DOI:
10.1039/C6RA27697K
(Paper)
RSC Adv., 2017,
7, 801-804
Synthesis of a cyclopentadienyl(imino)stannylene and its direct conversion into halo(imino)stannylenes†
Received
2nd December 2016
, Accepted 8th December 2016
First published on 3rd January 2017
Abstract
The reaction of stannocene Cp2Sn with iminolithium LiNIPr (NIPr = bis(2,6-diisopropylphenyl)imidazolin-2-iminato) afforded the dimeric cyclopentadienyl(imino)stannylene [(η1-Cp)SnNIPr]2 (1). Compound 1 exhibits unexpected reactivity towards haloalkanes. The high-yield conversion of 1 into chlorostannylene [ClSnNIPr]2 (2) and bromostannylene [BrSnNIPr]2 (3) were accomplished by treatment with dichloromethane or 1,2-dibromoethane, respectively, through a Cp-substitution reaction.
Low-valent and low-coordinate group 14 compounds have been of great interest in main group chemistry and much attention has been paid to this compound class over the last several decades. Since the first monomeric diaminostannylene [Sn(N(SiMe3)2)2] I was published in 1974 (Fig. 1),1 numerous compounds with a divalent tin center have been reported.2 At the same time, the chemistry of stannocenes that are tin(II) analogues of metallocenes, has been intensively studied by the group of Jutzi, and others.3 It is noteworthy that Cp2Sn converted into cyclopentadienylchlorostannylene [CpSnCl] by disproportionation of stannocene with SnCl2.4 Chlorostannylene can be used as a starting material for a novel low-valent organotin compounds. Although transition metal complexes with cyclopentadienyl ligand(s) are the most studied organometallic compound class, the field of low-valent half sandwich Sn(II) species has not been fully developed so far.5 For example, Wright and co-workers reported the synthesis and isolation of the Cp-substituted stannylene dimmer ([(η3-Cp)SnNC(NMe)2]2) II (Cp = C5H5) by the direct substitution of Cp2Sn with LiNC(NMe3)2 (Fig. 1).5a Furthermore, Power and co-workers reported the Cp-substituted arylstannylene III by C–H activation of cycloalkene with distannyne (Fig. 1).5h Due to the π-electron donating nature of Cp ligand, Cp-substituted stannylenes possess an electron rich tin(II) center that may show unique reactivity towards organic molecules. Yet, remarkably little is known about the reactivity of Cp-substituted tin derivatives. The Cp ligand in II could be replaced with lithiated 1,3-dithianes as nucleophiles.6 Development of a novel method for the facile access to halogenated stannylenes from a Cp-substituted stannylene would be of great importance because they could be suitable precursors for novel functionalized tin(II) compounds through nucleophilic substitutions.
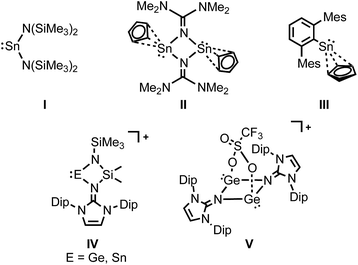 |
| Fig. 1 Selected tin(II) compounds I–III (top) and metallyliumylidenes IV and V (bottom). | |
It has been shown that imidazolin-2-imino ligands, namely N-heterocyclic imines (NHIs), can be employed as ligands for a variety of transition-metal complexes.7 Also, by the use of this ligand system, a number of fascinating main group element complexes8 (boron,9 aluminium,10 silicon,11 germanium,12 tin,13 and phosphorus14) have been reported. For instance, our group recently developed a new straightforward method for synthesizing Ge(II)12b and Sn(II)13a cations IV (Fig. 1). Furthermore, this ligand can also be implemented for the isolation of new triflate-coordinate bis(germyliumylidene) V (Fig. 1),12c ascribed to a combination of a strong electron-donating effect and a delocalization of a positive charge. Herein we describe the synthesis and structure of the Cp-substituted iminostannylene 1 as well as its unusual reactivity toward haloalkanes.
