DOI:
10.1039/C6RA25351B
(Paper)
RSC Adv., 2017,
7, 13019-13031
Dynamical regime of resorcinol based Belousov–Zhabotinsky chemical oscillator in the presence or absence of some hydrophobic antioxidants in aqueous–organic mixed media
Received
17th October 2016
, Accepted 21st February 2017
First published on 24th February 2017
Abstract
This study aims to overcome the difficulty of using hydrophobic moieties like antioxidants, hormones, etc. in oscillatory chemical reactions due to existing solubility problems in aqueous acid media. Several new perturbants viz. hydrophobic antioxidants have been found to affect the oscillatory parameters under batch conditions in the resorcinol based Belousov–Zhabotinsky (BZ) oscillating system performed in aqueous–organic mixed media. The α-tocopherol, 4,4,4-trifluoro-3-methyl-2-butenoic acid, melatonin and β-carotene react with radicals either generated from BZ reagents or from the organic solvents and scavenge them. One to one interactions of these additives with resorcinol, bromate or Mn(II) ions have been investigated using cyclic voltammetry and potentiometry. These interactions may lead to a better understanding of their mechanism corresponding to their role in the BZ chemical oscillators. A comparative trend of these antioxidants was observed. It is noteworthy to mention here that radical based polymerization has been achieved using a specific solvent system containing monomers of that polymer and an interesting behavior oscillatory parameters was observed during polymer synthesis in situ.
Introduction
Oscillating reactions are complex dynamic systems that involve cyclic or periodic changes in the concentration of some ingredients (whether a reactant, a product or an intermediate) with time.1 This type of system has been known since 1910, when the first model for an oscillating chemical system was developed.2,3 Oscillating reactions have so far been analyzed mainly in physico-chemical terms in order to elucidate the complex mechanism involved. Two of the better known oscillating chemical systems are the Belousov–Zhabotinsky (BZ) reaction4–10 and the Bray–Liebhafsky reaction.11–13,15 From the available knowledge on these reactions, it follows that the system must be far from thermodynamic equilibrium i.e., ΔG must be large and negative, which entails using a well stirred continuous-flow reactor (CSTR),14,16–18 and that one or more autocatalytic or cross-catalytic steps must take place between two steps of the reaction mechanism.19 Although some oscillating reactions have been used for analytical purposes, particularly the Ce4+-catalyzed reaction between malonic acid and KBrO3, known as BZ reaction,20,21 analytical determinations based on a closed system including the analyte involve labour-intensive procedures that entail re-starting the oscillating system before each new determination. This accounts for the little interest aroused so far by this type of reaction for analytical purposes. The recent inception of an open system for application of the analyte pulse perturbation (APP) technique by use of a CSTR22–28 has opened up new avenues for oscillating reactions in routine analyses. As a result, there has been a gradual shift from theoretical to practical interest.
Several subjects were determined by the APP technique, such as riboflavin,29 gallic acid, resorcinol,26 ascorbic acid,14,30 glutamic acid,31 glutathione,24 paracetamol,14,32 1-naphthylamine,33 thallium,34 morphine,35 vanillin,14 alpha-naphthol36 and Alizarin Red S using tetraazamacrocyclic complex of copper,37 but all these studies took place in aqueous acid media only.
The catalytic redox couples involved in these BZ reaction focused on Ce3+/Ce4+, Mn2+/Mn3+, Fe(phen)32+/Fe(phen)33+ and Ru(bpy)32+/Ru(bpy)33+. Recently, macrocyclic complexes as catalysts were widely investigated. The first publication was of a catalytic macrocyclic complex of Cu(II) and Ni(II) reported by Yatsimirskii in 1982.38 Lately, BZ reactions catalyzed by these complexes have attracted much more attention because of their unusual oscillating features, which are reflected in lower activation energies, higher oscillating frequencies39 and being vulnerable to external perturbations. By using macrocyclic Cu(II) or Ni(II) complexes as catalyst, the oscillating reactions involving lactic acid,40,41 malonic acid,42 and pyruvic acid43 as organic substrate species have been studied. Recently, a new BZ oscillating system with a tetraazamacrocyclic copper(II) complex [CuL] (ClO4)2 as catalyst and malic acid substrate44 have been reported. The ligand, L, in the complex is 5,7,7,12,14,14-hexamethyl-1,4,8,11-tetraazacyclotetradeca-4,11-diene. Based on this new BZ oscillating system, Ag+ (ref. 39) and pyrogallol45 were determined by perturbations of analyte on the oscillating system.
However, all these APP determinations involve substrates which were soluble in aqueous acid media and thus restricted the use of hydrophobic species like vitamins, antioxidants or hormones as perturbants in such systems. During this study, we have worked on several organic–aqueous mixed media to evaluate the best one that can be used for deterministic studies in future. Here, four hydrophobic biologically important antioxidants have been used as perturbants, solubilized by these non aqueous mixed media under unstirred batch conditions, as was the case with resorcinol as substrate in our previous works46–48 and this has opened a new chapter to carry out the investigations with similar other hydrophobic species in future. The resorcinol has been chosen owing to its sufficient solubility in aqueous acid medium and dynamic behavior over a wide range of concentrations.47 Moreover, the presence of two phenolic OH groups in resorcinol exhibits some similarity with several biomolecules and, therefore, the used of this substrate served in two ways; (i) it can serve as a prototype example in understanding the behaviors possible in biology such as signal transmission and membrane transport in living organisms and (ii) it can help in further establishing the mechanism of aromatic substrates in these complex oscillatory chemical reactions.
The main objective of this work was to solubilize the hydrophobic antioxidants like α-tocopherol, 2,2,2-trifluoro-3-methyl-2-butenoic acid (TFMBA), melatonin {N-acetyl-5-methoxytryptamine or N-[2-(5-methoxy-1H-indol-3-yl)ethyl] acetamide} and β-carotene, which generally act as radical scavengers in living systems, in acetonitrile (ACN) and acrylonitrile/dimethylformamide (AN/DMF) based aqueous organic mixed media. Although various other non-aqueous solvents have been attempted for the study such as THF, dioxane, DMF alone, n-hexane and amyl alcohol but all of them undergo either extensive bromination or didn't yield the expressive nonlinear behavior, thus limiting their use as feasible solvents in BZ reaction. The AN/DMF based mixed media, whose ratio has been specifically reached at by hit and trial methods, is of special interest because of observation of in situ radical polymerization and the use of antioxidants in this solvent system caused delay of polymerization reaction due to radical quenching phenomenon (Scheme 1).
