DOI:
10.1039/C6RA26727K
(Paper)
RSC Adv., 2017,
7, 5843-5852
Corrosion behavior and cytocompatibility of a Co–Cr and two Ni–Cr dental alloys before and after the pretreatment with a biological saline solution
Received
13th November 2016
, Accepted 8th January 2017
First published on 17th January 2017
Abstract
Objectives. The aim of this study was to evaluate the corrosion behavior and cytocompatibility of a Co–Cr and two Ni–Cr dental alloys before and after the pretreatment with a biological saline solution. Methods. A commercial cobalt–chromium (Co–Cr) and two nickel–chromium (Ni–Cr) dental alloys [beryllium (Be)-free and Be-containing] were selected and pretreated with a biological saline solution containing 3% bovine serum albumin (BSA) for 72 h. Before and after the pretreatment, alloy specimens were examined for surface element compositions using X-ray photoelectron spectroscopy (XPS). Corrosion behavior and metal ion release were measured by electrochemical corrosion and immersion tests in artificial saliva respectively. The released metal ions in the exposed biological saline solution were also detected after the pretreatment for 72 h. 3T3 fibroblasts were cultured and exposed to specimens. After 4 hours of incubation, cell morphology and spreading were observed under a laser scanning confocal microscope. Cell proliferations were evaluated using CCK-8 assay after culturing for 1, 3 and 6 days. Results. After the pretreatment with a biological saline solution, the corrosion potential (Ecorr) and breakdown potential (Ebr) of the three dental alloys increased significantly, which corresponded with evident decreases of Co, Ni and Be levels on the alloy surfaces measured by XPS. The corrosion current (Icorr) and the polarization resistance (Rp) of the three alloys were not significantly affected by the pretreatment, corresponding to the slight reductions of Cr, molybdenum (Mo) and oxygen (O) levels on the surfaces of alloys except the Be-containing alloy. For the three alloys, the pretreatment obviously decreased the metal ions release in artificial saliva. There were a large amount of metal ions, including Co, Ni and Be ions, released from the alloys in the exposed biological saline solution after the pretreatment for 72 h. The results of in vitro study demonstrated that the pretreatment up-regulated cell spreading and proliferation on the three metallic substrates. Conclusions. The pretreatment with a biological saline solution optimized the surface elemental compositions of a Co–Cr and two Ni–Cr dental alloys by removing more labile metal elements from the alloy surfaces in form of cations. The corrosion susceptibility and metal ion release of the three dental alloys decreased significantly after the pretreatment, which led to improvements of their cytocompatibility.
1. Introduction
For aesthetic purposes, dental alloys used in fixed prostheses may have a porcelain veneer fired onto the cast substructure. Cobalt–chromium (Co–Cr) and nickel–chromium (Ni–Cr) dental alloys are commonly used as substructures for metal-ceramic restorations in dentistry.1 Compared with precious dental alloys, these non-precious alloys offer the advantage of an increased modulus of elasticity that allows thinner substructures to be used, and thus less destruction of tooth tissue during the tooth preparation for crowns.
However, corrosion behavior of dental alloys occurs in the oral cavity due to the complex biological and electrolyte environment. The metal ions that release during the corrosion processes are toxic to the surrounding tissues, and the biological response to particular metal ions can be evaluated in vitro using cell culture techniques.2 Nickel and cobalt ions are the primary elements released from Ni-based and Co-based alloys; the other elements are released at much lower concentrations.3,4 Our recent studies found that the corrosion rate and metal ions (Co and Ni) release of both Ni-based and Co-based dental alloys increased significantly after a simulated porcelain firing, which, in turn, increased the cytotoxicity of these base-metal alloys.5–7 Therefore, the released ions (Co and Ni) in the gingival crevicular fluid jeopardize periodontal health and cause gingival inflammation or discoloration.8,9
Since a large number of casting alloys used for clinical applications in restorative dentistry, current studies have been focused on the cytotoxicity and cytocompatibility of such metallic biomaterials. Nelson et al.10,11 have proposed the utility of a short-term pre-exposure of dental alloys for 72 or 168 h to either saline solution, saline/bovine serum albumin (BSA) solution or cell culture medium. Among three biological solutions, preconditioning with the saline/BSA solution had best effect to reduce cytotoxicity of the tested precious metal alloys. Sandrucci et al.12 also reported that the pretreatment with saline/BSA solution or cell culture medium for 72 h reduced the released Ag+ and Cu2+ from dental precious alloys and improved their cytocompatibility in vitro. The rationale for these procedure have been based on the possibility of the preconditioning treatment to remove more ionizable metal elements from alloy surfaces, as to decrease alloy cytotoxicity.
Although improvements of cytocompatibility of dental precious alloys after the pretreatment had been reported, few studies to date have evaluated the preconditioning effectiveness for non-precious dental alloys,10 in particular for Co–Cr and Ni–Cr alloys, which generally have higher cytotoxicity. Moreover, the changes in detail of surface elements and their influences on corrosion properties of dental alloys, which closely correlate with the cytocompatibility, have not been further studied so far. During recent years, electrochemical corrosion test had been proven to be advantageous for corrosion characterization of metallic biomaterials compared with traditional immersing test.6,13 Also, surface chemistry of metal oxides can be discriminated and analyzed by X-ray photoelectron spectroscopy (XPS) wide- and high-resolution spectra.14 Such information would promote the understanding of effects of the pretreatment process on dental alloys. Therefore, the aim of the present study was to evaluate the effects of the pretreatment with a biologic saline solution on the surface elements, corrosion properties and cellular responses of a Co–Cr and two Ni–Cr dental alloys.
