salt fog causes high corrosion of materials. The porosity of films affects the corrosion resistance by providing preferential paths for the corrosive species, which causes galvanic corrosion at the interface between the film and substrate.27,28 Kochen et al.29,30 reported the corrosion potential and corrosion rate of uranium alloys in simulated sea water. Bland et al.31 reported the corrosion property of ion plated Cu, Au, Cr and Al films on uranium, which showed that the ion plated Al film had the best corrosion resistance. The galvanic corrosion between depleted uranium and steel was also studied. The Volta potential was evaluated by scanning a Kelvin probe.32,33 There have been few investigations on the corrosion products and corrosion morphology of uranium. Therefore, it is meaningful to study the corrosion behavior of Al film coated uranium and bare uranium in the salt fog environment. After the materials are removed from the salt fog environment, the corrosion pits expand due to the reactivity of the active anions in their surfaces. It is necessary to study the corrosion process of uranium and Al films deposited on uranium in the accelerating mode by salt spray tests to simulate ocean atmosphere corrosion.
In this study, an Al film on the surface of uranium (depleted uranium) was deposited via the magnetron sputtering technology. A neutral salt spray test was carried out to simulate the ocean atmospheric corrosion behavior of uranium and Al film on uranium. The samples were reserved in sealed chamber for 1 month after the salt spray test to evaluate the corrosion process. The morphology and chemical element compositions after the salt spray test were analyzed. The mechanism of salt spray corrosion is discussed to investigate the corrosion behavior of uranium (denoted as U for short) and Al film deposited on uranium (denoted as Al/U for short) under the ocean atmosphere with electrochemical performance tests.
2 Experimental
2.1 Al film preparation
The sputtering of the Al film was carried out on a magnetron sputtering instrument. The target was sintered Al of 99.99% purity. Φ 25 × 2 mm uranium samples were placed into a vacuum chamber after ultrasonic cleaning in acetone and alcohol. The distance between the substrate and the target was kept at 100 mm. After the base pressure was pumped just below 5 × 10−4 Pa, the working pressure was kept at 0.3 Pa with argon (99.999% purity) flowing into the chamber with a flow of 40 sccm to carry out the deposition process. The substrate temperature was kept at 473 K during the deposition. The target power was 3 kW during the sputtering process. The bias voltage was −100 V. The thickness of the Al film obtained was approximately 10 μm.
2.2 Film characterization
The structure of the film was measured using an Empyrean X-ray diffractometer employing Cu Kα radiation (λ = 0.15406 nm). The morphology of the sample before and after the salt spray test was observed by scanning electron microscopy (KYKY-EM3200) and optical microscopy (OLS-4000). Energy dispersion X-ray spectroscopy (EDX) was used to analyze the composition of the corrosion product.
2.3 Salt spray corrosion test
Neutral salt spray corrosion tests34 were performed according to ASTM B117-97. Tests on five samples were performed in a salt spray chamber and the experimental conditions were as follows: continuous salt spray of 5 ± 0.5 wt% NaCl (purity of 99.5%) solution; temperature of 35 °C ± 1 °C; pH of 6.5–7.2; and deposition rate of 1.5 mL/80 cm2 h, all within the specifications given in ASTM B117-97. The backsides and rims of the samples were sealed with epoxy resin. Samples were observed by optical microscopy every 0.5 h. After the salt spray test, samples were reserved in a sealed chamber. The reservation conditions were as follows: temperature of 25 °C and humidity of 20% RH. The morphologies and chemical element compositions were analyzed after the salt spray test.
2.4 Electrochemical corrosion tests
Electrochemical tests were carried out using a PARSTAT 2263 electrochemical workstation equipped with three electrodes. The sample was set as the working electrode. A graphite electrode and saturated calomel electrode (SCE) were used as the counter and reference electrodes, respectively. The effective area of the samples was 1 cm2 embedded in epoxy resin. The experiments were performed in 50 μg g−1 Cl− solution in distilled water. The chemical used in the experiment was KCl (AR, purity of 99.5%). The samples were dipped in the solution to reach the steady open circuit potential (OCP). The potential sweep rate of 2 mV s−1 was adopted to perform the potentiodynamic polarization tests.
2.5 Corrosion product analysis
Raman spectra of depleted uranium after salt spray corrosion were recorded with an XploRA Raman spectrometer (Horiba Jobin Yvon Company). The spectra were accumulated with a resolution of ∼4 cm−1 and an excitation source of 532 nm radiation from an Nd: YVO4 laser (frequency-doubled).