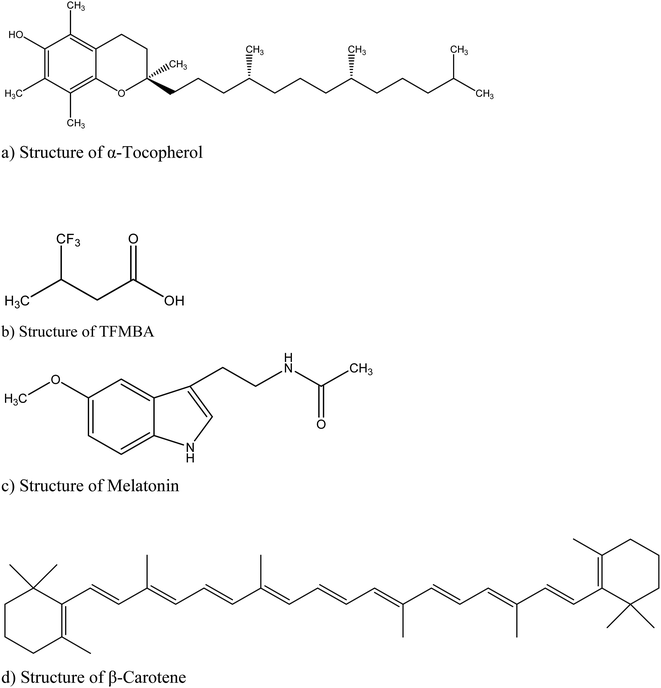 |
| Scheme 1 | |
Experimental
Materials
The reagents used were resorcinol (Aldrich), potassium bromate (Merck), manganese(II) sulfate monohydrate (Aldrich), sulfuric acid (Merck), dimethyl formamide (DMF) (Merck), acetonitrile (ACN) (Merck), acrylonitrile (AN) (Aldrich), α-tocopherol (Sigma); 4,4,4-trifluoro-3-methyl-2-butenoic acid (Aldrich), melatonin (Aldrich), β-carotene (Aldrich). All the reagents used were analytical grade chemicals with high degree of purity and were used without any further purification. The solutions of the desired chemicals were prepared in respective percentages of organic solvents (5% v/v for acetonitrile and 5% v/v AN: 4.4% v/v DMF for other solvent system) in 1.4 mol L−1 sulfuric acid using double distilled water. In this study the initial concentrations of BZ reagents like resorcinol, bromate and MnSO4 solutions are 0.0225 mol L−1, 0.1 mol L−1 and 0.005 mol L−1 respectively (for all those reaction systems where concentrations are not mentioned), which are the optimal concentrations for producing typical time dependant oscillatory regime, i.e. with optimal oscillatory parameters like amplitude, number of oscillations, frequency, etc., which is a pre-requisite for perturbation studies. The oscillations are observed by adding bromate solution to the mixture of resorcinol and Mn2+ ion solution.
Procedure
Simple potentiometric studies have been performed. The reaction system for consists of two reaction cells, connected through salt bridge (potassium nitrate in agar agar). One cell contains platinum electrode in which bromate, resorcinol and Mn(II) sulfate solutions are mixed and the other contains calomel electrode as reference in which 2.5 × 10−4 mol L−1 solution of potassium chloride is kept. Both these electrodes are then hooked to Orion 4 star, pH ISE Ion Analyzer (Cole Parmer (India) Pvt. Ltd.) in order to monitor change in potential (mV) with time (s) connected to PC via RS232 cable and operated through 21CFR11 software. The desired thermostatic conditions of the reaction system under investigation were maintained using Advantec water bath (TBS451PA, Cole Parmer (India) Pvt. Ltd.) with a precision of ±0.1 °C. The advanced cyclic voltammetry (CV) was performed with a potentiostat (SP-150, Biologic France. SAS), using three micro electrodes viz. platinum wire and Glassy Carbon Electrode (GCE, ALS QI058, GC6 × 1.6) as counter and working electrodes respectively and saturated calomel as reference electrode (SCE, ALS 002056, RE-2B, Japan) in a voltammetry cell (ALS 012669, SVC-3), connected to a PC. All the CV experiments were performed at room temperature (25 ± 0.5 °C).
The percentage compositions used for two organic media were: 5% v/v for acetonitrile and 5.6% v/v acrylonitrile: 4.4% v/v DMF (mixed), both in 1.4 mol L−1 of H2SO4. The solutions of all the reagents as well as antioxidants of desired concentrations were prepared in aqueous–organic mixed media. Except bromate solution, all the BZ reagent solutions including that of antioxidants of suitable concentrations were prepared in organic–aqueous acid mixed media, to avoid the direct early reaction of the former, as bromate is responsible for bromination of the organic solvent as well. As reported in our previous papers46,47 also, for potentiometric studies, the volumes of initial reagents which are mixed in a proper sequence (resorcinol and metal first followed by bromate) are 2 mL each, wherein, bromate solution is always added last out of the BZ reagents.
Results and discussion
Due to the non feasibility of using all the organic solvents as mentioned above, we have chosen only two for their better oscillatory parameters. Acetonitrile based system is having prominent amplitudes for the main BZ system and bromination of organic substrate in presence of acetonitrile is favored49 and there may be lesser bromination of antioxidants in presence of this solvent mixture, because the solvent occupies all the sites where bromination can take place. Hence the effect observed is mostly due to quenching of radicals generated in situ by the antioxidants. It is to be noted here that owing to the different chemical structure of antioxidants which may act as bromination centers upon one to one interaction of bromine and these antioxidants, we have also tried for using them as main substrates in the BZ reaction, but none of them showed any oscillatory regime. However, in all these systems the potential first showed continuous increase and then decreases. This can be due to the quenching of radicals generated in situ by these antioxidants and antioxidants don't take part in autocatalytic reaction steps as per FKN50 and OKN51 mechanisms. The second solvent system chosen was AN/DMF based system, which was specifically chosen in order to confirm the radical generation in situ as well as role of BZ reagents and intermediates in the polymer matrix. This study may help in understanding radical control because of generation of polymers in situ.
Acetonitrile based mixed media
Fig. 1 gives us the typical oscillatory profile of the resorcinol based BZ system in acetonitrile based mixed medium. Large amplitude oscillations was observed for 0.16 mol L−1 [BrO3−] with decrease in life time than the already known oscillations from aqueous acid medium,47 hence, used as typical oscillatory profile for this medium.
