DOI:
10.1039/C6RA25291E
(Paper)
RSC Adv., 2017,
7, 4237-4242
Fe3O4@SiO2 supported aza-crown ether complex cation ionic liquids: preparation and applications in organic reactions†
Received
15th October 2016
, Accepted 25th November 2016
First published on 16th January 2017
Abstract
A series of aza-crown ether ionic liquids supported on magnetic Fe3O4@SiO2 core–shell particles were designed, synthesized and characterized by elemental analysis, TEM, TG and FT-IR. These new aza-crown ether complex cation ionic liquids were utilized as heterogeneous acidic catalysts in Friedel–Crafts alkylation and Hantzsch reaction in good yields under convenient reaction conditions. Moreover, these magnetic particle supported IL catalysts could be readily recovered by an external magnet and reused five times without obvious loss of activity.
Introduction
Ionic liquids composed entirely of ions have received much attention since they can be functionalized to be catalysts in light of their specific properties and various purposes.1–3 Although thousands of ILs have been fabricated over the past decades, they commonly consisted of quaternary ammonium, N,N′-dialkylimidazolium and N-alkylpyridinium cations.4,5 Differing from these traditional ionic liquids, a series of novel crown ether complex cation ionic liquids were defined and applied to different organic reactions in 2011.6 Recently, a range of aza-crown ether complex cation ionic liquids, Brønsted and Lewis acidic aza-crown ether complex cation ionic liquids have been reported.7–9 Those ionic liquids have been utilized in different organic reactions, such as Henry, Michael addition, Biginelli, and Mannich reaction etc. Nonetheless they couldn't be recycled conveniently as homogeneous catalysts. By transforming from a homogeneous catalyst to a heterogeneous catalyst, they could be immobilized to overcome this issue.10
In accordance with the principles of green chemistry, the researches on the area of separable, reusable, non-toxic, low-cost, and insoluble catalysts have become more and more important in chemistry. Design and preparation of more efficient and stable catalysts has been a main goal for chemists in organic synthesis based on environmental and economic consideration.11–13 Ionic liquids are these kinds of catalysts possessing green and environmentally benign features. Recently, the immobilization of structurally well-devised ionic liquids has been proven to be an effective approach to solve the problems of catalyst leaching and product extracting from ionic liquids.14,15 Moreover, the magnetic nanoparticles (MNPs) as excellent supporters for ionic liquids have been well investigated because of their easy synthesis and functionalization, high surface area and facile separation under a magnetic field instead of traditional supporters.16 Although unmodified MNPs have these advantages, they aggregate into large clusters in terms of their anisotropic dipolar attraction leading to deleterious effect on their properties. A suitable protection of silica layers can be coated onto the surfaces of nanoparticles to prevent their aggregation.17,18 For instance, N-methylimidazolium chloride ionic liquids are bonded by covalently immobilizing onto the surfaces of SiO2 by reaction of alkoxysilyl groups with hydroxyl groups of the surfaces.19,20
According to the understanding aforementioned, we have fabricated a series of magnetically separable aza-crown ether complex cation ionic liquids. In this work, the crown ether ionic liquids were also immobilized onto the interfaces of magnetic core–shell particles Fe3O4@SiO2 by forming C–N bond between the nitrogen atom of the aza-crown ether and the carbon atom of carriers. Comparing with homogeneous acid catalysts, such as H2SO4, HF, and H3PO4, generally used by chemists in industrial catalytic processes, these new heterogeneous catalysts exhibited a good acidity and could be separated from the final products without a requirement of neutralization.
Indoles and their derivatives are known as an important class of heterocyclic compounds in pharmaceutical as well as synthetic chemistry.21–24 The most ubiquitous alkaloids are based on the indole moiety. Particularly bisindolylmethanes (BIMs) are widely existed in various natural products and exhibit a wide range of biological activities including anticancer activity against several cancer cell lines.25,26 Bisindolylmethanes have been commonly prepared by reactions of indoles with various aldehydes or ketones in the presence of either Brønsted or Lewis acids.27–29 Herein, the supported aza-crown ether complex cation ionic liquid containing [HSO4−] was applied to the Friedel–Crafts alkylation reaction as a good heterogenous catalyst.