The reaction of Cp2Sn with one equivalent of LiNIPr (NIPr = bis(2,6-diisopropylphenyl)imidazolin-2-iminato) in THF affords the dimeric cyclopentadienyl(imino)stannylene [(η1-Cp)SnNIPr]2 (1) (Scheme 1). The formation of 1 was confirmed by multinuclear NMR spectroscopy, high resolution mass spectrometry and single-crystal X-ray diffraction data (Fig. 2).
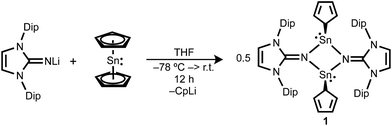 |
| Scheme 1 Synthesis of 1 [CpSnNIPr]2 (Dip = 2,6-diisopropylphenyl). | |
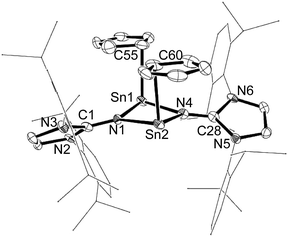 |
| Fig. 2 ORTEP representation of the molecular structure of 1 in the solid state. Thermal ellipsoids are at the 40% probability level. Hydrogen atoms are omitted for clarity. Dip groups are depicted as wireframes. Selected bond lengths (Å) and bond angles (deg): Sn1–N4 2.220(7), Sn1–N1 2.227(7), Sn2–N1 2.193(7), Sn2–N4 2.222(7), Sn1–C55 2.442(10), Sn2–C60 2.438(10), N1–C1 1.287(12), N2–C1 1.414(11), N3–C1 1.398(12), N4–C28 1.297(12), N5–C28 1.392(12), N6–C28 1.412(12); N4–Sn1–N1 76.9(3), N1–Sn2–N4 77.6(3), Sn2–N1–Sn1 102.2(3), Sn1–N4–Sn2 101.5(8), N4–Sn1–C55 96.3(3), N1–Sn1–C55 99.0(4), N1–Sn2–C60 93.7(3), N4–Sn2–C60 97.9(4). | |
The molecular structure of 1 consists of a nearly centrosymmetric dimer arrangement with a nearly planar Sn2N2 core (the sum of internal tetragonal angles = 358.2°) protected by two bulky IPr groups. The imino groups bridge the two Sn centers almost symmetrically in the Sn2N2 ring (Sn(1)–N(1) 2.227(7) Å, Sn(1)–N(4) 2.220(7) Å, Sn(2)–N(1) 2.193(7) Å, Sn(2)–N(1) 2.222(7) Å), which defines the presence of strong N → Sn interaction. These values are slightly elongated in comparison to the corresponding values of azido- (av. 2.186(3) Å), and chlorostannylene (av. 2.198(5) Å) analogues,13b probably owing to the more sterically crowded environment on the Sn atoms in 1. Unlike other imino-substituted tin(II) dimers ([XSnNIPr]2, X = N3, Cl),13 the terminal η1-Cp ligands have a cis orientation with respect to the Sn2N2 ring for 1. The C–N distances in the imidazoline fragment (1.287(12), 1.297(12) Å) are typical for a carbon–nitrogen double bond.15
The asymmetric coordination mode of Cp ligands found in the solid structure of 1 is not reflected by the solution-state 1H NMR measurements, where a single resonance for the Cp ring is observed at 5.66 ppm. This data clearly shows that 1 exhibits fluxional behaviour of a Cp ligand in a solution. In sharp contrast to II, compound 1 does not exist in equilibrium between the cis and trans Cp isomers in the solution. The 119Sn NMR spectrum displays a singlet at −232 ppm. This resonance is low field shifted compared to that of stannocene (δ = −2199 ppm),16 but is high field shifted than that of the stannylene III (δ = 94 ppm)5h probably due to the dimeric form of 1.
To take a closer look at the overall electronic nature of 1, DFT calculations for 1 were carried out using the B3LYP theory level with the def2-SVP basis set. The optimized structure closely reproduced the experimentally observed structure of 1. The frontier molecular orbitals of 1 show that the HOMO corresponds to the Sn lone-pair electrons, while the LUMO is the π*-orbital of the Dip groups in the imidazoline ligands (Fig. S13†).