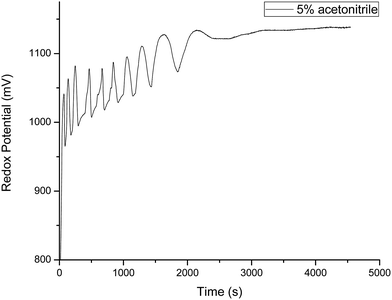 |
| Fig. 1 Typical oscillatory profile of the system containing [resorcinol]0 = 0.0225 mol L−1, [BrO3−]0 = 0.16 mol L−1, [Mn2+]0 = 0.005 mol L−1 in 5% v/v acetonitrile prepared in 1.4 mol L−1 of [H2SO4]0 at 30 °C. BrO3− solution is prepared in only aqueous acid and the other reagents in aqueous–organic mixed media. 2 mL of each of the reagents are mixed in the reaction vessel (25 mm × 100 mm) with resorcinol and Mn2+ solutions first followed by bromate solution. | |
Antioxidants in acetonitrile based media
Addition of antioxidants to the above mentioned system normally quenches the oscillations, but the extent of quenching under given conditions varies with the nature of the hydrophobic antioxidant used. The quenching for these antioxidants under given conditions is shown by the following trend:
α-tocopherol < TFMBA < melatonin < β-carotene |
Quite contrary to the effect of antioxidants, 0.005 mol L−1 concentration of α-tocopherol had shown increased number of oscillations after a gap of 2000 s, which is due to its complete consumption in situ and due to the formation of para-quinonoid species, which decreases its radical quenching behavior. Bohmdorfer, et al. found that in case of α-tocopherol, halogenating effect is minor and the major pathway is the oxidative action resulting in the para-quinone.52 Hence, the bromination is mainly observed by the resorcinol instead of α-tocopherol. Further, it is observed that increasing the concentration of TFMBA up to 0.005 mol L−1 and for β-carotene up to 0.0005 mol L−1 completely quenches the oscillations. TFMBA is having a C
C in the molecule, but owing to its proximity with most electronegative three fluorine atoms it does not favor the attack of bromonium ion for bromination. Therefore, bromine generated in situ will be mostly used by in FKN mechanism. Fig. 2 gives a comparative data of different antioxidants as perturbants/radical scavengers. Out of these β-carotene has been found to be strong quencher followed by melatonin for oscillations, whereas α-tocopherol shows oscillations for a longer life time followed by TFMBA which also show some oscillations under given conditions. There is marked increase in number and amplitude of oscillations in case of α-tocopherol, which may be due to its complete consumption in quenching radicals and reducing metal ion in the autocatalytic process of oscillatory reactions.47 When whole of the antioxidant is used up, the intermediates regenerate and oscillations with larger time periods re-emerge. But it was observed that increasing the concentration from 0.005 to 0.01 mol L−1 for α-tocopherol and up to 0.001 mol L−1 for melatonin, completely quenched the oscillations under given set of conditions. Further, the addition of melatonin and β-carotene initially decreased the overall potential of the system, which is due to the reactions of oxybromine species [Br−/HOBr, HBrO2/Br2 couples] generated in situ with the antioxidants and increased generation of [Mn3+] ion with these, thereby decreasing the [Mn2+]/[Mn3+] couple.3 Melatonin is used due to its role in controlling circadian rhythms and various other biological activities such as inhibition of Alzheimer β-fibrillogenesis, anti-aging properties relating to radical scavenging activities, etc. and β-carotene is the dietary source of vitamin A and has antioxidant properties that can help neutralize free radicals like reactive oxygen molecules potentially damaging lipids in cell membranes and genetic material, which may lead to the development of cardiovascular disease and cancer.
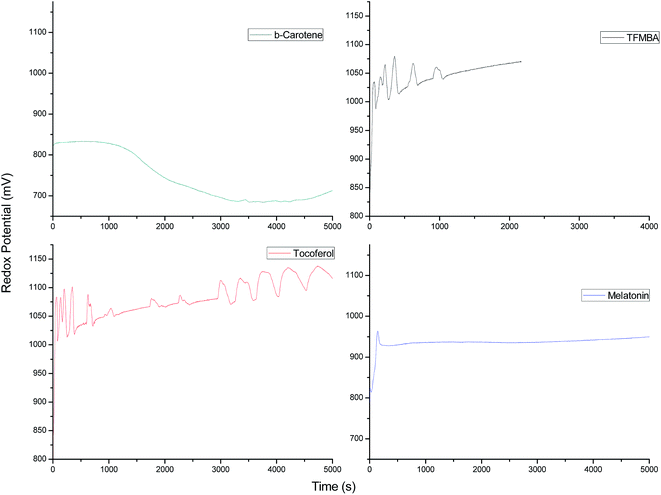 |
| Fig. 2 Potential (mV) versus time (s) plots of the oscillations of resorcinol–BrO3−–Mn2+–H2SO4 system in 5% v/v acetonitrile organic–aqueous mixed media after perturbation by 0.5 mL of different antioxidants, added just after the addition of BrO3− solution. The added concentration for β-carotene and α-tocopherol is 0.005 mol L−1 and for 4,4,4-trifluoro-3-methyl-2-butenoic acid (TFBMA) and melatonin = 0.0025 mol L−1. The concentration of BZ mixture is given in Fig. 1. | |
The mechanism of resorcinol based Mn2+ catalyzed BZ system can be understood by the following steps (1) to (12). Here, HAr(OH)2 is an aromatic compound (like resorcinol) containing at least two phenolic groups, HAr(OH)O˙ is the radical obtained by hydrogen atom abstraction, HArO2 is the related quinone, BrAr(OH)2 is the brominated derivative, and Ar2(OH)4 is the coupling product.
|
BrO3− + Br− + 2H+ ↔ HBrO2 + HOBr
| (1) |
|
HBrO2 + Br− + H+ → 2HOBr
| (2) |
|
BrO3− + HBrO2 + H+ ↔ 2BrO2 + H2O
| (3) |
|
BrO2˙ + HAr(OH)2 → HBrO2 + HAr(OH)O˙
| (4) |
|
2HBrO2 → BrO3− + HOBr + H+
| (5) |
|
HOBr + HAr(OH)O˙ ↔ Br˙ + HArO2 + H2O
| (6) |
|
Br˙ + HAr(OH)O˙ → Br− + HArO2 + H+
| (7) |
|
HOBr + Br− + H+ ↔ Br2 + H2O
| (8) |
|
Br2 + HAr(OH)2 → BrAr(OH)2 + Br− + H+
| (9) |
|
HOBr + HAr(OH)2 → BrAr(OH)2 + H2O
| (10) |
|
2(BrO3− + HBrO2 + 2MRED + 3H+ ↔ 2HBrO2 + 2MOX + H2O)
| (11) |
|
BrO3− + 4MRED + HAr(OH)2 + 5H+ → BrAr(OH)2 + 4MOX + 3H2O
| (12) |
Fig. 3 showed the interactions of β-carotene with resorcinol and BrO3− after different time intervals. The cyclic voltammograms for only resorcinol in acetonitrile based mixed medium showed two anodic peaks (3.75 μA, 6.33 μA), which are due to oxidation of both the hydroxyl groups on resorcinol. With the addition of BrO3−, there is increase in oxidation peak of one corresponding to main anodic peak of resorcinol based system (4.31 μA) and a sharp decrease in the other oxidation peak (4.34 μA) or in other words the other peak of other OH disappears. After certain intervals of time, there is continued decrease in oxidation of the 1st peak corresponding to [resorcinol], which decreased further with the addition of β-carotene (Fig. 3c and d). This is due to the partial enhanced stability of the oxidized substrate in this polar medium, which gets decreased by the subsequent bromination of the mixed organic polar medium i.e. acetonitrile, thereby making it less polar and hence favoring oxidation of only one hydroxyl group. With the addition of β-carotene and after certain intervals of time, there is usual decrease in oxidation of substrate with small generation of oxybromine species because of radical quenching activity thereby, decreasing the oxidation peak further.