2. Materials and methods
2.1 Materials and specimen preparation
One commercial Co–Cr dental alloy (Wirobond C, Bego Dental, Bremen, Germany), and two Ni–Cr dental alloys that comprised a high Cr, Mo alloy without Be (Stellite N9, Shanghai Stellite, Shanghai, PR China) and a low Cr, Mo alloy with added Be (ChangPing, Shanghai ChangPing, Shanghai, PR China) were used in this study. The compositions of these alloys, as given by the manufacturers, are shown in Table 1. All three alloys can be used for fabricating PFM restorations.
Table 1 Compositions of the three base-metal dental alloysa
Alloy |
Composition (wt%) |
Co |
Ni |
Cr |
Mo |
W |
Al |
Be |
Other elements |
Information supplied by the manufacturers. |
Wirobond C (Co–Cr) |
63.3 |
— |
24.8 |
5.1 |
5.3 |
— |
— |
Si, Fe, Ce each < 1 |
Stellite N9 (Ni–Cr) |
— |
64 |
22.5 |
9.5 |
— |
1 |
— |
Nb 1, Si 1, Fe 0.5, Ce 0.5 |
ChangPing (Ni–Cr–Be) |
— |
76.5 |
14 |
4.5 |
— |
2.5 |
2 |
Fe 0.5 |
A total of 78 specimens (10 mm diameter and 3 mm thickness), 26 specimens for each alloy, were fabricated by a flame casting method using oxygen–propane gas mixture in accordance with the manufacturers' recommendations. The same technician made all castings in a dental laboratory. To simulate the application of a porcelain veneer, all specimens were subjected to a PFM firing cycle under vacuum in a dental porcelain furnace. Briefly, the specimens were degassed at 1010 °C under vacuum holding for 5 min, opaque fired at 980 °C under vacuum and air cooled, body fired at 970 °C under vacuum and air cooled, and finally glaze fired at 980 °C and air cooled.6 After firing, using a grinder-polisher machine (Beta, Buehler Ltd., Lake Bluff, IL, USA), the casting specimens were wet grinded with a series of silicon carbide papers (180, 400, 600, and 1200 grit) and then polished with 3 μm diamond suspension (Metadi, Buehler Ltd., USA). After that, the specimens were ultrasonically cleaned in ethanol, and then in de-ionized water.
2.2 Preconditioning treatments
Half of all metal specimens, selected using a table of random numbers, were subjected to the preconditioning treatment using a biological saline solution as the experiment group. The other half of all specimens did not undergo any preconditioning treatment as the control group.
Preconditioning treatment was performed by immersing each specimen into 3 ml of a biological saline solution containing 0.9% NaCl added with 3% (wt vol−1) bovine serum albumin (BSA, Sigma, St. Louis, MO, USA) and by leaving them there undisturbed for 72 h in a fully humidified air atmosphere containing 5% CO2 at 37 °C.11,12 Finally, the metal specimens were placed on sterile gauze to remove residual preconditioning solution, and ultrasonically rinsed in sterile water.
2.3 Surface analysis
X-ray photoelectron spectroscopy (XPS) was used to determine the elemental components and chemical states present on the surfaces of three specimens of each alloy before and after the pretreatment. XPS was performed (Axis Ultra DLD surface analysis system, Kratos Analytical, Hadano, Japan) utilizing a monochromatic Al Kα electrode at 15 kV and 150 W at a 45° take-off angle. Survey and high-resolution spectra were obtained using pass energies of 160 and 40 eV, respectively. Reference binding energies of each element were obtained from the National Institute of Standards and Technology XPS Online Database (http://srdata.nist.gov/xps/). All spectral features were referenced to the binding energy of adventitious carbon (284.8 eV). Quantitative analysis of the surface chemical composition was performed using the peak areas and atomic sensitivity factors.
2.4 Electrochemical corrosion test
Before testing, the metal specimens were carefully mounted in self-cured epoxy resin, exposing their surfaces, and ultrasonically cleaned in ethanol, and then in de-ionized water. Corrosion tests were performed using an electrochemical potentiostat (PARSTAT 2273, Princeton Applied Research, Oak Ridge, TN, USA) via a test cell with the mounted specimen as the working electrode, a high-purity platinum wire as the counter electrode, and Ag/AgCl as the reference electrode. Corrosion tests were performed in triplicate for each alloy, before and after the pretreatment, in Fusayama artificial saliva solution (0.4 g l−1 NaCl, 0.4 g l−1 KCl, 0.795 g l−1 CaCl2·2H2O, 0.690 g l−1 NaH2PO4·H2O, 0.005 g l−1 Na2S·9H2O, 1.0 g l−1 urea, pH = 5.0),15 at 37 ± 0.5 °C. Each specimen was allowed to reach a steady open circuit potential (Ecorr) for 2 h. The Ecorr was recorded and then a potentiodynamic test was initiated within a scanning range from −400 mV to +1600 mV (versus reference electrode) at a sweep rate of 1 mV s−1. The acquired polarization curves were analyzed using the curve-fitting routine of the dedicated PowerSuite software (Princeton Applied Research, USA) to calculate the corrosion current (Icorr) and the polarization resistance (Rp) of the alloys. The breakdown potential (Ebr), at which the corrosion current increases abruptly, was also estimated from the polarization curves. Icorr and Rp represent the corrosion rate and corrosion resistance, respectively. Because the breakdown of passive oxide film, marked by a large and generally increasing current, is caused by localized or pitting-type corrosion, the corrosion resistance of the alloys can also be assessed by using the breakdown potential, which means that a greater Ebr value indicates a better resistance to pitting-type corrosion.16
2.5 Measurement of metal ions release
Immersion tests were performed to evaluate the types and amounts of metal ions released from the alloys. Three specimens of each alloy before and after the pretreatment were immersed in artificial saliva solutions. Specimens were incubated in closed sterile centrifuge tubes at 37 °C for 7 day.6 At the end of this period, the specimens were removed and the artificial saliva solutions were analyzed for metal ions release by inductively coupled plasma atomic emission spectrometry (ICP-AES Vista AX, Varian Inc., Palo Alto, CA, USA). In addition, after the pretreatment for 72 h, the biological saline solutions exposed to the specimens of the three alloys were detected for released metal ions by ICP-AES.