The AES depth profile was used to analyze the distribution of C, O, Al and Cl elements for the Al film after the salt spray test. The AES instrument model was PHI650 SAM, the excitation energy was 3 keV, electron beam current was 100 nA, and sputtering area was 1 mm2.
3 Results and discussion
3.1 Al film characterization
The surface and cross-section morphologies of the Al film prepared via magnetron sputtering are shown in Fig. 1. From the SEM images, it can be seen that the micro-structure of the Al film is densely packed, with no voids and no micro-cracks (Fig. 1a). A few macro-particles are found on the surface. The maximum size of the particles is about 0.5 μm. Fig. 1b shows the cross-section image of the Al film. There was no columnar crystal structure in the film. The thickness of the Al film was about 10 μm.
 |
| Fig. 1 Surface image (a) and cross-section image (b) of the Al film. | |
To evaluate the phase structure of the Al film, XRD analysis was performed. Fig. 2 shows the XRD patterns of the Al film. The coexistence of (111), (200), (220), (311) and (222) peaks reveal that Al has no distinct preferential orientation. It can be observed that the peaks are relative to the data of the standard PDF 04-0787.
 |
| Fig. 2 XRD spectrum of the Al film. | |
3.2 Results of salt spray corrosion
Fig. 3 illustrates the macro-optical morphologies of U and Al/U after 1.5 h salt spray corrosion. Two typical features are observed for uranium: small pits (Fig. 3a) and large corrosion zones (Fig. 3b). A few corrosion pits appear in the uranium surface after 0.5 h exposure in the salt spray. As the corrosion time increased, the number of pits increased, the diameter of pits expanded gradually, and the pits began to connect with each other. The small pits are almost exhibited in the machining trace. The large corrosion zones are due to the connecting of small corrosion pits. The largest depth of the corrosion area is about 18.04 μm. There is no significant change in the Al film after the 1.5 h salt spray test, as shown in Fig. 3c and d.
 |
| Fig. 3 Macro-optical morphologies of the U (a and b) and Al/U (c and d) samples after 1.5 h salt spray corrosion. | |
Fig. 4 shows the micro-structure and EDX result of the Al film and uranium after 1.5 h salt spray corrosion. Fig. 4a is the SEM image of uranium after the salt spray corrosion test. From the EDX patterns of spots 1–3, it can be observed that the elements (U, C and O) in the corrosion area (spot 1 and spot 2) are identical with the matrix (spot 3). In contrast, Fe or Al elements have strong lines in the corrosion area (spot 1 and spot 2). The impurity elements could lead to surface heterogeneity and induce corrosion features.3 These impurity elements influence the corrosion morphology and increase the corrosion rate, which is irrelevant to the corrosion nucleation site. Cracks were found on the surface of uranium, which caused spalling phenomena, as shown in Fig. 4b. The Al film was only slightly corroded with a dense layer of corrosion products on the top of the film after 1.5 h salt spray corrosion, as shown in Fig. 4c. The EDX result of the Al film after salt spray corrosion is shown in Fig. 4c (spot 4), in which Al and O atoms are the main compositions of the film, which indicates that the base uranium was well protected.
 |
| Fig. 4 Micro-structure and EDX spectra of U (a and b) and Al/U (c) after 1.5 h salt spray corrosion. | |
Fig. 5 illustrates the macro-optical morphologies of the U and Al/U samples after 1.5 h salt spray corrosion and 1 month sealed reservation. Uranium was heavily corroded with its surface overlaid by an enormous amount of corrosion products, as shown in Fig. 5a and b. From Fig. 5c and d, a few corrosion pits (darker zones) are observed in a randomized arrangement on the surface of the Al film. The diameter of the corrosion pits is below 50 μm. The invalidations are mainly expressed in the form of corrosion pits and cracks in uranium (Fig. 5a and b). The corrosion areas connected to each other to form a ring-like morphology along the machining trace. A large amount of debris spalled from the surface, and the largest depth of the corrosion area was about 39.86 μm.