Classical Hantzsch reaction provides a convenient method to approach 1,4-dihydropyrimidines (1,4-DHPs) as scaffold in useful drugs30,31 from 1,3-dicarbonyl compounds, aldehydes and ammonia in acetic acid at room temperature or in alcohols under refluxing for a long time.32,33 However, these methodologies still suffer from some drawbacks.34–37 In this context, the heterogeneous catalysts of supported aza-crown ether complex cation ionic liquid containing counter anion of [BF4−] can smoothly initiate this traditional reaction.
Experimental
General
All reagents were obtained from commercial resources and used without further purification. Elemental analyses were carried out on a Carioel elemental analyzer. FT-IR spectra were recorded on a Nicolet Fourier-transform infrared spectrometer (NEXUS 670) using a KBr pallet in the range of 400–4000 cm−1. TEM images were photographed on a field emission transmission electron microscope (Tecnai-G2-F30). Thermal gravimetric (TG) analyses were measured on a Linseis STA PT 1600 thermoanalyzer. The analyses were carried out in N2 atmosphere from room temperature to 800 °C with a heating rate of 10 °C min−1. The synthetic route for the magnetic core–shell particle supported aza-crown ether complex cation ionic liquids was illustrated in Scheme 1.
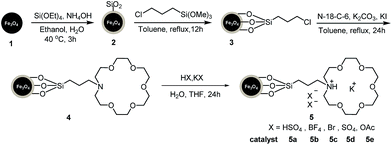 |
| Scheme 1 Synthetic route for the magnetic core–shell particle supported aza-crown ether complex cation ionic liquids. | |
Preparation of catalysts
Synthesis of Fe3O4 particles. The magnetic particles of Fe3O4 were prepared through a solvothermal reaction.11 Briefly, 2.70 g of FeCl3·6H2O and 7.20 g of sodium acetate were dissolved in 100 mL of ethylene glycol under magnetic stirring. The obtained homogeneous yellow solution was transferred to a Teflon-lined stainless-steel autoclave and sealed to heat at 200 °C for 8 h. After completion, the autoclave was cooled down to room temperature. The obtained black magnetite particles were washed with ethanol for 6 times, and then dried in vacuum at 60 °C for 12 h.
Synthesis of Fe3O4@SiO2 microspheres. Typically, 200 mg of the prepared Fe3O4 MNPs were dispersed in a mixture of 320 mL of ethanol and 80 mL of distilled water by ultrasonication for 15 min. After adding 12 mL of ammonia solution (25%) to the mixture under continuous mechanical stirring, 1.2 mL of tetraethyl orthosilicate (TEOS) was added dropwise. The reaction was then allowed to proceed at 40 °C for 3 h. The solid products were collected using an external magnetic bar, rinsed with water and ethanol, dried in a vacuum drying oven at 60 °C for 6 h.38
γ-Chloropropyltrimethoxysilane supported on Fe3O4@SiO2. After dispersing Fe3O4@SiO2 and γ-chloropropyltrimethoxy-silane (weight ratio 1
:
1) into dehydrated toluene, the mixture was refluxed for 48 h under argon. When the reaction was completion, toluene was removed from mixture by a distillation under reduced pressure, and the excess γ-chloropropyltrimethoxy-silane was removed by washing with anhydrous ethanol several times. The modified magnetic particles were then dried in vacuum at 60 °C for 12 h.
Aza-18-crown-6 immobilized on Fe3O4@SiO2. 1.00 g obtained product 3, 0.848 g (8 mmol) anhydrous Na2CO3 and 13 mg (0.08 mmol) KI were added in sequence to a toluene solution of 0.21 g (0.8 mmol) aza-18-crown-6. The resulting mixture was refluxed for 24 h under argon.39 After cooling to room temperature, the catalysts were separated from the liquid phase by an external magnetic bar. The solid products were isolated by evaporating the solvent under reduce pressure, washed with water, anhydrous ethanol and dichloromethane several times, and then dried in vacuum at 60 °C for 12 h.