2.6 Cell culture studies
NIH 3T3 mouse fibroblasts, obtained from the Cell Bank of the Chinese Academy of Sciences (Shanghai, China), were cultured in Dulbecco's Modified Eagle's Medium (DMEM, Sigma-Aldrich LLC, St. Louis, MO). For cell spreading assay, 3T3 mouse fibroblasts (5 × 103 cells per well) were seeded on one specimen of each alloy before and after the pretreatment. After culturing cells for 4 hours, each sample was rinsed with PBS, and then fixed with 4% paraformaldehyde in PBS at room temperature for 10 minutes.17–19 Afterwards, each sample was stained with Rhodamine phalloidin (Cytoskeleton, USA) at room temperature in the dark for 30 minutes and then 4′,6′-diamidino-2-phenylindole (DAPI) (Beyotime, Shanghai, China) for 30 seconds. The cell spreading morphology in three random fields on each sample were observed under a laser scanning confocal microscope (LSM710, Zeiss, GER) at 200× magnification.
To compare the cell proliferation rates, the cells were exposed indirectly to the alloys. Three specimens of each alloy before and after the pretreatment were immersed in DMEM for 7 days. After that, the cells were seeded at 3 × 103 cells per cm2 into a 96-well culture plate containing the exposed medium and incubated in a 5% CO2 humidified environment at 37 °C, replacing the culture medium after 3 days. After 1, 3 and 6 days, the cell proliferation was investigated using a CCK-8 assay. The absorbance of each solution was measured by a microplate reader (Spectramax190, MD, USA) at 450 nm wavelength. All the cell experimental investigations were performed in triplicate compared with control cultures using medium not exposed to alloys.
2.7 Statistical analysis
Data from the three base-metal dental alloys for corrosion test, metal ion release and cell proliferation were statistically analyzed by SPSS 22.0 software (SPSS, Inc., Chicago, IL, USA) using standard analysis of variance (ANOVA). The Student's independent-samples t-test was used to compare differences in numerical results before and after the pretreatment. The probability level for statistical significance was set at α = 0.05.
3. Results
3.1 Surface analysis
The representative XPS survey spectra of Wirobond C (Co–Cr), Stellite N9 (Ni–Cr) and ChangPing (Ni–Cr–Be) alloys are shown in Fig. 1(a, c and e). Cr, Mo, and O (and if present in the alloy composition, Co, Ni, Be and other elements) were shown to be present on the alloy surfaces. Adventitious C and N peaks originated from the laboratory environment. Fig. 1(b, d and f) compares the relative composition of elements on the alloy surfaces. For Wirobond C, the relative level of Co reduced evidently after the pretreatment, and the levels of Cr, Mo, W and O showed little changes (Fig. 1b). After the pretreatment, Stellite N9 exhibited an evident decrease in the level of Ni and slight decreases in the levels of Cr, Mo, and O (Fig. 1d), while ChangPing exhibited decreases in the levels of elements, especially Ni and Be (Fig. 1f).
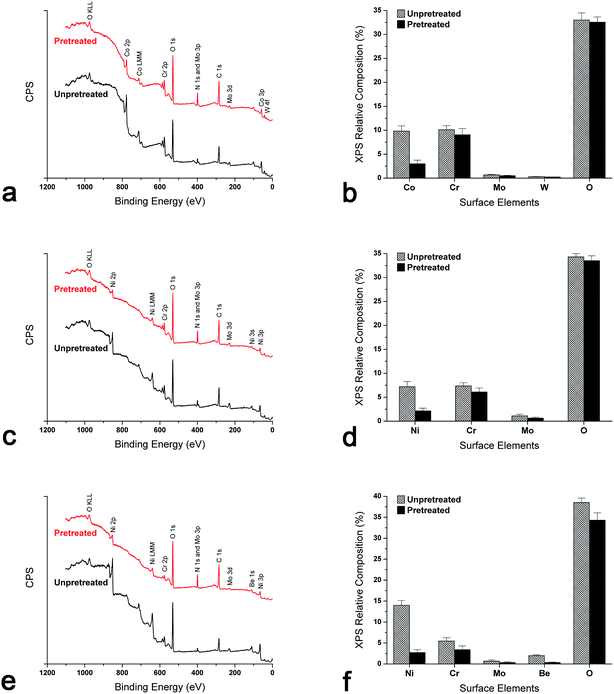 |
| Fig. 1 Representative XPS survey spectra for (a) Wirobond C (Co–Cr), (c) Stellite N9 (Ni–Cr), and (e) ChangPing (Ni–Cr–Be) alloys before and after the pretreatment. Relative compositions of surface elements for (b) Wirobond C, (d) Stellite N9, and (f) ChangPing alloys before and after the pretreatment. | |
The representative XPS high-resolution spectra of Wirobond C (Co–Cr), Stellite N9 (Ni–Cr) and ChangPing (Ni–Cr–Be) alloys are presented in Fig. 2. The complex peaks of Co 2p, Ni 2p and Be 1s exhibited binding energies that correlated with the presence of Co3O4, NiO and BeO as well as Co0, Ni0 and Be0, respectively. As shown in Fig. 2(a and b), Wirobond C exhibited reductions of Co3O4 and Co0 sub-peaks indicating decreases of Co3O4 and metallic Co levels after the pretreatment, which led to a decrease of Co 2p peak. The proportions of sub-peaks were similar in both conditions. As shown in Fig. 2(c–f), after the pretreatment, both Stellite N9 and ChangPing exhibited decreases of NiO and Ni0 sub-peaks indicating decreases of NiO and metallic Ni levels, which caused reductions of Ni 2p peaks. The sub-peaks in Ni 2p peaks revealed different proportions after the pretreatment. For Stellite N9, NiO obviously increased from 26.2% to 64.5%, whereas Ni0 evidently decreased from 73.8% to 35.5%. For ChangPing, NiO increased from 61.1% to 70.3%, while Ni0 decreased from 38.9% to 29.7%. As shown in Fig. 2(g and h), after the pretreatment, ChangPing also exhibited reductions of BeO and Be0 sub-peaks indicating decreases of BeO and metallic Be levels, which led to a decrease of Be 1s peak. The sub-peaks in Be 1s peak revealed different proportions after the pretreatment. BeO increased from 53.8% to 61.4%, whereas Be0 decreased from 46.2% to 38.6%.