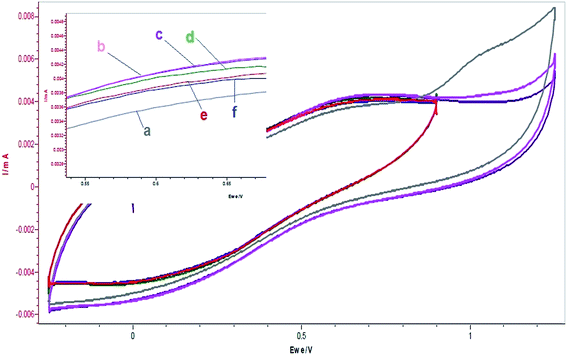 |
| Fig. 3 Cyclic voltammograms of the reactions of resorcinol (0.0225 mol L−1) + [BrO3−]0 (=0.1 mol L−1) at different times and different volume additions of [β-carotene]0 = 0.005 mol L−1: (a) resorcinol = 5 mL, (b) resorcinol + 3 mL BrO3− (c) resorcinol + 3 mL BrO3− after 3 min, (d) resorcinol + 3 mL BrO3− + 2 mL β-carotene, (e) resorcinol + 3 mL BrO3− + 2 mL β-carotene after 3 min, (f) resorcinol + 3 mL BrO3− + 4 mL β-carotene. (Resorcinol and β-carotene solutions are prepared in 5% v/v acetonitrile in [H2SO4]0 = 1.4 mol L−1 and BrO3− in 1.4 mol L−1 [H2SO4].) Scan rate = 200 mV s−1. | |
Fig. 4 depicted the CV for the interactions of β-carotene with Mn2+ at different times and volume additions of the β-carotene and resorcinol. It is observed that rate of oxidation increases with time after addition of β-carotene, whereas addition of resorcinol to this mixture produced marginal increase in oxidation peak current. This is due to the reduction of Mn3+ by the β-carotene initially, but after some time when whole of the [antioxidant] is consumed. Oxidation peak increases due to formation of Mn3+ species, which have feeble interactions with the resorcinol and the organic solvent as compared to Mn2+ ion.48
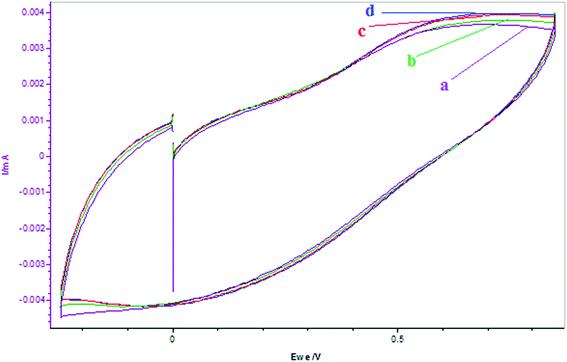 |
| Fig. 4 Cyclic voltammograms of the reactions of MnSO4 (0.005 mol L−1) + [β-carotene]0 = 0.005 mol L−1 at different times and addition of [resorcinol]0 = 0.0225 mol L−1: (a) MnSO4 = 5 mL, (b) MnSO4 + 2 mL β-carotene (c) MnSO4 + 2 mL β-carotene after 5 min, (d) MnSO4 + 2 mL β-carotene + 3 mL resorcinol. MnSO4, β-carotene and resorcinol solutions are prepared in 5% v/v acetonitrile in [H2SO4]0 = 1.4 mol L−1. Scan rate = 200 mV s−1. | |
Interaction of antioxidants in acrylonitrile/DMF based aqueous acid mixed media
As from our observations regarding the confirmation of polymer species generated in situ for the AN/DMF based mixed medium, it was a good initiative to introduce the antioxidants to this system. The antioxidants are having strong radical scavenging capacity and thus could affect the rate of radical polymerization vis-à-vis quenching of the radical intermediates generated for BZ system. For this study, all the four antioxidants were used, whereas the thorough studies were carried out for melatonin and α-tocopherol with other BZ reagents. Fig. 5 depicts the typical oscillatory profile of resorcinol based system in AN/DMF based mixed medium. For this system, the induction period is 130 s followed by average time period of about 60 s (region I), which showed a continuous increase with time. The amplitude decreased steadily up to 1790 s followed by an increase with increase in time period as well (region II). The peculiarity of this system is that it showed prolonged minimal amplitude oscillations (5 mV) at later stages of the reaction (region III).
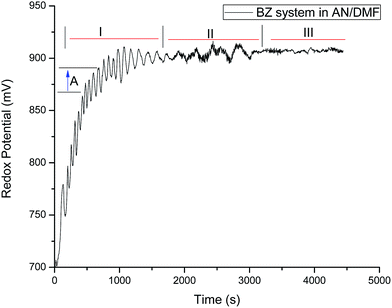 |
| Fig. 5 Typical oscillatory profile of the system containing 2 mL each of [resorcinol]0 = 0.0225 mol L−1, [BrO3−]0 = 0.1 mol L−1, [Mn2+]0 = 0.005 mol L−1 in 5% v/v AN: 4.4% v/v DMF prepared in 1.4 mol L−1 of [H2SO4]0 at 30 °C. BrO3− is prepared in aqueous acid medium and the other reagents in aqueous–organic mixed media. | |
Melatonin in acrylonitrile/DMF
The effect of additions of different concentrations of melatonin on the oscillatory behavior of resorcinol based system in this media is depicted from potential–time plots shown in Fig. 6. It is observed that with increase in concentration of melatonin at the start of reaction, there is decrease in number as well as amplitude of large amplitude oscillations. However, there is increase in time period of small amplitude oscillations. This system showed strong quenching for concentrations ≥0.01 mol L−1 of melatonin. This can be due to the radical scavenging activity of melatonin, as was observed from the decreased rate of formation of polymer (yellowish due to bromoresorcinol, actually white precipitate) as depicted in Fig. 7. The polymer material was confirmed using IR and have been synthesized in situ. These polymers were found to be Nearly Ultra High Molecular Weight (NUHMW) polymers with molecular weights >350
000 daltons.53 Here the yellowish color is due to bromination of the resorcinol, which can be easily separated using any organic solvent other than DMF and DMSO. The polymer has been found to be soluble in only these two solvents. In our recently published work, similar polymer was synthesized and characterized using several spectroscopic techniques and the synthesis have been performed using visible light as well for generation of radicals. The polymer isolated and separated from BZ mixture showed similar characteristics. Further, in AN/DMF based system, minimal amplitude oscillations were observed for longer time duration and in case of Fig. 6D minimal amplitude and larger time period oscillations are observed even after t = 3000 s. This may be due to diffusion control and extended release of some of the BZ intermediates responsible for oscillations, which are entrapped in hydrophobic environment of the polymer earlier. This behavior may be correlated with the sustained release and diffusion control of recent drugs, wherein drug release through matrix system or diffusion of drugs from reservoir coated with suitable polymers, helps the patients for longer periods of time.54,55 Similarly, the BZ intermediates and reagents also trap in the polymer matrix from where they are released in smaller concentrations, leading to small amplitude but longer time period oscillations. Further, now due to the inhomogeneity caused by the solid polymer inside the reaction mixture, appearance of some noise is also observed throughout the oscillatory regime. It is noteworthy to mention here that the resorcinol based system showed optimal oscillatory parameters only under unstirred conditions and stirring doesn't yield significant oscillatory parameters for such a study. It is to be noted that due to the bromination of the solvent,56 we used higher concentrations of bromate solutions for organic–aqueous mixed media as compared to that of aqueous acid media in our previous studies for resorcinol based BZ system. This bromination effect can be seen from continuous decrease in amplitude of oscillation (region I in Fig. 5). Further, it is observed that none of the BZ systems showed any oscillatory regime using antioxidants alone as substrates without resorcinol. Hence, it was concluded that primarily all the antioxidants may be responsible for quenching of radicals in resorcinol based chemical oscillator depending upon their structure and functionalities rather than acting as co-substrates.