Aza-crown ether complex cation ionic liquids on Fe3O4@SiO2. To a solution of 4 (1.00 g, 0.45 mmol), 10 mL H2O, 10 mL THF, 78 mg KHSO4 (0.45 mmol), and 0.45 mL H2SO4 (1 mol L−1) were added in sequence. The obtained solution was then stirred for 24 h at room temperature. The excess THF and water was evaporated under reduced pressure. The desired product 5a was obtained by drying at 80 °C under vacuum for 12 h.The synthesis methods of other magnetic particle supported aza-crown ether complex cation ionic liquids (5b–5e) are very similar to magnetic particle supported aza-crown ether complex cation ionic liquid 5a.
General procedure for the synthesis of bisindolylmethanes
Aldehyde (1.00 mmol), indole (2.00 mmol, 0.2343 g) and catalyst 5a (0.1 mmol, 0.2222 g) were added in 2 mL solvent. The mixture was stirred at 30 °C for appropriate time. The reaction was monitored by TLC. After completion, catalyst 5a was separated by an external magnet from reaction mixture, washed with 3 × 5 mL CH2Cl2. After evaporating solvent, the residue was then purified by a flash column chromatography (eluent: petroleum ether/ethyl acetate) yielding target product. To examine the reusability of catalyst, the recovered 5a was dried at 60 °C in a vacuum dry oven for the next cycle.
General procedure for the Hantzsch reaction
Aldehyde (1 mmol), ethyl acetoacetate (3 mmol, 0.38 mL), ammonium acetate (1 mmol, 0.0771 g) and catalyst 5b (0.1 mmol, 0.2222 g) were added to ethanol (2 mL) and heated to reflux for 2 h. The reaction was monitored by TLC. The mixture was cooled down to room temperature when aldehyde was disappeared. Catalyst 5b was recovered by an external magnet. The solvent was evaporated under reduced pressure to yield the crude product, which was then purified by flash column chromatography (eluent: petroleum ether/ethyl acetate). To examine the reusability of catalyst, the recovered catalyst 5b was dried at 60 °C in vacuum for the next cycle.
Results and discussion
The magnetic nano-particles Fe3O4 (1), SiO2 coated core–shell particles Fe3O4@SiO2 (2), silane modified particles (3), aza-crown ether functionalized particles (4) and magnetic core–shell particle supported aza-crown ether metal complex ionic liquids (5) were successfully fabricated step by step (vide infra Scheme 1). The TEM of catalyst 5a are illustrated in Fig. 1 and verify the core–shell structure of particles. The related mapping pictures demonstrate that the distributions of C, N, O, S, Si, and Fe elements are well arranged (Fig. 2). The crystal faces (111) and (311) of Fe3O4 can be seen in the HRTEM picture (Fig. S8†). The elemental analyses of particles 3, 4 and catalyst 5a are summarized in Table 1. The percentage of 3.96% carbon and 0.85% hydrogen clearly indicate that silanes are successfully hybridized onto particles 3. The percentage of 0.64% nitrogen in particles 4 shows that the aza-crown ethers are introduced in the particles via a covalent bond of C–N. The percentage of 2.85% sulfur in catalyst 5a demonstrates that equivalent moles of H2SO4 and chelates KHSO4 are really penetrated into the aza-crown ether skeleton, in which, the loading is 0.44 mmol of aza-crown ether complex cation ionic liquids on per gram magnetic supporters of Fe3O4@SiO2.