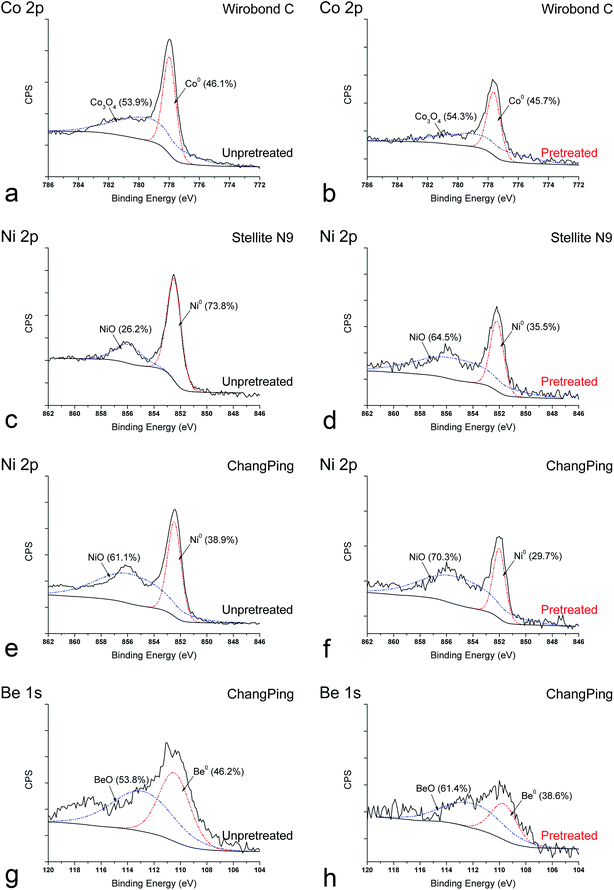 |
| Fig. 2 Representative XPS high-resolution spectra of Co 2p, Ni 2p, and Be 1s for Wirobond C (Co–Cr), Stellite N9 (Ni–Cr), and ChangPing (Ni–Cr–Be) alloys before and after the pretreatment. | |
3.2 Corrosion behavior
The representative open circuit potential and potentiodynamic curves to show typical corrosion behaviors of Wirobond C (Co–Cr), Stellite N9 (Ni–Cr) and ChangPing (Ni–Cr–Be) alloys before and after the pretreatment are illustrated in Fig. 3. The open circuit potential (Ecorr), corrosion current (Icorr), polarization resistance (Rp) and breakdown potential (Ebr) of the three alloys, as determined through electrochemical corrosion curves, are shown in Table 2. For Wirobond C, before and after the pretreatment, Ecorr ranged from −76.7 to −45.2 mV, Icorr from 0.31 to 0.24 μA cm−2, Rp from 203.7 to 268.9 kΩ cm−2, Ebr from 810.7 to 1273.3 mV. For Stellite N9, Ecorr ranged from −185.7 to −63.4 mV, Icorr from 0.34 to 0.27 μA cm−2, Rp from 193.4 to 190.4 kΩ cm−2, Ebr from 797.7 to 1123.3 mV. For ChangPing, Ecorr ranged from −204.7 to −64.3 mV, Icorr from 0.41 to 0.32 μA cm−2, Rp from 179.7 to 213.6 kΩ cm−2, Ebr from 514.3 to 876.0 mV. After the pretreatment, the three alloys exhibited statistically higher Ecorr and Ebr values (P < 0.05), which indicated decreases of corrosion susceptibility and increases of pitting corrosion resistance, respectively. Though not significant (P > 0.05), the pretreatment led to slight changes in Icorr and Rp values for the three alloys.