 |
| Fig. 5 Macro-optical morphologies of the U (a and b) and Al/U (c and d) samples after 1.5 h salt spray corrosion and 1 month sealed reservation. | |
Fig. 6 shows the micro-structure and EDX spectra of the uranium and Al film after 1.5 h salt spray corrosion and 1 month sealed reservation. The corrosion areas connected to each other to form a ring-like morphology along the machining trace, as shown in Fig. 6a. Cracks were found on the surface of uranium, as shown in Fig. 6b. From the EDX patterns of spot 1 and spot 2, the elements (U, C and O) in the corrosion area are identical. In contrast, the spot 1 corrosion area has a greater content of Al element than the spot 2 area, which means that the area without impurity Al element was also heavily corroded after 1 month sealed reservation. It can be seen from Fig. 6d that the Al film chapped after 1.5 h salt spray corrosion and 1 month sealed reservation. The EDX spectra only show a small amount of C, O, Cl and Ar elements without the U element that was obtained in the matrix, which indicate that the Al film was only slightly corroded and uranium was well protected.
 |
| Fig. 6 Micro-structure and EDX spectra of U (a and b) and Al/U (c and d) after 1.5 h salt spray corrosion and 1 month sealed reservation. | |
3.3 Potentiodynamic polarization test
In order to determine the effectiveness of the Al film in corrosion protection, potentiodynamic polarization measurements were conducted in a 50 μg g−1 Cl− solution. Fig. 7 shows the potentiodynamic polarization curves of U and the Al film on uranium. It can be seen that the samples have the same electrochemical behavior in the test potential range. On the zones above the self-corrosion potentials, the anodic current densities increased rapidly with the enhancement of the polarization potentials. The anodic reaction curve corresponds to the dissolution reactions of uranium and Al. The anodic reaction was inhibited in the presence of the Al film, indicating that the Al film effectively enhances the corrosion resistance.
 |
| Fig. 7 Polarization curves of U and Al/U in 50 μg g−1 Cl− solution. | |
The self-corrosive potential and self-corrosive current is presented in Table 1. Resistance to electrochemical corrosion can be estimated by calculating the sample's self-corrosive potential (Ecorr) and self-corrosive current (Icorr). A high Ecorr indicates good resistance to corrosion, and a low Icorr means a low corrosion rate.35 The self-corrosive potential of uranium is about −641 mV vs. SCE. The self-corrosive potential of Al on uranium declined by about 67 mV vs. SCE, whereas the corrosion current density dropped by an order of magnitude, indicating lower corrosion rate for the Al film on uranium. The Al film possessing a negative electrode potential works as a sacrificial anode, forming a galvanic couple with uranium and is preferentially corroded rather than uranium.
Table 1 Electrochemical corrosion measurement results in 50 μg g−1 Cl− solution
Samples |
Ecorr (mV vs. SCE) |
Icorr (μA cm−2) |
U |
−641 ± 23 |
2.697 ± 0.04 |
Al/U |
−708 ± 35 |
0.29 ± 0.01 |
3.4 Mechanism of salt spray corrosion
Fig. 8 shows the Raman spectra of the corrosion product of uranium after the salt spray corrosion test. There are four main Raman peaks at the positions of about 112.6 cm−1, 236.1 cm−1, 445.7 cm−1 and 752.4 cm−1 (Fig. 8b) at spot 1 (Fig. 8a). There are three main Raman peaks at the positions of about 444.5 cm−1, 578.9 cm−1 and 1151.8 cm−1 (Fig. 8c) at spot 2 (Fig. 8a). The bands at 112.6 cm−1, 236.1 cm−1 and 752.4 cm−1 are assigned to U3O8, and the bands at 444.5 cm−1, 578.9 cm−1 and 1151.8 cm−1 are assigned to UO2.36–38 The corrosion product of the darker zones (Fig. 8a) is a mixture of U3O8 and UO2. The corrosion product of the lighter zones (Fig. 8a) is UO2.
 |
| Fig. 8 Optical morphology (a) and Raman spectra of the corrosion product for the severe corrosion region (b) and slight corrosion region (c). | |
An extra thin salt-containing moisture film, with more oxygen adsorbing and dissolving, formed due to deposition during the salt spray test. The salt spray corrosion is electrochemical corrosion. The Cl− in the media accelerated the destruction of the oxidized film and corrosion holes occurred. After the formation of corrosion holes, the dissolution of uranium in the holes accelerated under the effect of a large cathode and small anode. Then, the pits connected to each other, the cracks connected to each other, and finally the layer surface spalling phenomenon appeared.