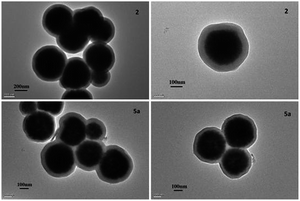 |
| Fig. 1 TEM of Fe3O4@SiO2 (2) and catalyst 5a. | |
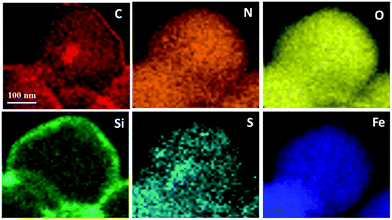 |
| Fig. 2 The mapping of 5a. | |
Table 1 The elemental analyses of nanoparticle 3, 4, 5a
Entry |
Substance |
Elemental analyses (%) |
Organic component (mmol g−1) |
S |
N |
C |
H |
Catalyst 5a after five times reuse in Friedel–Crafts alkylation. |
1 |
3 |
— |
— |
3.96 |
0.85 |
1.10 |
2 |
4 |
— |
0.63 |
12.53 |
2.22 |
0.45 |
3 |
5a |
2.84 |
0.62 |
12.45 |
2.15 |
0.44 |
4 |
5a |
2.55 |
0.61 |
12.76 |
2.19 |
0.40a |
In Fig. S1,† IR band around 570 and 475 cm−1 are attributed to characteristic vibrations of Fe–O bands in all samples. When SiO2 particles are coated to Fe3O4 particles producing core–shell particles of Fe3O4@SiO2·2, a very strong band around 1100 cm−1 and a wide weak band of 3430 cm−1 should be assigned to the stretching vibration of Si–O–Si and Si–OH bonds, respectively. The peak at 2957 cm−1 significantly belongs to stretching vibrations of C–H in alkyl group of 3. When the aza-crown ether is combined with organic silane in particles 4, and 5a, the S
O stretching vibrations40 is close to the Si–O field at 1000–1150 cm−1 leading to a slight change of the peak. The peak at 619 cm−1 in fingerprint region of 5a is attributed to the bending vibration of S
O bonds. A new medium band in the range of 1635 cm−1 should be the combination peak of 587 and 1105 cm−1 in catalyst 5a.
The thermal properties of the catalysts 5a and 5b are determined by TG analyses and the results are illustrated in Fig. S1 and S2.† Generally, the decomposition points of catalysts are up to 235 °C shown their good stability. The 14% weight losses of catalysts appeared between 230 °C and 400 °C is assigned to the decomposition of aza-18-C-6 component.
In our previous studies, we have reported the use of an acidic Brønsted ionic liquids aza-[18-C-6KH][HSO4]2 as an homogeneous catalyst for the synthesis of bis-indolylmethanes in good to excellent yields.8 In this work, aza-crown ether complex cation ionic liquids are immobilized on the surfaces of Fe3O4@SiO2 particles as heterogeneous catalysts for the synthesis of bis-indolylmethanes. The results are summarized in Table 2. Initially, the catalyst 5a–e are demonstrates various activities in this Friedel–Crafts alkylation reaction, in which, 5a with a Brønsted anion of HSO4− reveals the better activity than 5b–e (Table 2 entries 1–4 vs. 5–8). In contrast, a blank reaction is carried out using a silica coated magnetic particles Fe3O4@SiO2 as catalyst and showing no activity in the absence of aza-crown ether complex cation ionic liquids (Table 2, entry 9). In addition, different solvents are also screened (Table 2, entries 1–4), which the methanol is evidently the optimal solvent. As comparison, the traditional imidazole cation ionic liquid can only achieved a Friedel–Crafts alkylation reaction in 31% yield.41
Table 2 Screening of catalysts in the reaction of benzaldehyde and indolea
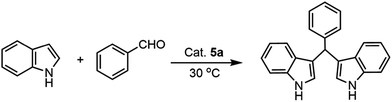
|
Entry |
Catalyst |
Solvent |
Yieldb (%) |
Reaction conditions: indole 2.0 mmol, benzaldehyde 1.0 mmol, catalyst 5a 0.1 mmol, solvent 2 mL, 30 °C, 1.5 h. Isolated yield. |
1 |
5a |
CH3OH |
92 |
2 |
5a |
CH2Cl2 |
82 |
3 |
5a |
C2H5OH |
86 |
4 |
5a |
CH3CN |
89 |
5 |
5b |
CH3OH |
Trace |
6 |
5c |
CH3OH |
10 |
7 |
5d |
CH3OH |
26 |
8 |
5e |
CH3OH |
41 |
9 |
Fe3O4@SiO2 |
CH3OH |
NA |
Under the optimized reaction conditions, this Friedel–Crafts alkylation reaction is then extended to different substrates (Table 3). It can be seen that excellent yields are obtained from a variety of aldehydes. Obviously, both the electron-withdrawing groups and the electron-donating groups of aromatic aldehydes have no negative effects on this reaction (Table 3, entries 1–10). Aliphatic aldehydes and ketones can also take place this reaction under mild conditions in good yields (Table 3, entries 11–14).