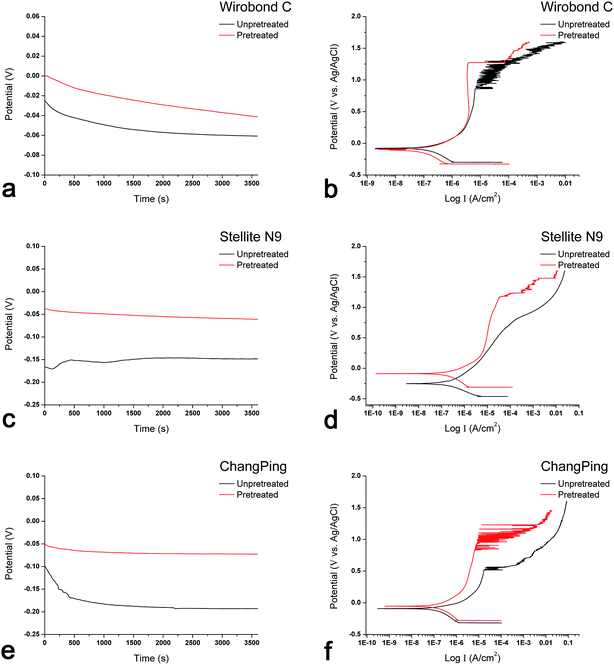 |
| Fig. 3 Representative open circuit potential curves for (a) Wirobond C (Co–Cr), (c) Stellite N9 (Ni–Cr), and (e) ChangPing (Ni–Cr–Be) alloys before and after the pretreatment. Representative potentiodynamic curves for (b) Wirobond C, (d) Stellite N9, and (f) ChangPing alloys before and after the pretreatment. | |
Table 2 Comparison of corrosion parameter values for the three base-metal dental alloys before and after the pretreatmenta
Alloy |
Condition |
Corrosion parameters (n = 3) |
Ecorr (mV) |
Icorr (μA cm−2) |
Rp (kΩ cm−2) |
Ebr (mV) |
Wirobond C: Co–Cr alloy; Stellite N9: Ni–Cr alloy; ChangPing: Ni–Cr–Be alloy; Ecorr: open circuit potential; Icorr: corrosion current; Rp: polarization resistance; Ebr: breakdown potential. *Indicates a statistical difference between the unpretreated and pretreated conditions (P < 0.05). |
Wirobond C |
Unpretreated |
−76.7 (15.7) |
0.31 (0.09) |
203.7 (82.8) |
810.7 (127.6) |
Pretreated |
−45.2 (3.5)* |
0.24 (0.12) |
268.9 (193.2) |
1273.3 (55.1)* |
Stellite N9 |
Unpretreated |
−185.7 (32.0) |
0.34 (0.24) |
193.4 (54.1) |
797.7 (13.7) |
Pretreated |
−63.4 (5.1)* |
0.27 (0.05) |
190.4 (71.8) |
1123.3 (86.2)* |
ChangPing |
Unpretreated |
−204.7 (17.7) |
0.41 (0.13) |
179.7 (59.4) |
514.3 (15.0) |
Pretreated |
−64.3 (7.2)* |
0.32 (0.11) |
213.6 (75.7) |
876.0 (205.9)* |
3.3 Metal ion release
Fig. 4 shows the quantity of metal ions released over 7 days into the artificial saliva from Wirobond C (Co–Cr), Stellite N9 (Ni–Cr) and ChangPing (Ni–Cr–Be) alloys before and after the pretreatment. Generally, total metal ion release from the three alloys decreased after the pretreatment. The release of Cr ion was below the detection limit for the alloys in both conditions. Wirobond C exhibited significant reductions in the ions release of Co and Mo after the pretreatment (P < 0.05), ranging from 6.594 to 1.313 μg cm−2 and from 0.417 to 0.156 μg cm−2, respectively (Fig. 4a and c). For both Stellite N9 and ChangPing, the ions release of Ni (ranging from 2.667 to 0.563 μg cm−2, from 3.740 to 1.958 μg cm−2, respectively) and Mo (ranging from 0.750 to 0.188 μg cm−2, from 0.222 to 0 μg cm−2, respectively) decreased significantly after the pretreatment (P < 0.05) (Fig. 4b and c). The ion release of Be also decreased significantly for ChangPing after the pretreatment (P < 0.05), ranging from 0.069 to 0.010 μg cm−2 (Fig. 4d). As shown in Fig. 5, after the pretreatment for 72 h, a large amount of Co (6.13 μg cm−2), Ni (1.63 μg cm−2 for Stellite N9, 11.98 μg cm−2 for ChangPing), Mo (1.40 μg cm−2 for Wirobond C, 0.38 μg cm−2 for Stellite N9, 0.20 μg cm−2 for ChangPing), and Be (1.64 μg cm−2) ions released from the specimens of the three alloys were detected in the exposed biological saline solutions. The release of Cr ion was still below the detection limit.
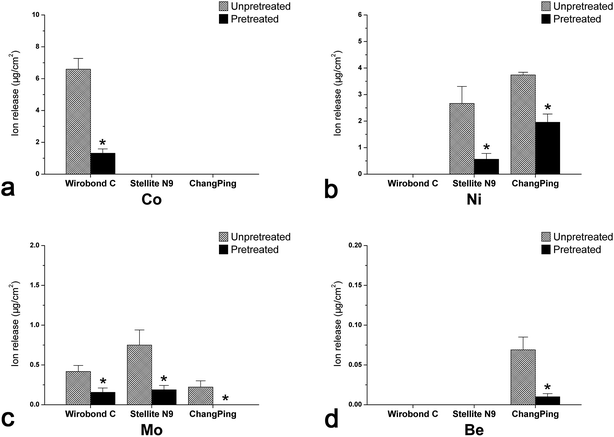 |
| Fig. 4 Total metal ions released into the artificial saliva over 7 days from Wirobond C (Co–Cr), Stellite N9 (Ni–Cr), and ChangPing (Ni–Cr–Be) alloys before and after the pretreatment. Error bars indicate standard deviations (n = 3). *Indicates a statistical difference for each alloy before and after the pretreatment (P < 0.05). | |
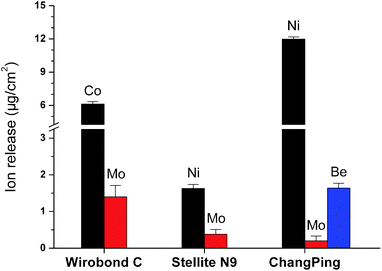 |
| Fig. 5 Total metal ions released into the biological saline solutions exposed to Wirobond C (Co–Cr), Stellite N9 (Ni–Cr), and ChangPing (Ni–Cr–Be) alloys after the pretreatment for 72 h. | |
3.4 Cell spreading
After culturing for 4 hours, differences in NIH 3T3 cell spreading morphology on the specimens of Wirobond C (Co–Cr), Stellite N9 (Ni–Cr) and ChangPing (Ni–Cr–Be) alloys before and after the pretreatment could be observed (Fig. 6). After the pretreatment, the cells spread more evenly across alloy surfaces and extended more pseudopodia on three substrates. There was no significant difference in the cell spreading between the three alloys surfaces after the pretreatment.