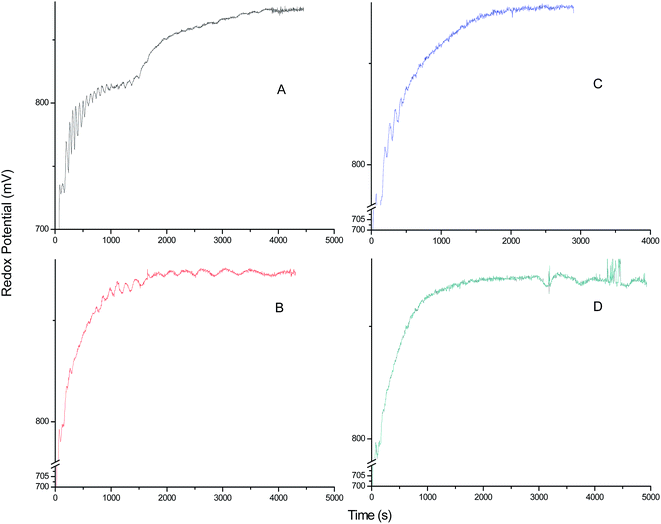 |
| Fig. 6 Potential (mV) versus time (s) plots of the oscillations of resorcinol–BrO3−–Mn2+–H2SO4 BZ system in 5.6% AN: 4.4% DMF in 1.4 mol L−1 H2SO4 organic–aqueous acid mixed media, after perturbation by 0.5 mL of different concentrations of melatonin, added just after the addition of BrO3− solution. The added concentration for melatonin: (A) 0.004 mol L−1, (B) 0.006 mol L−1, (C) 0.008 mol L−1, (D) 0.01 mol L−1. The concentrations and volumes of BZ reagents are given in Fig. 5. | |
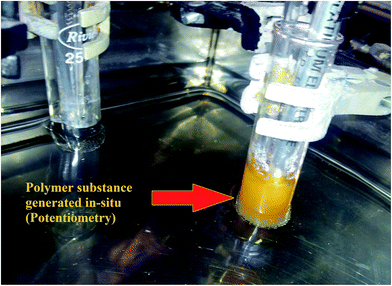 |
| Fig. 7 Polymeric substance generated in situ for the system containing [resorcinol]0 = 0.0225 mol L−1, [BrO3−]0 = 0.1 mol L−1, [Mn2+]0 = 0.005 mol L−1 in 5% v/v AN: 4.4% v/v DMF prepared in 1.4 mol L−1 [H2SO4]0 at 30 °C. | |
α-Tocoferol in acrylonitrile/DMF
Fig. 8 showed the effect of [α-tocopherol] on the oscillatory behavior of the BZ system under investigation using potentiometry. As compared to melatonin, it showed strong quenching for concentrations ≥0.001 of α-tocopherol, with a similar appearance of minimal amplitude prolonged oscillations. The change in oscillatory parameters observed for increased [α-tocopherol] are similar as that of melatonin. This may be due to the fact that with the addition of α-tocopherol, there is decrease in oxidation peak of metal ion as shown in Fig. 9. The CV plots depicted that there is continuous decrease in oxidation peak for Mn2+ ion with time till whole of the antioxidant get consumed, after which there is again a steady increase (Fig. 9c and d).
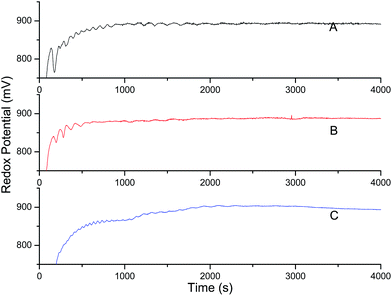 |
| Fig. 8 Potential (mV) versus time (s) plots of resorcinol–BrO3−–Mn2+–H2SO4 BZ system in 5.6% AN: 4.4% DMF of 1.4 mol L−1 H2SO4 organic aqueous acid mixed media after perturbation by 0.5 mL of different concentrations of α-tocopherol, added just after the addition of BrO3− solution. The added concentration for α-tocopherol: (A) 0.0002 mol L−1, (B) 0.0004 mol L−1, (C) 0.001 mol L−1. The concentration and volumes of BZ reagents are given in Fig. 5. | |
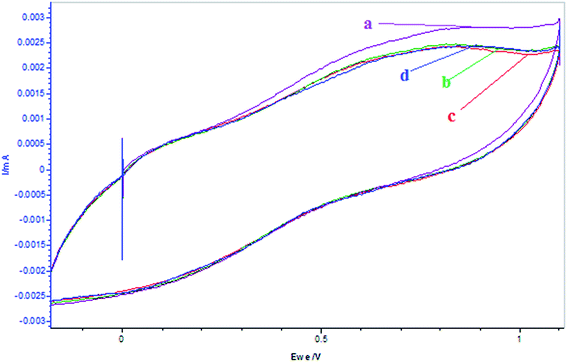 |
| Fig. 9 Cyclic voltammograms of the reactions of MnSO4 (0.005 mol L−1) + [α-tocopherol]0 = 0.001 mol L−1 at different times after addition of α-tocopherol: (a) MnSO4 only = 5 mL, (b) 0 min (c) 3 min, (d) 15 min. (MnSO4, and α-tocopherol solutions are prepared in 5.6% AN: 4.4% DMF in 1.4 mol L−1 H2SO4 aqueous acid mixed media. Scan rate = 100 mV s−1.) | |
Further, it is observed from the CV plots shown in Fig. 10 that the addition of α-tocopherol to resorcinol decreased its oxidation peak, which may be attributed to its reduction by the antioxidant, which is reversed by the addition of BrO3− due to higher reduction potential of the later. However, there is much interaction between α-tocopherol and Mn2+ ion observed in this media as compared to resorcinol. This can be seen from CV plots shown in Fig. 11, wherein there is larger decrease in oxidation peak for Mn2+ with the addition of α-tocopherol. But, this decrease continues with the addition of BrO3−, quite opposite to the case of resorcinol. This can be attributed to the increased rate of reduction of Mn3+ by this concentration of α-tocopherol, and the addition of 0.2 mmol L −1 of BrO3− could not oxidize it further. This can be confirmed by the CV plots shown in Fig. 12 for the interaction of BrO3− with α-tocopherol.