Table 3 Reaction of aldehydes with indole over catalyst 5aa
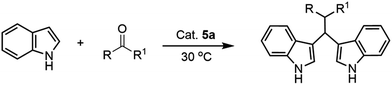
|
Entry |
R |
R1 |
Time/h |
Yieldb (%) |
Reaction conditions: indole 2.0 mmol, substrate 1.0 mmol, catalyst 5a 0.1 mmol, methanol 2 mL, r.t. Isolated yield. |
1 |
2-NO2C6H4 |
H |
1 |
90 |
2 |
3-NO2C6H4 |
H |
1 |
92 |
3 |
4-NO2C6H4 |
H |
1 |
95 |
4 |
3-ClC6H4 |
H |
1 |
92 |
5 |
4-ClC6H4 |
H |
1 |
93 |
6 |
4-BrC6H4 |
H |
1 |
95 |
7 |
2-CH3OC6H4 |
H |
1 |
91 |
8 |
3-CH3OC6H4 |
H |
1 |
95 |
9 |
4-CH3C6H4 |
H |
1 |
93 |
10 |
4-OHC6H4 |
H |
0.5 |
96 |
11 |
CH3CH2CH2 |
H |
3 |
88 |
12 |
CH3(CH2)4CH2 |
H |
3 |
89 |
13 |
R + R1: CH2(CH2)3CH2 |
3 |
85 |
14 |
C6H5 |
CH3 |
3 |
81 |
A plausible mechanism for this Friedel–Crafts alkylation reaction in the presence of supported ILs is proposed and is similar to our previous report9 (Scheme 2). First, the carbonyl group of the aldehyde is activated by the anion [HSO4]− and attacked by a molecule of indole to generate intermediate I. After the loss of H2O to afford intermediate II, it is attacked by another molecule of indole to afford intermediate III. The bis(indolyl)methane 6 is finally formed with the leaving of cation [18C-6K]+. Meanwhile, the catalyst of ionic liquid [18-C-6K][HSO4] is regenerated for the next catalytic cycle.
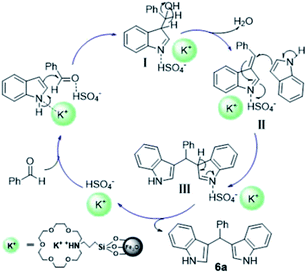 |
| Scheme 2 Proposed mechanism for Friedel–Crafts alkylation catalysed by IL. | |
As a continuation studies of the application of crown ether complex cation IL in organic reactions, we report in this article a novel and efficient synthesis of dihydropyridines by a classical Hantzsch reaction using supported aza-crown ether complex cation ionic liquids as catalysts under mild conditions. After screening different catalysts (Table 4, entries 1–5), the catalysts 5a and 5b shown better catalytic activities than other catalysts because that 5a is acidic and able to activate the carbonyl group; BF4− in 5b has more affinity with ammonium group than the others. As comparison, the blank experiment was carried out and gave 48% yield in the present of carriers Fe3O4@SiO2 (Table 4, entry 6). When the ratio of benzaldehyde (BA)
:
ethyl acetoacetate (EAAT)
:
ammonium acetate (AAT) was 1
:
3
:
1, the highest yield of 92% could be obtained using 5b as catalyst (Table 4, entry 2).
Table 4 Screening of catalysts in Hantzsch reactiona

|
Entry |
Catalyst |
Yieldb (%) |
Reaction conditions: EAAT 3.0 mmol, BA 1.0 mmol, AAT 1.0 mmol, catalyst 0.1 mmol, ethanol 2 mL, reflux, 2 h. Isolated yield. |
1 |
5a |
83 |
2 |
5b |
92 |
3 |
5c |
58 |
4 |
5d |
65 |
5 |
5e |
61 |
6 |
Fe3O4@SiO2 |
48 |
It can be explained by a proposed mechanism depicted in Scheme 3.42 Initially, ethyl acetoacetate might be activated by BF4− anion to form an enolate anion 1 that attacks benzaldehyde to produce intermediate 2. Under aid of IL catalyst, ammonium acetate reacts with 2 generating intermediated 3 accompanying with the loss of water and HOAc. The intermediated 3 can be then transformed by 4 that is immediately attacked by 1 to produce intermediate 5. The target product 6 is then obtained by an intramolecular cyclization of 5 in the presence of ILs. We can see that the excessive amount of ethyl acetoacetate could promote increase of intermediate 2 as well as 5 to enhance better yield.