The typical Auger depth profiles of the Al, C, Cl, and O elements in the Al film on uranium after salt spray corrosion are illustrated in Fig. 9. On the initial surface, the content of the C and O elements are very high. With an increase in the Ar+ etching time, the content of C decreases quickly, which shows that some of the C element came from the surface contaminants. There is a small content of Cl at the surface. The content of O element is very low, and the content of Al element is over 98%, which indicates that the Al film is only slightly corroded on the surface and uranium is well protected.
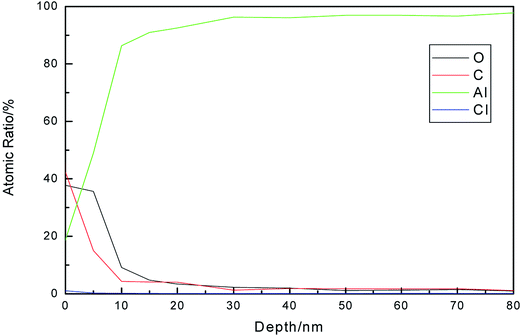 |
| Fig. 9 AES depth profiles of the Al, C, Cl and O elements in the Al film on uranium after salt spray corrosion. | |
Al reacts with active oxygen and water, which are formed due to deposition during the salt spray test, to form Al(OH)3 (see eqn (1)). Then, Cl− replaces OH−.39 As can be observed from Fig. 6d and 9, Cl is found in the EDX and AES results, which indicate that the product is AlCl3 (see eqn (2)).
|
4Al + 3O2 + 6H2O → 4Al(OH)3
| (1) |
|
Al(OH)3 + 3Cl− + 3H+ → AlCl3 + 3H2O
| (2) |
The surface morphologies of the Al film after salt spray corrosion are illustrated in Fig. 3–6. Only a few corrosion pits are observed on the surface. After 1 month reservation, spalling was found in the Al film. The main reason for this is that the Al film had a uniform distribution of elements without a weak zone to cause a large cathode and small anode. The corrosion pits in the Al film were small and in a randomized arrangement. The Al film was eroded first upon exposure to salt spray as a sacrificial anode to protect uranium.
4 Conclusion
An Al film of about 10 μm thickness was deposited on depleted uranium using the magnetron sputtering technology to improve its corrosion resistance. There was no columnar crystal structure in the dense Al film. Corrosion pits were found in uranium after exposure to salt spray for 0.5 h on the area with Al or Fe impurity elements. The corrosion areas in the surface of uranium connected to each other to form a ring-like morphology along the machining trace after salt spray exposure for 1.5 h and sealed reservation for 1 month. The corrosion product was a mixture of U3O8 and UO2. The Al film was only slightly corroded with a dense layer of corrosion products on the top of the film after 1.5 h salt spray corrosion. After 1 month reservation, the Al film chapped on the surface. Al film was eroded preferentially upon exposure to salt spray as a sacrificial anode to protect uranium.
Acknowledgements
We would like to thank Dingmu Lang, Yan Liu and Qingfu Wang for their assistance in experimental measurements. This study is supported by the National Natural Science Foundation of China No. 51401188.
References
- C. Alippi, A unique timely moment for embedding intelligence in applications, CAAI Transactions on Intelligence Technology, 2016, 1, 1–3 CrossRef.
- C. Tang, R. Wang, Z. Li, W. Wang and W. Gao, Frame interpolation with pixel-level motion vector field and mesh based hole filling, CAAI Transactions on Intelligence Technology, 2016, 1, 72–78 CrossRef.
- D. F. Roeper, D. Chidambaram, C. R. Clayton and G. P. Halada, Development of an Environmental Friendly Protective Coating for the Depleted Uranium-0.75 wt% Titanium Alloy: Part V. Electrochemical Impedance Spectroscopy of the Coating, Electrochim. Acta, 2008, 53, 2130–2134 CrossRef CAS.
- D. F. Roeper, D. Chidambaram, C. R. Clayton and G. P. Halada, Development of an Environmental Friendly Protective Coating for the Depleted Uranium-0.75 wt% Titanium Alloy: Part I. Surface Morphology and Electrochemistry, Electrochim. Acta, 2005, 50, 3622–3633 CrossRef CAS.
- J. M. Haschke, Corrosion of Uranium in Air and Water Vapor: Consequences for Environmental Dispersal, J. Alloys Compd., 1998, 278, 149–160 CrossRef CAS.
- L. J. Weirick, Hot-dipped Tin–Zinc on U-0. 75 wt% Ti, SAND-79–0478, pp. 1–46 Search PubMed.