The reactivity of bis(amino)stannylene [Sn(N(SiMe3)2)2] I towards halogenated substrates has been thoroughly investigated by Lappert and co-workers.17 The oxidative addition of I with haloalkanes was found to proceed via an electron transfer reaction between the stannylene and the substrate, followed by abstraction of the halide to leave the tin and alkyl radicals, which act as the propagating species in a radical chain reaction.17 In sharp contrast, the study of half-sandwich stannylenes has been limited so far. This motivated us to explore the reactivity of 1 bearing both Cp as well as imino ligand.
The stannylene 1 readily reacts with CH2Cl2 or BrCH2CH2Br, producing the halogenated compounds [ClSnNIPr]2 2 and [BrSnNIPr]2 3, respectively (Scheme 2). This reaction is thought to be the substitution of Cp ligand by halide of the substrates. While the arene elimination at the tin(II) center of stannylene instigated by hydrogen or ammonia was investigated both experimentally and theoretically,18 the observed reaction is a rare example of a direct transformation of metallylenes to halometallylenes using haloalkanes.19 This is in sharp contrast to that of [Sn(N(SiMe3)2)2] I, which undergoes oxidative addition.17 The identity of the chlorostannylene dimer 2 was confirmed by comparison of the NMR spectra to literature data.13b The bromostannylene 3 has been characterized by high-resolution mass spectrum, NMR spectroscopy and X-ray structure analysis. The 119Sn NMR spectrum of 3 exhibits a singlet resonance at −88 ppm, which is low-field shifted by the chloride analogue 2 (−125 ppm) owing to the lower electronegativity of Br than Cl. The X-ray single-crystal structure of 3 revealed a four-membered Sn2N2 ring with two additional terminal bromine atoms. The bromine and tin atoms in 3 are disordered and only one component is shown in Fig. 3. The internal ring angle at the tin is 77.53(12)°, and that at nitrogen average 102.47(11)°. The average Sn–N bond length (2.173(3) Å) is shorter than those in 1 (2.216(7) Å) and 2 (2.198(5) Å). Akin to 2, the halide moieties of 3 adopt a trans configuration with respect to the Sn2N2 ring after substitution of the Cp ligands. The Sn–Br bond in 3 is oriented nearly perpendicular to both Sn–N bonds, with Br1–Sn1–N1 and Br1–Sn1–N2 bond angles of 89.65(8)° and 86.93(7)°, respectively, which are comparable to those in 2 (average 87.72(15)°).
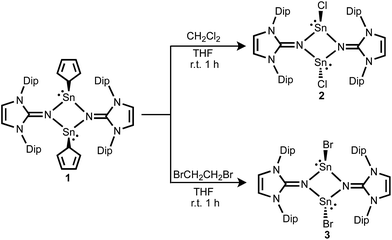 |
| Scheme 2 Synthesis of 2 and 3. | |
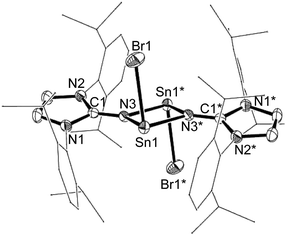 |
| Fig. 3 ORTEP representation of the molecular structure of the cation of 3 in the solid state. Thermal ellipsoids are at the 40% probability level. Dip groups are depicted as wireframes. Hydrogen atoms are omitted for clarity. Selected bond lengths (Å) and bond angles (deg): Sn1–N3 2.185(3), Sn1–N3* 2.161(3), Sn1–Br1 2.6933(6), C1–N1 1.378(5), C1–N2 1.410(5), C1–N3 1.302(5); N3–Sn1–N3* 77.53(12), Sn1–N3–Sn1* 102.47(11), N3–Sn1–Br1 86.93(7), N3*–Sn1–Br1 89.65(8). | |
The relative energy calculations for the cis/trans isomers of [(η1-Cp)SnNIPr]2 1 and [ClSnNIPr]2 2 were carried out. The cis isomer of 1 is thermodynamically more stable than the trans isomer by 1.2 kcal mol−1 calculated at the B3LYP/def2-SVP level of theory. In contrast, the calculation shows that trans-[ClSnNIPr]2 is more stable compared to cis-[ClSnNIPr]2 in 2 by 6.2 kcal mol−1. Although energy differences for these isomers were not large, this theoretical study is consistent with the experimental observations, demonstrating that the cis/trans conformation of the dimeric iminostannylenes deeply depends on the steric factors of the substituents.