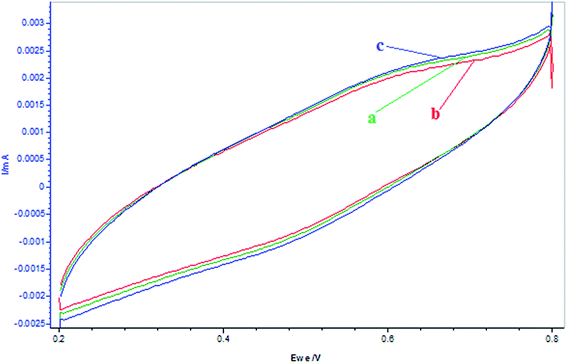 |
| Fig. 10 Cyclic voltammograms of the reactions pertaining to resorcinol (0.0225 mol L−1) + [α-tocopherol]0 = 0.003 mol L−1 at different times and addition of [BrO3−]0 = 0.1 mol L−1: (a) resorcinol = 5 mL, (b) resorcinol + α-tocopherol after 2 min (c) resorcinol + α-tocopherol + BrO3−. (α-Tocopherol and resorcinol solutions are prepared in 5.6% AN: 4.4% DMF in 1.4 mol L−1 H2SO4 and BrO3− is prepared in 1.4 mol L−1 H2SO4 only.) Scan rate = 200 mV s−1. | |
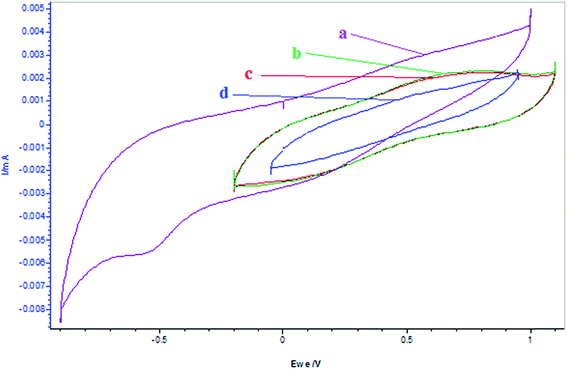 |
| Fig. 11 Cyclic voltammograms of the reactions of MnSO4 (0.005 mol L−1) + α-tocopherol at different times and additions of [BrO3−] = 0.1 mol L−1 and α-tocopherol: (a) MnSO4 = 5 mL, (b) MnSO4 + α-tocopherol = 0.001 mol L−1, (c) MnSO4 + α-tocopherol = 0.002 mol L−1 after 25 min, (d) MnSO4 + α-tocopherol = 0.002 mol L−1 + 2 mL BrO3−. (α-Tocopherol and MnSO4 solutions are prepared in 5.6% AN: 4.4% DMF in 1.4 mol L−1 H2SO4 and BrO3− is prepared in aqueous acid medium.) Scan rate = 100 mV s−1. | |
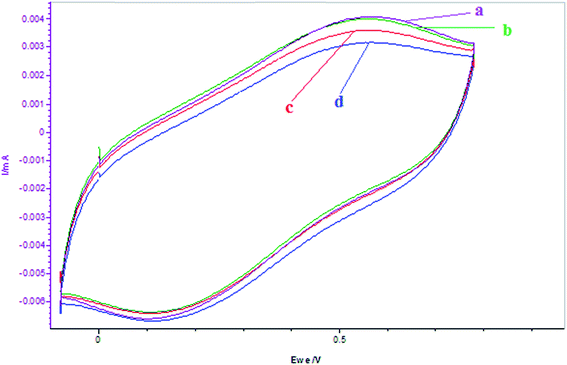 |
| Fig. 12 Cyclic voltammograms of the reactions of BrO3− + α-tocoferol after 10 minutes of additions of α-tocoferol: (a) BrO3− = 5 mL, (b) BrO3− + α-tocoferol = 0.001 mol L−1, (c) BrO3− + α-tocoferol = 0.002 mol L−1, (d) BrO3− + α-tocoferol = 0.003 mol L−1. (α-Tocoferol is prepared in 5.6% AN: 4.4% DMF in 1.4 mol L−1 H2SO4 and BrO3− is prepared in aqueous acid medium.) Scan rate = 200 mV s−1. | |
With increase in [α-tocopherol]0 and time there is continuous decrease in reduction peak potential of BrO3− because of two reasons: one is due to extensive bromination of organic solvent, hence decreasing its concentration and the second is due to its reduction with the α-tocopherol added.
TFMBA and β-carotene in acrylonitrile/DMF
Fig. 13 depicted the expression of oscillatory behavior with the addition of TFMBA and β-carotene at the start of reaction. The potential–time plots clearly show that there is marked quenching of oscillations for both these systems, whereas the quenching is larger for TFMBA than β-carotene. Both these antioxidants were added to the resorcinol based system with increased [BrO3−]0 = 0.16 mol L−1, as there is extensive bromination of the organic solvents and 0.1 mol L−1 concentration didn't yield any oscillations after addition of antioxidants. For these systems low amplitude oscillations were observed due to quenching of radical species and also due to removal of Br2 by its involvement in bromination of organic solvent. Fig. 14 clearly depicted that addition of β-carotene to the BrO3− in such media, marginal increase in its anodic peak, whereas there is larger decrease in the cathodic peak with time. The decrease in cathodic peak can be due to bromination of the solvent, hence decreasing its concentration, whereas increase in anodic peak could be due to promotion of oxidation effect of the β-carotene in this solvent.
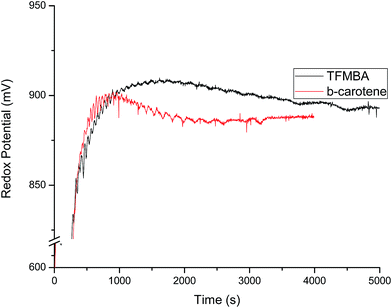 |
| Fig. 13 Potential (mV) versus time (s) plots of resorcinol–BrO3−–Mn2+–H2SO4 BZ system in 5.6% AN: 4.4% DMF in 1.4 mol L−1 H2SO4 aqueous acid mixed media after perturbation by different antioxidants just before the addition of BrO3− solution. TFMBA = 0.005 mol L−1 (Black), β-carotene = 0.00025 mol L−1 (Red). The concentration of BZ matrix is [resorcinol]0 = 0.0225 mol L−1, [BrO3−]0 = 0.16 mol L−1, [Mn2+]0 = 0.005 mol L−1. | |
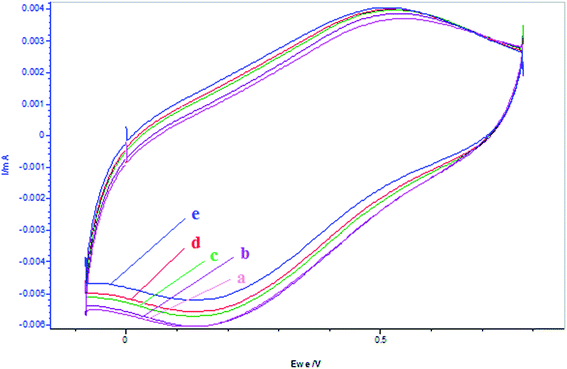 |
| Fig. 14 Cyclic voltammograms of the reactions of BrO3− (0.16 mol L−1) + β-carotene after different times of additions of β-carotene: (a) BrO3− = 5 mL, (b) BrO3− + β-carotene = 0.001 mol L−1 after 1 min, (c) BrO3− + β-carotene = 0.002 mol L−1 after 10 min, (d) BrO3− + β-carotene = 0.003 mol L−1 after 10 min, (e) BrO3− + β-carotene = 0.003 mol L−1 after 10 min. (β-Carotene is prepared in 5.6% AN: 4.4% DMF in 1.4 mol L−1 H2SO4 and BrO3− is prepared in aqueous acid medium.) Scan rate = 200 mV s−1. | |
Conclusions
The use of mixed media in solubilizing the water insoluble antioxidants has been carried out for the first time in oscillatory chemical reactions. A comparative study pertaining to radical scavenging effect of different water insoluble antioxidants in two different organic–aqueous mixed media has been performed. In AN/DMF based organic–aqueous mixed medium, different amounts of inhibition of the radical polymerization took place depending upon the generation of BZ intermediates or the initial reagent concentrations. The oscillatory parameters vary significantly due to in situ polymerization and the results showed extended oscillations than the main resorcinol based BZ system. This study can be performed with a variety of biologically important hydrophobic additives in order to understand their mechanism of action with suitable reagents, thereby, can serve as prototype example for understanding the mechanisms of similar type of reactions in vivo.