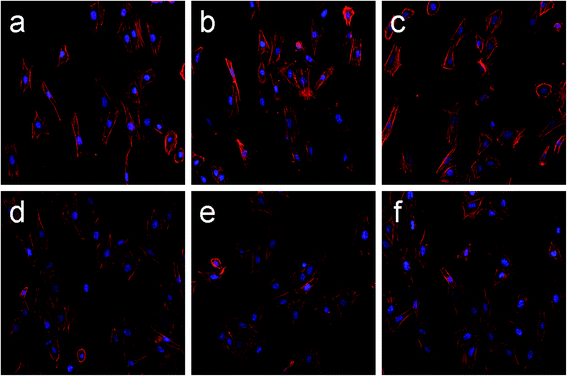 |
| Fig. 6 Fluorescence images (magnification 200×) of NIH 3T3 fibroblasts spreading after 4 h of incubation on Wirobond C (Co–Cr alloy) before (a) and after (d) the pretreatment. Stellite N9 (Ni–Cr alloy) before (b) and after (e) the pretreatment. ChangPing (Ni–Cr–Be alloy) before (c) and after (f) the pretreatment. | |
3.5 Cell proliferation
Cell proliferation measured by CCK-8 assay is shown in Fig. 7. As time went on, NIH 3T3 cells proliferated on all specimens, indicating excellent cell viabilities. After the pretreatment, significant increases of cell proliferation could be observed on Wirobond C (Co–Cr), Stellite N9 (Ni–Cr) and ChangPing (Ni–Cr–Be) alloys after culturing for 3 and 6 days (P < 0.05). The results demonstrated that the three pretreated alloys offered more favorable environment for cell proliferation than those without the pretreatment.
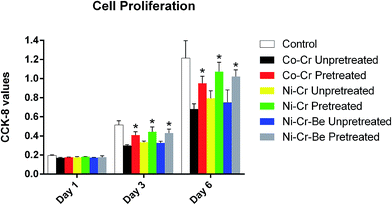 |
| Fig. 7 After culturing for 1, 3 and 6 days, cell proliferation of NIH 3T3 cells on three substrates of Wirobond C (Co–Cr), Stellite N9 (Ni–Cr), and ChangPing (Ni–Cr–Be) alloys before and after the pretreatment was measured by CCK-8 assay. Error bars indicate standard deviations (n = 3). *Indicates a statistical difference for each alloy before and after the pretreatment (P < 0.05). | |
4. Discussion
Previous studies have reported that preconditioning treatments of dental alloys with protein-containing biological solutions could significantly reduce alloy cytotoxicity by inducing a relevant release of ionized metal elements.10–12 Cytotoxicity of dental alloys is intimately related to their corrosion behaviors, which rely on the alloy surface oxides.20 However, up to date, few studies have addressed the influences of such preconditioning treatments on alloy surface and corrosion property. Such pretreatments may alter the composition of the surface oxides that may, in turn, alter the alloy's corrosion behavior and subsequent cell responses. In this work, effects of the pretreatment with a biological saline solution on the surface elements, corrosion property and cytocompatibility of a Co–Cr and two Ni–Cr dental alloys have been evaluated.
Co–Cr and Ni–Cr dental alloys depend on a surface oxide layer for corrosion resistance in the oral environment.21,22 Metal oxides on the alloy surfaces are formed spontaneously because of the rapid O uptake from the atmosphere after polishing.23 In this study, the oxides of Co, Ni, Cr, Mo, and other alloying elements on three alloy surfaces were found by XPS (Fig. 1). Previous studies had reported that the surface oxide layers formed on Co-based and Ni-based alloys were composed predominantly of Cr oxides and less Co and Ni oxides respectively.24–26 Regarding the surface oxide layer, the outer layer was Co-rich or Ni-rich oxides mixed with metallic Co or Ni, and the predominant inner one was compact Cr-rich oxides.26,27 As is well known, the compact Cr oxides act as a vital role in the corrosion resistance for non-precious dental alloys in the electrolyte.5–7,28 After the pretreatment, in the current study, the XPS examination of Wirobond C (Co–Cr) and Stellite N9 (Be-free Ni–Cr) alloys demonstrated evident reductions in the levels of Co and Ni respectively, which originated from the reductions of metallic Co or Ni and their oxides, as shown in the XPS survey and high-resolution spectra (Fig. 1 and 2). This result indicated that the pretreatment reduced the metallic Co or Ni and delayed the growth of the outer oxide layer on the two alloy surfaces. However, after the pretreatment, the XPS data revealed little, if any, changes in the relative amounts of Cr and O on both alloy surfaces, which indicated that the inner Cr-rich oxide layer retained compact and continuous. Thus, for Wirobond C and Stellite N9, changes of the surface oxide layer involved primarily in its outer part after the pretreatment. The optimized outer oxide layer containing less metallic Co or Ni, which are more liable, might lead to the decreases of corrosion susceptibility. This presumption was confirmed by the results of corrosion tests shown in Table 2, where both Wirobond C and Stellite N9 exhibited significantly higher Ecorr and Ebr values after the pretreatment, and, though not significant, Icorr values decreased slightly.
As for ChangPing (Be-containing Ni–Cr) alloy, after the pretreatment, the XPS results exhibited reductions in the levels of elements, especially Ni and Be (Fig. 1). Furthermore, as shown in the XPS high-resolution spectra for Ni 2p and Be 1s (Fig. 2), the percentage for metallic Ni decreased from 38.9% to 29.7% and that for metallic Be from 46.2% to 38.6%. It was reported that the corrosion resistance of Ni–Cr alloys was reduced by as little as 0.6 wt% Be content.29,30 In the present study, the ChangPing alloy had a 2.0 wt% Be content, which was considered to be negative for its corrosion resistance. The small atomic radius of Be facilitated its migration to the alloy surface.25 Due to the surface migration of Be, Be-containing Ni–Cr alloys were reported to form the Ni–Be eutectic phase that induced the development of non-homogeneous Cr surface oxides and depleted areas of Cr, which were less corrosion resistant.31 Therefore, after the pretreatment, although the surface oxides of the ChangPing alloy decreased, evident reductions of surface metallic Ni and Be might weaken the adverse effect of Ni–Be eutectic phase on the surface distribution of Cr oxides. The optimization, leading to less segregation and more homogeneous of Cr surface oxides, was positive for the alloy's corrosion resistance. This result was supported by the corrosion data shown in Table 2, where ChangPing exhibited higher Ecorr and Ebr values after the pretreatment, and, though not statistical, Icorr value decreased and Rp value increased slightly.