Conclusions
In summary, we report the synthesis and characterization of a Cp-substituted iminostannylene 1. In addition, we have shown its reactivity toward haloalkanes, resulting in the C–E (E = Cl, Br) bond cleavage reaction as well as substitution reaction of the Cp group by the halides. This methathesis reaction afforded the dimeric organotin(II) halides 2 and 3 in high yield. We are currently investigating other small molecules activation such as O2, CO2 and N2O by using dimeric tin(II) compounds 1–3 and preparing novel four-membered stannylenes by nuclephiric addition or halide abstraction reaction of halogen-substituted stannylens 2 and 3.
Acknowledgements
We are exceptionally grateful to the Alexander von Humboldt Foundation (Sofja Kovalevskaja Program) and the WACKER Chemie AG for financial support. This work was supported by the German Research Foundation (DFG) and the Technische Universität München within the Open Access Publishing Funding Programme. We thank Dr Elisabeth Irran for reviewing the X-ray diffraction data. Parts of this paper have been published in the PhD Thesis of Tatsumi Ochiai.20
Notes and references
-
(a) D. H. Harris and M. F. Lappert, J. Chem. Soc., Chem. Commun., 1974, 895 RSC;
(b) P. J. Davidson, D. H. Harris and M. F. Lappert, J. Chem. Soc., Dalton Trans., 1976, 2268 RSC;
(c) D. E. Goldberg, D. H. Harris, M. F. Lappert and K. M. Thomas, J. Chem. Soc., Chem. Commun., 1976, 261 RSC;
(d) T. Fjeldberg, A. Haaland, B. E. R. Schilling, M. F. Lappert and A. J. Thorne, J. Chem. Soc., Dalton Trans., 1986, 1551 RSC;
(e) D. E. Goldberg, P. B. Hitchcock, M. F. Lappert, K. M. Thomas, A. J. Thorne, T. Fjeldberg, A. Haaland and B. E. R. Schilling, J. Chem. Soc., Dalton Trans., 1986, 2387 RSC;
(f) L. M. Engelhardt, B. S. Jolly, M. F. Lappert, C. L. Raston and A. H. White, J. Chem. Soc., Chem. Commun., 1988, 336 RSC.
- For reviews on stannylenes see:
(a) M. Veith, Angew. Chem., Int. Ed. Engl., 1987, 26, 1 CrossRef;
(b) W. P. Neumann, Chem. Rev., 1991, 91, 311 CrossRef CAS;
(c) H. V. R. Dias, Z. Wang and W. Jin, Coord. Chem. Rev., 1998, 176, 67 CrossRef CAS;
(d) N. Tokitoh and R. Okazaki, Coord. Chem. Rev., 2000, 210, 251 CrossRef CAS;
(e) O. Kühl, Coord. Chem. Rev., 2004, 248, 411 CrossRef;
(f) I. Saur, S. G. Alonso and J. Barrau, Appl. Organomet. Chem., 2005, 19, 414 CrossRef CAS;
(g) W.-P. Leung, K.-W. Kan and K.-H. Chong, Coord. Chem. Rev., 2007, 251, 2253 CrossRef CAS;
(h) S. Nagendran and H. W. Roesky, Organometallics, 2008, 27, 457 CrossRef CAS;
(i) A. V. Zabula and F. E. Hahn, Eur. J. Inorg. Chem., 2008, 5165 CrossRef CAS;
(j) M. Asay, C. Jones and M. Driess, Chem. Rev., 2011, 111, 354 CrossRef CAS PubMed;
(k) G. He, O. Shynkaruk, M. W. Lui and E. Rivard, Chem. Rev., 2014, 114, 7815 CrossRef CAS PubMed.