Acknowledgements
The authors are thankful to Department of Science and Technology, Government of India for providing equipment grant in the form of FIST level-1 to the department. The authors also thank Head, Department of Chemistry University of Kashmir for facilitating us to undertake the work in the department.
References
- R. J. Field and F. Schneider, Oscillating chemical reactions and nonlinear dynamics, J. Chem. Educ., 1989, 66, 195–204 CrossRef CAS.
- A. Lotka, Contribution to the theory of periodic reactions, J. Phys. Chem., 1910, 14, 271–274 CrossRef CAS.
- R. M. Noyes, Some models of chemical oscillators, J. Chem. Educ., 1989, 66, 190–191 CrossRef CAS.
- A. Karavayev and V. Kazakov, Chemiluminescence and free-radical stages of the Belousov–Zhabotinskii reaction, React. Kinet. Catal. Lett., 1987, 34, 15–20 CrossRef.
- H. Saigusa, Chemiluminescence detection of a phase response to an oxygen perturbation in the Ru(bpy)32+ catalyzed Belouzov–Zhabotinskii reaction, Chem. Phys. Lett., 1989, 157, 251–256 CrossRef CAS.
- V. P. Kazakov, A. D. Karavayev and S. R. Vakhidova, Some peculiarities of chemiluminescence (CL) in Belousov–Zhabotinskii reactions. I. Unusual shape of CL oscillations, React. Kinet. Catal. Lett., 1991, 45, 199–205 CrossRef CAS.
- H. R. Weigt, Chemiluminescence Oscillations Driven by a Flow-Through Reactor in the [Ru(bpy)3]2+ Catalyzed Belousov–Zhabotinskii Reaction (pages 355–357), Angew. Chem., Int. Ed. Engl., 1992, 31, 355–357 CrossRef.
- A. I. Zhuravlev and V. M. Trainin, Chemiluminescent reactions in the Belousov–Zhabotinskii oscillating system, J. Biolumin. Chemilumin., 1990, 5, 227–234 CrossRef CAS PubMed.
- K. B. Yatsimirrskii, P. E. Strizhak and T. S. Ivaschenko, Potential of chaotic chemical systems in nanotrace analysis based on the Belousov–Zhabotinskii reaction (BrO3−–malonic acid–ferroin). Determination of manganese(II), Talanta, 1993, 40, 1227–1232 CrossRef.
- R. T. Echols, M. K. Caroll and J. F. Tyson, Flow injection study of the Belousov–Zhabotinskii reaction, Anal. Proc., 1995, 32, 3–5 RSC.
- L. Kolar-Anic and G. Schmitz, Mechanism of the Bray–Liebhafsky reaction: effect of the oxidation of iodous acid by hydrogen peroxide, J. Chem. Soc., Faraday Trans., 1992, 88, 2343–2349 RSC.
- S. Anic and L. Kolar-Anic, Kinetic aspects of the Bray–Liebhafsky oscillatory reaction, J. Chem. Soc., Faraday Trans. 1, 1988, 84, 3413–3421 RSC.
- S. Anic, L. Kolar-Anic, D. Stanisavljev, N. Begovic and D. Mitic, Dilution reinitiated oscillations in the Bray–Liebhafsky system, React. Kinet. Catal. Lett., 1991, 43, 155–162 CrossRef CAS.
- R. Jiménez-Prieto, M. Silva and D. Pérez-Bendito, Application of Oscillating Reaction-Based Determinations to the Analysis of Real Samples, Analyst, 1997, 122, 287–292 RSC.
- S. Anic, D. Mitic and M. Curcija, J. Serb. Chem. Soc., 1987, 52, 575–579 CAS.
- G. Schmitz, J. Chim. Phys. Phys.-Chim. Biol., 1987, 84, 957–965 CAS.
- A. Nagy and L. Treindl, Modifikacia Prietokoveho reaktora na sledovanie chemickych oscilacii, Chem. Listy, 1988, 82, 1097–1099 CAS.
- M. Yoshimoto, K. Yoshikawa, Y. Mori and I. Hanazaki, Asymmetric coupling stabilizes the out-of-phase mode: experimental evidence in the Belousov–Zhabotinsky reaction, Chem. Phys. Lett., 1992, 189, 18–22 CrossRef CAS.
- I. R. Epstein, The role of flow systems in far-from-equilibrium dynamics, J. Chem. Educ., 1989, 66, 191 CrossRef CAS.
- R. Melka, B. Olsen, L. Beavers and J. Draeger, The kinetics of oscillating reactions. Laboratory experiment for physical chemistry, J. Chem. Educ., 1992, 69, 596 CrossRef CAS.
- Z. Qingyuan and C. Jie, Fenxi Shiyanshi, 1988, 7, 4 Search PubMed.
- M. Jiang, Y. Li, X. Zhou, Z. Zhao, H. Wang and J. Mo, Kinetic determination of hexacyanoferrates by their inhibition of an oscillating chemical reaction, Anal. Chim. Acta, 1990, 236, 411–416 CrossRef CAS.
- R. Jiménez-Prieto, M. Silva and D. Pérez-Bendito, Analyte Pulse Perturbation Technique: A Tool for Analytical Determinations in Far-from-Equilibrium Dynamic Systems, Anal. Chem., 1995, 67, 729–734 CrossRef.
- R. Jiménez-Prieto, M. Silva and D. Pérez-Bendito, Determination of trace amounts of reduced glutathione by a chemical oscillating reaction, Analyst, 1996, 121, 563–566 RSC.
- R. Jiménez-Prieto, M. Silva and D. Pérez-Bendito, Determination of gallic acid by an oscillating chemical reaction using the analyte pulse perturbation technique, Anal. Chim. Acta, 1996, 321, 53–60 CrossRef.
- R. Jiménez-Prieto, M. Silva and D. Pérez-Bendito, Simultaneous determination of gallic acid and resorcinol based on an oscillating chemical reaction by the analyte pulse perturbation technique, Anal. Chim. Acta, 1996, 334, 323–330 CrossRef.
- M. Orbán, Oscillations and bistability in the copper(II)-catalyzed reaction between hydrogen peroxide and potassium thiocyanate, J. Am. Chem. Soc., 1986, 108, 6893–6898 CrossRef.
- M. Orbán, Cu(II)-catalyzed oscillatory chemical reactions, React. Kinet. Catal. Lett., 1990, 42, 343–353 CrossRef.