- F. Chang, M. Levy, B. Jackman and W. B. Nowak, Assessment of Corrosion-resistant Coatings for a Depleted Uranium-0.75 Titanium Alloy, Surf. Coat. Technol., 1991, 48, 31–39 CrossRef CAS.
- F. Chang, M. Levy, R. Huie, M. Kane, P. Buckley, T. Z. Kattamis and G. R. Lakshminarayan, Adhesion and Corrosion Behavior of Al–Zn and TiN/Ti/TiN Coatings on a DU-0.75 wt% Ti Alloy, Surf. Coat. Technol., 1991, 49, 87–96 CrossRef CAS.
- A. K. Singh, S. Kaity, K. Singh, J. Thomas, T. R. G. Kutty and S. Sinha, Pulsed Laser Deposition of Alumina Coating for Corrosion Protection Against Liquid Uranium, Mater. Chem. Phys., 2014, 143, 1446–1451 CrossRef CAS.
- T. W. Liu, C. Dong, S. Wu, K. Tang, J. Y. Wang and J. P. Jia, TiN, TiN Gradient and Ti/TiN Multilayer Protective Coatings on Uranium, Surf. Coat. Technol., 2007, 201, 6737–6741 CrossRef CAS.
- S. Zhu, L. Chen, Y. Wu, Y. Hu, T. Liu, K. Tang and Q. Wei, Microstructure and Corrosion Resistance of Cr/Cr2N Multilayer Film Deposited on the Surface of Depleted Uranium, Corros. Sci., 2014, 82, 420–425 CrossRef CAS.
- S. Zhu, Y. Wu, T. Liu, K. Tang and Q. Wei, Interface Structure and Corrosion Resistance of Ti/Cr Nanomultilayer Film Prepared by Magnetron Sputtering on Depleted Uranium, ACS Appl. Mater. Interfaces, 2013, 5, 6598–6602 CAS.
- J. Charles and L. J. Weirick, The Oxidation of Uranium-0.75 wt% Titanium in Environments Containing Oxygen and/or Water Vapor at 140 °C, J. Nucl. Mater., 1987, 144, 110–120 CrossRef.
- J. M. Haschke, T. H. Allen and L. A. Morales, Reactions of Plutonium Dioxide with Water and Hydrogen–Oxygen Mixtures: Mechanisms for Corrosion of Uranium and Plutonium, J. Alloys Compd., 2001, 314, 78–91 CrossRef CAS.
- G. W. McGillivray, D. A. Geeson and R. C. Greenwood, Studies of the Kinetics and Mechanism of the Oxidation of Uranium by Dry and Moist Air: a Model for Determining the Oxidation Rate over a Wide Range of Temperatures and Water Vapor Pressures, J. Nucl. Mater., 1994, 208, 81–97 CrossRef CAS.
- K. H. Eckelmeyer, A. D. Romig and L. J. Weirick, The Effect of Quench Rate on the Microstructure, Mechanical Properties, and Corrosion Behavior of U-6 Wt Pct Nb, Metall. Mater. Trans. A, 1984, 15, 1319 CrossRef.
- H. M. Volz, R. E. Hackenberg and A. M. Kelly, X-Ray diffraction analyses of aged U-Nb alloys, J. Alloys Compd., 2007, 444–445, 217–225 CrossRef CAS.
- Y. Zhang, X. Zhang, X. Cheng, W. Guan and X. Wang, Effect of grain size on phase stability of monoclinic U-Nb alloy during low-temperature aging, J. Nucl. Mater., 2015, 456, 167–169 CrossRef.
- V. F. Peretrukhin, A. G. Maslennikov, A. Y. Tsivadze, C. H. Delegard, A. B. Yusov, V. P. Shilov, A. A. Bessonov, K. E. German, A. M. Fedoseev, L. P. Kazanskii, N. Y. Budanova, A. V. Kareta, A. V. Gogolev, K. N. Gedgovd and G. S. Bulatov, Corrosion of uranium and its low content Zr, Nb, and Ru alloys in aqueous solution, Prot. Met., 2008, 44, 211–232 CrossRef CAS.
- B. A. Hilton, Review of oxidation rates of DOE spent nuclear fuel, Part 1: Metallic fuel, Report ANL-00/24, Argonne national laboratory, Idaho Falls, ID, USA, 2000 Search PubMed.
- J. S. Bullock, Electrochemical studies of uranium alloys corrosion, in Physical Metallurgy of Uranium Alloys, ed. J. J. Burke, Brook Hill, Chestnut Hill, MA, 1976, pp. 847–895 Search PubMed.