- For reviews on stannocenes see:
(a) P. Jutzi, Chem. Rev., 1986, 86, 983 CrossRef CAS;
(b) P. Jutzi and N. Burford, Chem. Rev., 1999, 99, 969 CrossRef CAS PubMed;
(c) P. Jutzi, Chem. Unserer Zeit, 1999, 33, 342 CrossRef CAS;
(d) P. Jutzi and G. Reumann, J. Chem. Soc., Dalton Trans., 2000, 2237 RSC.
- K. D. Bos, E. J. Bulten and J. G. Noltes, J. Organomet. Chem., 1972, 39, C52 CrossRef CAS.
-
(a) D. Stalke, M. A. Paver and D. S. Wright, Angew. Chem., Int. Ed. Engl., 1993, 32, 428 CrossRef;
(b) M. A. Paver, C. A. Russell, D. Stalke and D. S. Wright, J. Chem. Soc., Chem. Commun., 1993, 1349 RSC;
(c) M. Veith, C. Mathur and V. Huch, Organometallics, 1996, 15, 2858 CrossRef CAS;
(d) G. M. de Lima, H. G. L. Siebald, J. L. Neto and V. D. de Castro, Main Group Met. Chem., 2001, 24, 223 CAS;
(e) J. N. Jones, J. A. Moore, A. H. Cowley and C. L. B. Macdonald, Dalton Trans., 2005, 3846 RSC;
(f) W.-P. Leung, K.-P. Chan, K.-W. Kan and T. C. W. Mak, Organometallics, 2008, 27, 2767 CrossRef CAS;
(g) E. Y. Njua, A. Steiner and L. Stahl, J. Organomet. Chem., 2011, 696, 3301 CrossRef CAS;
(h) O. T. Summerscales, J. C. Fettinger and P. P. Power, J. Am. Chem. Soc., 2011, 133, 11960 CrossRef CAS PubMed.
- A. J. Edwards, M. A. Paver, P. R. Raithby, M.-A. Rennie, C. A. Russell and D. S. Wright, J. Chem. Soc., Dalton Trans., 1995, 1587 RSC.
-
(a) M. Tamm, S. Randoll, T. Bannenberg and E. Herdtweck, Chem. Commun., 2004, 876 RSC;
(b) S. Randoll, P. G. Jones and M. Tamm, Organometallics, 2008, 27, 3232 CrossRef CAS;
(c) B. Haberlag, X. Wu, K. Brandhorst, J. Grunenberg, C. G. Daniliuc, P. G. Jones and M. Tamm, Chem.–Eur. J., 2010, 16, 8868 CrossRef CAS PubMed;
(d) X. Wu and M. Tamm, Coord. Chem. Rev., 2014, 260, 116 CrossRef CAS;
(e) K. Nomura, B. K. Bahuleyan, S. Zhang, P. M. V. Sharma, S. Katao, A. Igarashi, A. Inagaki and M. Tamm, Inorg. Chem., 2014, 53, 607 CrossRef CAS PubMed.
-
(a) D. Franz and S. Inoue, Dalton Trans., 2016, 45, 9385 RSC;
(b) T. Ochiai, D. Franz and S. Inoue, Chem. Soc. Rev., 2016, 45, 6327 RSC.
-
(a) D. Franz and S. Inoue, Chem.–Asian J., 2014, 9, 2083 CrossRef CAS PubMed;
(b) D. Franz, E. Irran and S. Inoue, Angew. Chem., Int. Ed., 2014, 53, 14264 CrossRef CAS PubMed;
(c) M. W. Lui, N. R. Paisley, R. McDonald, M. J. Ferguson and E. Rivard, Chem.–Eur. J., 2016, 22, 2134 CrossRef CAS PubMed.
-
(a) D. Franz, E. Irran and S. Inoue, Dalton Trans., 2014, 43, 4451 RSC;
(b) D. Franz and S. Inoue, Chem.–Eur. J., 2014, 20, 10645 CrossRef CAS PubMed;
(c) D. Franz, T. Szilvási, E. Irran and S. Inoue, Nat. Commun., 2015, 6, 10037 CrossRef CAS PubMed;
(d) A. D. K. Todd, W. L. McClennan and J. D. Masuda, RSC Adv., 2016, 6, 69270 RSC;
(e) D. Franz, L. Sirtl, A. Pöthig and S. Inoue, Z. Anorg. Allg. Chem., 2016, 642, 1245 CrossRef CAS.