- K. Zhang, W. H. Ma, R. X. Cai, Z. X. Lin and N. Q. Gan, Determination of riboflavin by the perturbation of active oxygen on a chemical oscillating reaction, Anal. Chim. Acta, 2000, 413, 115–123 CrossRef.
- J. Z. Gao, H. Yang, X. Liu, J. Ren, X. Lu, J. Hou and J. Kang, Kinetic determination of ascorbic acid by the BZ oscillating chemical system, Talanta, 2001, 55, 99–107 CrossRef CAS PubMed.
- J. Z. Gao, H. Yang, X. Liu, J. Ren, Q. Li and J. Kang, Determination of glutamic acid by an oscillating chemical reaction using the analyte pulse perturbation technique, Talanta, 2002, 57, 105–114 CrossRef CAS PubMed.
- R. J. Prieto, M. Silva and D. P. Bendito, Application of Oscillating Reaction-Based Determinations to the Analysis of Real Samples, Analyst, 1997, 122, 287–292 RSC.
- J. Z. Gao, X. X. Wei and W. Yang, Determination of 1-naphthylamine by using oscillating chemical reaction, J. Hazard. Mater., 2007, 144, 67–72 CrossRef CAS PubMed.
- P. E. Strizhak, O. Z. Didenko and T. S. Ivashchenko, Determination of traces of thallium using the transient chaotic regime in the Belousov–Zhabotinskii oscillating chemical reaction, Anal. Chim. Acta, 2001, 428, 15–21 CrossRef CAS.
- N. D. Pejic, et al., Kinetic determination of morphine by means of Bray–Liebhafsky oscillatory reaction system using analyte pulse perturbation technique, Anal. Chim. Acta, 2007, 582, 367–374 CrossRef CAS PubMed.
- W. Yang, et al., Determination of alpha-naphthol by an oscillating chemical reaction using the analyte pulse perturbation technique, Anal. Chim. Acta, 2005, 554, 218–223 CrossRef CAS.
- H. Gang, L. Chen, J. Zheng, P. Chen, W. Wang, J. Song, L. Qiu, J. Song and L. Hu, Determination of Alizarin Red S using a novel B–Z oscillation system catalyzed by a tetraazamacrocyclic complex, Cent. Eur. J. Chem., 2009, 7(3), 291–297 Search PubMed.
- K. B. Yatsimirskii, L. P. Tikhonova and L. N. Zakrevskaya, New oscillating chemical reactions involving copper and nickel tetraazamacrocyclic complexes, React. Kinet. Catal. Lett., 1982, 21, 381–386 CrossRef CAS.
- L. Hu, G. Hu and H. H. Xu, Kinetic determination of Ag+ using a novel Belousov–Zhabotinskii oscillating system, J. Anal. Chem., 2006, 61, 1021–1025 CrossRef CAS.
- G. Hu, L. Hu and S. S. Ni, Oscillatory reactions in the lactic acid/acidic bromate system with a tetraazamacrocyclic nickel(II) complex as catalyst, React. Kinet. Catal. Lett., 2006, 88, 349–355 CrossRef CAS.
- G. Hu and Z. D. Zhang, A New Chemical Oscillator With a Macrocyclic Copper(II) Complex as Catalyst and Lactic Acid as the Substrate, Chem. Lett., 2006, 35, 1154–1155 CrossRef CAS.
- J. D. Xu and S. S. Ni, Oscillating reactions involving a nickel complex with a 13-membered tetraaza macrocyclic ligand in acidic bromate medium, Inorg. Chem., 1986, 25, 1264–1268 CrossRef CAS.
- G. Hu, L. Hu, Z. Q. Xu, F. X. Xie and S. S. Ni, Period of Homogeneous Oscillations in the B–Z System Involving a Tetraazamacrocyclic Complex, Asian J. Chem., 2004, 16, 1063–1068 CAS.
- G. Hu, Z. D. Zhang, L. Hu and J. M. Song, A New Oscillating Reaction of the Belousov–Zhabotinskii-Type With a Macrocyclic Copper(II) Complex as Catalyst, Transition Met. Chem., 2005, 30, 856–860 CrossRef CAS.
- G. Hu, P. Chen, W. Wang, L. Hu, J. Song, L. Qiu and J. Song, Kinetic determination of pyrogallol by a novel oscillating chemical reaction catalyzed by a tetraazamacrocyclic complex, Electrochim. Acta, 2007, 52, 7996–8002 CrossRef CAS.
- N. B. Ganaie and G. M. Peerzada, Catalyst, Co-ion and the media effect on the oscillatory behavior of resorcinol in the BZ reaction, J. Braz. Chem. Soc., 2009, 20, 1262–1267 CrossRef CAS.
- N. B. Ganaie and G. M. Peerzada, Effect of initial reagent concentrations on the oscillatory behavior of the BZ reaction in a batch reactor, Int. J. Chem. Kinet., 2009, 41, 650–657 CrossRef CAS.
- N. B. Ganaie, G. M. Peerzada and I. A. Shah, Effect of different counter-ions of the manganese salt as catalyst on the kinetics of resorcinol based Belousov–Zhabotinsky, Int. J. Chem. Kinet., 2013, 45, 343–353 CrossRef CAS.
- G. Bellucci, R. Bianchini and C. Chiappe, Bromination of alkenes in acetonitrile: a rate and product study, J. Org. Chem., 1991, 56, 3067–3073 CrossRef CAS.
- R. J. Field, E. Koros and R. M. Noyes, Oscillations in chemical systems. II. Thorough analysis of temporal oscillation in the bromate–cerium–malonic acid system, J. Am. Chem. Soc., 1972, 94, 8649–8664 CrossRef CAS.
- M. Orban, E. Koros and R. M. Noyes, Chemical oscillations during the uncatalyzed reaction of aromatic compounds with bromate. 2. A plausible skeleton mechanism, J. Phys. Chem., 1979, 83, 3056–3057 CrossRef CAS.
- S. Bohmdorfer, A. Patel, A. Hofinger, T. Netscher, L. Gille and T. Rosenau, Bromination of Tocopherols: Oxidative Halogenations and Rearrangements, Eur. J. Org. Chem., 2011, 3036–3049, DOI:10.1002/ejoc.201100153.
- N. B. Ganaie, G. M. Peerzada and U. Gull, In vitro Synthesis of Nearly Ultra High Molecular Weight (NUHMW) Polymers Using Solvent-Water Suspension Method, Colloid Polym. Sci., 2017, 295, 89–97 CAS.
- M. P. Ratnaparkhi and J. P. Gupta, Sustained Release Oral Drug Delivery System – An Overview, Int. J. Pharma Res. Rev., 2013, 2, 11–21 CrossRef.
- M. Jamini and A. Kothari, Sustained Release Matrix Type Drug Delivery System: A Review, J. Drug Delivery Ther., 2012, 2, 142–148 Search PubMed.
- P. V. Lalitha and R. Ramaswamy, Structure reactivity correlation in bromate oscillatory systems in aqueous–acetonitrile mixed medium, React. Kinet. Catal. Lett., 1993, 51, 431–442 CrossRef CAS.
|
This journal is © The Royal Society of Chemistry 2017 |
Click here to see how this site uses Cookies. View our privacy policy here.