In the present study, the pretreated Wirobond C (Co–Cr), Stellite N9 (Ni–Cr) and ChangPing (Ni–Cr–Be) alloys released less metal ions into the artificial saliva compared with the same alloys without the pretreatment (Fig. 4). These findings were in consistent with the XPS and corrosion results. After the pretreatment, the reduced surface levels of alloying elements, in particular for metallic Co, Ni, and Be, led to the decreases of the alloys' corrosion susceptibility, which, in turn, caused less dissolution of metal elements into the artificial saliva. Also, it was found that the tested alloys released a large amount of metal ions into the biological saline solution when underwent the pretreatment (Fig. 5). This was in accordance with Nelson et al.,10,11 who found high concentrations of Ag and Cu in the solution containing NaCl and BSA employed for preconditioning precious dental alloys. Nevertheless, in this study, little Cr ions dissolved in the biological saline solution and were still below the detection limit, indicating that the pretreatment almost had little influence on the tested alloys' surface Cr oxides. Together with the XPS analysis, this could confirm that the biological saline solution provoked the release of metal ions except Cr within the pretreatment process. Consequently, it is reasonable to assume that the pretreatment could be considered a useful method to remove ionizable metal elements, such as Co, Ni and Be, from surfaces of the three dental alloys.
As far as cellular responses were concerned, the pretreatment with a biological saline solution enhanced the cytocompatibility of Wirobond C (Co–Cr), Stellite N9 (Ni–Cr), and ChangPing (Ni–Cr–Be) alloys in this study. In the assessment of biomaterial cytocompatibility, cell adhesion and proliferation rate are two of the most studied biological parameters on in vitro experimental models with cell lines.32–34 Furthermore, it is well known that cell proliferation is strictly correlated to cell capability to adhere to the substrate; hence it seemed appropriate to investigate both two biological aspects in relationships to the presence of different dental alloys during performing the current study. We observed that the pretreatment influenced the biological behavior of fibroblast cultures on the alloys. Cells spread more evenly and extended more pseudopodia in the presence of the preconditioned alloys in comparison with those on the unconditioned alloys (Fig. 6). This evidence, together with the increased cell proliferation rates (Fig. 7), could confirm the effectiveness of the pretreatment for these base-metal dental alloys, in parallel with previous studies, which reported higher cell viability in the presence of noble-metal dental alloys preconditioned with protein-containing biological solutions.10–12 Cytotoxicity of dental alloys, especially base-metal alloys, had been obviously correlated with their metal ion release,35–37 which could induce adverse physiological effects in adjacent oral tissues, including gingival inflammation or discoloration. In our work, the biological saline solution encouraged the release of more labile metal elements in the pretreatment phase, which significantly decreased subsequent cytotoxicity of the three dental alloys. Thus, this observation of avoiding or limiting successive metal ion release and improving the cytocompatibility implied the possible utilization of the preconditioning treatment before clinical applications of these dental alloys.
Our investigation primarily assessed the effects of the pretreatment on the in vitro cytocompatibility of the dental alloys. However, the exact mechanism of the effectiveness and its biocompatibility evaluation still require further in vitro and in vivo studies.
5. Conclusions
On the basis of results obtained in the present study, it was found that the employ of the pretreatment with a biological saline solution for a Co–Cr and two Ni–Cr dental alloys could enhance their cytocompatibility, since more labile metal elements were removed from the alloy surfaces in form of cations, which optimized the surface elemental compositions and decreased the corrosion susceptibility. Thus, the positive effects of avoiding or limiting successive metal ion release and improving the alloys' cytocompatibility implied the possible utilization of the preconditioning treatment before clinical applications of these Co–Cr and Ni–Cr dental alloys.
Acknowledgements
This study was supported by the National Natural Science Foundation of China (Project number: 81472928), the Natural Science Foundation of Jiangsu Province (Project number: BK20130898), the Medical Science Foundation of Jiangsu Provincial Health Department (Project number: H201641), the “Six Talent Peaks” Project of Jiangsu Province (Project number: 2014-WSW-035), the Practice Innovation Training Program Project for the Jiangsu College Students (Project number: 201310312020Z), and the Foundation of Priority Academic Program Development of Jiangsu Higher Education Institutions (Project number: 2014-37).