-
(a) S. Inoue and K. Leszczyńska, Angew. Chem., Int. Ed., 2012, 51, 8589 CrossRef CAS PubMed;
(b) T. Ochiai, T. Szilvási and S. Inoue, Molecules, 2016, 21, 1155 CrossRef PubMed.
-
(a) M. W. Lui, C. Merten, M. J. Ferguson, R. McDonald, Y. Xu and E. Rivard, Inorg. Chem., 2015, 54, 2040 CrossRef CAS PubMed;
(b) T. Ochiai, D. Franz, X.-N. Wu and S. Inoue, Dalton Trans., 2015, 44, 10952 RSC;
(c) T. Ochiai, T. Szilvási, D. Franz, E. Irran and S. Inoue, Angew. Chem., Int. Ed., 2016, 55, 11619 CrossRef CAS PubMed.
-
(a) T. Ochiai, D. Franz, E. Irran and S. Inoue, Chem.–Eur. J., 2015, 21, 6704 CrossRef CAS PubMed;
(b) T. Ochiai, D. Franz, X.-N. Wu, E. Irran and S. Inoue, Angew. Chem.,
Int. Ed., 2016, 55, 6983 CAS.
-
(a) R. Kinjo, B. Donnadieu and G. Bertrand, Angew. Chem., Int. Ed., 2010, 49, 5930 CrossRef CAS PubMed;
(b) F. Dielmann, O. Back, M. Henry-Ellinger, P. Jerabek, G. Frenking and G. Bertrand, Science, 2012, 337, 1526 CrossRef CAS PubMed;
(c) O. Back, B. Donnadieu, M. von Hopffgarten, S. Klein, R. Tonner, G. Frenking and G. Bertrand, Chem. Sci., 2011, 2, 858 RSC;
(d) F. Dielmann, C. E. Moore, A. L. Rheingold and G. Bertrand, J. Am. Chem. Soc., 2013, 135, 14071 CrossRef CAS PubMed;
(e) Y. KaiLoh, C. Gurnani, R. Ganguly and D. Vidović, Inorg. Chem., 2015, 54, 3087 CrossRef PubMed;
(f) C. C. Chong, R. Ganguly, Y. Li and R. Kinjo, Z. Anorg. Allg. Chem., 2016, 642, 1264 CrossRef CAS.
- F. H. Allen, O. Kennard, D. G. Watson, L. Brammer, A. G. Orpen and R. Taylor, J. Chem. Soc., Perkin Trans. 2, 1987, S1 RSC.
- B. Wrackmeyer, Annu. Rep. NMR Spectrosc., 1999, 38, 203 CrossRef CAS.
- M. F. Lappert, M. C. Misra, M. Onyszchuk, R. S. Rowe, P. P. Power and M. J. Slade, J. Organomet. Chem., 1987, 330, 31 CrossRef CAS.
- Y. Peng, J.-D. Guo, B. D. Ellis, Z. Zhu, J. C. Fettinger, S. Nagase and P. P. Power, J. Am. Chem. Soc., 2009, 131, 16272 CrossRef CAS PubMed.
- P. P. Samuel, A. P. Singh, S. P. Sarish, J. Matussek, I. Objartel, H. W. Roesky and D. Stalke, Inorg. Chem., 2013, 52, 1544 CrossRef CAS PubMed.
- T. Ochiai, PhD thesis, Technische Universität Berlin, Berlin, 2016.
Footnote |
† Electronic supplementary information (ESI) available: Experimental details, crystallographic data and details to the DFT calculations. CCDC 1515071 for 1 and 1515070 for 3. For ESI and crystallographic data in CIF or other electronic format see DOI: 10.1039/c6ra27697k |
|
This journal is © The Royal Society of Chemistry 2017 |
Click here to see how this site uses Cookies. View our privacy policy here.