- M. Levy and C. V. Zabielski, Physical Metallurgy of Uranium Alloys, ed. J. J. Burke, Hill publishing company, Chestnut Hill, USA, 1976, p. 897 Search PubMed.
- X. Fu, X. Wang, C. Bai and Z. Zhao, Electrochemical corrosion behavior of uranium–niobium alloy in aqueous solutions, Ordnance Mater. Sci. Eng., 2001, 24, 13–16 Search PubMed.
- D. Kelly, J. A. Lillard, W. L. Manner, R. Hanrahan Jr and M. T. Paffett, Surface characterization of oxidative corrosion of U-Nb alloys, J. Vac. Sci. Technol., A, 2001, 19, 1959 CAS.
- S. Orman, Metallurgy of uranium alloys, ed. J. J. Burke and D. A. Colling, J Greenspan Brook Hill, New York, 1974 Search PubMed.
- L. J. Weirick. Corrosion of uranium and uranium alloys, ASM Metals Handbook, American Society of Metals, Metals Park, OH, 1988 Search PubMed.
- F. C. Walsh, C. Ponce, C. Kerr, S. Court and B. D. Barker, Electrochemical characterisation of the porosity and corrosion resistance of electrochemically deposited metal coatings, Surf. Coat. Technol., 2008, 202, 5092–5102 CrossRef CAS.
- A. Lekatou, D. Zois, A. E. Karantzalis and D. Grimanelis, Electrochemical behavior of cermet coatings with a bond coat on Al7075: Pseudopassivity, localized corrosion and galvanic effect considerations in a saline environment, Corros. Sci., 2010, 52, 2616–2635 CrossRef CAS.
- J. M. Kochen, The galvanic corrosion behavior of uranium alloys in hydrochloric acid and ocean water, RFP-1592, Rocky Flats Plant, Feb 1971 Search PubMed.
- J. M. Kochen, The corrosion behavior of uranium-Base U-Nb, U-Nb-Zr, and U-Mo alloys in hydrochloric acid and ocean water, RFP-1586, Rocky Flats Plant, Feb 1971 Search PubMed.
- R. D. Bland, Ion-plated coating for the corrosion protection of uranium, Atomic energy commission contract report, 1965, SC-DR-65–519 Search PubMed.
- Z. Pu, Q. Wang and M. Shuai, Galvanic corrosion between depleted uranium and 40Cr steel, Int. J. Electrochem. Sci., 2013, 8, 991–1001 CAS.
- Z. Pu, Q. Wang and M. Shuai, Galvanic corrosion between depleted uranium and 45 steel, Mater. Sci. Forum, 2013, 749, 606–610 CrossRef.
- J. Zhu, S. Wei, I. Y. Lee, S. Park, J. Willis, N. Haldolaarachchige, D. P. Young, Z. Luo and Z. Guo, Silica stabilized iron particles toward anti-corrosion magnetic polyurethane nanocomposites, RSC Adv., 2012, 2, 1136–1143 RSC.
- Z. B. Bao, Q. M. Wang, W. Z. Li, J. Gong, T. Y. Xiong and C. Sun, Corrosion behavior of AIP NiCoCrAlYSiB coating in salt spray tests, Corros. Sci., 2008, 50, 847–855 CrossRef CAS.
- M. L. Palacios and S. H. Taylor, Characterization of uranium oxides using in situ micro-Raman spectroscopy, Appl. Spectrosc., 2000, 54(9), 1372–1378 CrossRef CAS.
- D. Manara and B. Renker, Raman spectra of stoichiometric and Hyperstoichiometric uranium dioxide, J. Nucl. Mater., 2003, 321(2–3), 233–237 CrossRef CAS.
- E. A. Stefaniak, A. Anita and W. Anna, Recognition of uranium oxides in soil particulate matter by means of μ-Raman spectrometry, J. Nucl. Mater., 2008, 381(15), 278–283 CrossRef CAS.
- C. F. Dong, Y. H. An, X. G. Li, H. Sheng and K. Xiao, Electrochemical performance of initial corrosion of 7A04 aluminium alloy in marine atmosphere, Chin. J. of Nonferrous Met., 2009, 19, 346–352 CAS.
|
This journal is © The Royal Society of Chemistry 2017 |
Click here to see how this site uses Cookies. View our privacy policy here.