References
- J. Palaskar, D. V. Nadgir and I. Shah, Contemp. Clin. Dent., 2010, 1, 237–242 CAS
. - L. Ristic, D. Vucevic, L. Radovic, S. Djordjevic, M. Nikacevic and M. Colic, J. Prosthodontics, 2014, 23, 221–226 CrossRef PubMed
. - A. S. Al-Hiyasat and H. Darmani, J. Prosthet. Dent., 2005, 93, 158–163 CrossRef CAS PubMed
. - P. Imirzalioglu, E. Alaaddinoglu, Z. Yilmaz, B. Oduncuoglu, B. Yilmaz and S. Rosenstiel, J. Prosthet. Dent., 2012, 107, 24–33 CrossRef CAS PubMed
. - J. Qiu, C. B. Tang, Z. J. Zhu, G. X. Zhou, J. Wang, Y. Yang and G. P. Wang, J. Mater. Sci.: Mater. Med., 2013, 24, 2519–2528 CrossRef CAS PubMed
. - J. Qiu, W. Q. Yu, F. Q. Zhang, R. J. Smales, Y. L. Zhang and C. H. Lu, Eur. J. Oral Sci., 2011, 119, 93–101 CrossRef CAS PubMed
. - J. Qiu, W. Q. Yu and F. Q. Zhang, J. Mater. Sci., 2011, 46, 1359–1368 CrossRef CAS
. - Y. Issa, P. Brunton, C. M. Waters and D. C. Watts, Dent. Mater., 2008, 24, 281–287 CrossRef CAS PubMed
. - G. Schmalz and P. Garhammer, Dent. Mater., 2002, 18, 396–406 CrossRef CAS PubMed
. - S. K. Nelson, J. C. Wataha and P. E. Lockwood, J. Prosthet. Dent., 1999, 81, 715–720 CrossRef CAS PubMed
. - S. K. Nelson, J. C. Wataha, A. M. Neme, R. M. Cibirka and P. E. Lockwood, J. Prosthet. Dent., 1999, 81, 591–596 CrossRef CAS PubMed
. - M. A. Sandrucci, V. Nicolin, L. Casagrande, M. Biasotto, L. Breschi and R. D. Lenarda, J. Mater. Sci., 2005, 40, 6233–6240 CrossRef CAS
. - D. M. Sarantopoulos, K. A. Beck, R. Holsen and D. W. Berzins, J. Prosthet. Dent., 2011, 105, 35–43 CrossRef CAS PubMed
. - M. C. Biesinger, B. P. Payne, A. P. Grosvenor, L. W. M. Lau, A. R. Gerson and R. S. C. Smart, Appl. Surf. Sci., 2011, 257, 2717–2730 CrossRef CAS
. - T. Fusayama, T. Katayori and S. Nomoto, J. Dent. Res., 1963, 42, 1183–1197 CrossRef CAS PubMed
. - T. Matkovic, P. Matkovic and J. Malina, J. Alloys Compd., 2004, 366, 293–297 CrossRef CAS
. - W. Zhang, Z. Li, Y. Liu, D. Ye, J. Li, L. Xu, B. Wei, X. Zhang, X. Liu and X. Jiang, Int. J. Nanomed., 2012, 7, 4459–4472 CAS
. - A. R. Ribeiro, F. Oliveira, L. C. Boldrini, P. E. Leite, P. Falagan-Lotsch, A. B. Linhares, W. F. Zambuzzi, B. Fragneaud, A. P. Campos, C. P. Gouvêa, B. S. Archanjo, C. A. Achete, E. Marcantonio Jr, L. A. Rocha and J. M. Granjeiro, Mater. Sci. Eng., C, 2015, 54, 196–206 CrossRef CAS PubMed
. - R. C. Reddy, V. R. Narala, V. G. Keshamouni, J. E. Milam, M. W. Newstead and T. J. Standiford, Blood, 2008, 112, 4250–4258 CrossRef CAS PubMed
. - Y. J. Bai, Y. B. Wang, Y. Cheng, F. Deng, Y. F. Zheng and S. C. Wei, Mater. Sci. Eng., C, 2011, 31, 702–711 CrossRef CAS
. - V. S. Saji and H.-C. Choe, Trans. Nonferrous Met. Soc. China, 2009, 19, 785–790 CrossRef CAS
. - R. W. W. Hsua, C. C. Yang, C. A. Huang and Y. S. Chen, Mater. Chem. Phys., 2005, 93, 531–538 CrossRef
. - G. J. Baran, J. Dent. Res., 1984, 63, 76–80 CrossRef CAS PubMed
. - A. W. E. Hodgson, S. Kurz, S. Virtanen, V. Fervel, C. O. A. Olsson and S. Mischler, Electrochim. Acta, 2004, 49, 2167–2178 CrossRef CAS
. - H. H. Huang, Biomaterials, 2003, 24, 1575–1582 CrossRef CAS PubMed
. - P. Lortrakul, R. W. Trice, K. P. Trumble and M. A. Dayananda, Corros. Sci., 2014, 80, 408–415 CrossRef CAS
. - X. Ren, K. Sridharan and T. R. Allen, Corrosion, 2007, 63, 603–612 CrossRef CAS
. - H. H. Huang, J. Biomed. Mater. Res., 2002, 60, 458–465 CrossRef CAS PubMed
. - J. Geis-Gerstorfer and K. Pässler, Dent. Mater., 1993, 9, 177–181 CrossRef CAS PubMed
. - J. J. Pan, Electrochem. Soc., 1995, 142, 1454–1458 CrossRef CAS
. - J. D. Bumgardner and L. C. Lucas, Dent. Mater., 1993, 9, 252–259 CrossRef CAS PubMed
. - N. M. Bettahalli, I. T. Arkesteijn, M. Wessling, A. A. Poot and D. Stamatialis, Acta Biomater., 2013, 9, 6928–6935 CrossRef CAS PubMed
. - M. Bigerelle and K. Anselme, J. Biomed. Mater. Res., Part A, 2005, 72, 36–46 CrossRef CAS PubMed
. - N. J. Hallab, K. J. Bundy, K. O'Connor, R. L. Moses and J. J. Jacobs, Tissue Eng., 2001, 7, 55–71 CrossRef CAS PubMed
. - A. Milheiro, K. Nozaki, C. J. Kleverlaan, J. Muris, H. Miura and A. J. Feilzer, Odontology, 2016, 104, 136–142 CrossRef CAS PubMed
. - M. C. Cortizo, M. F. De Mele and A. M. Cortizo, Biol. Trace Elem. Res., 2004, 100, 151–168 CrossRef CAS PubMed
. - W. Geurtsen, Crit. Rev. Oral Biol. Med., 2002, 13, 71–84 Search PubMed
.
Footnote |
† These authors contributed equally to this work. |
|
This journal is © The Royal Society of Chemistry 2017 |