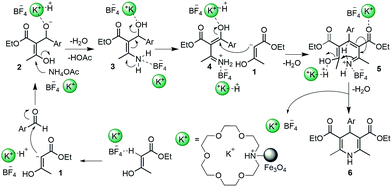 |
| Scheme 3 Proposed mechanism for the formation of 1,4-DHP compounds. | |
To extend the scope of this reaction, a variety of substituted aldehydes were investigated under the optimal conditions. The results are listed in Table 5. It can be seen that the substituted aromatic aldehydes and olefin aldehydes can generate the target products readily (Table 5, entries 1–17). When the temperature was decreased from reflux to 40 °C, the yield deceased obviously due to low activity of benzaldehyde (Table 5, entry 2). The aromatic aldehydes bearing electro-withdrawing group were superior to this reaction than that with electron-donating group (Table 5, entries 3–5 vs. 9–15) since the electron-donating group in aldehyde could stabilized intermediate 2. In contrast, the n-butylaldehyde gave the worst yield of 61%.
Table 5 Hantzsch reaction over catalyst 5ba
Entry |
R |
Yieldb (%) |
Entry |
R |
Yield (%) |
Reaction conditions: BA 1.0 mmol, EAAT 3.0 mmol, AAT 1.0 mmol, catalyst 5b 0.1 mmol, ethanol 2 mL, reflux, 2 h. Isolated yield. Temperature 40 °C. |
1 |
C6H5 |
92 |
11 |
3-CH3OC6H5 |
85 |
2 |
C6H5 |
53c |
12 |
4-CH3OC6H4 |
82 |
3 |
2-NO2C6H4 |
88 |
13 |
2,5-CH3OC6H4 |
74 |
4 |
3-NO2C6H4 |
95 |
14 |
4-HOC6H4 |
80 |
5 |
4-NO2C6H5 |
96 |
15 |
4-OH-3-CH3OC6H4 |
71 |
6 |
4-ClC6H4 |
93 |
16 |
Furfural |
96 |
7 |
2,4-ClC6H4 |
92 |
17 |
Picolinaldehyde |
72 |
8 |
4-BrC6H4 |
90 |
18 |
Cinnamaldehyde |
94 |
9 |
4-CH3C6H4 |
86 |
19 |
Citral |
88 |
10 |
2-CH3OC6H4 |
85 |
20 |
Butylaldehyde |
61 |
The catalyst recovery was quite simple. Upon completion of the reaction, the solid catalyst was easily recovered by an external magnetic bar. The catalytic activities of recycling catalysts of 5a and 5b were both investigated and illustrated in Fig. 3. The yields decreased slightly after five times reuse which could be attributed to the loss of anion that was confirmed by elemental analysis of used catalyst due to washing (Table 1, entry 4). The TEM of used catalyst 5a manifests that the core–shell structure of nano-particles is stable (Fig. S9†).
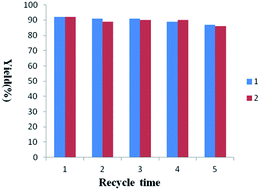 |
| Fig. 3 Recycling results: 5a in Friedel–Crafts alkylation (1), 5b in Hantzsch reaction (2). | |
Conclusions
In summary, we devised and synthesized a series of aza-crown ether complex cation ionic liquids supported by Fe3O4@SiO2. Compared with crown ether complex cation ionic liquids, the new supported ionic liquids have protons enabling the catalytic activity of the cations and can increase effectively the electrophilicity of the carbonyl. Then we investigated their applications in Friedel–Crafts alkylation and Hantzsch reaction. The magnetic core–shell nano-particle Fe3O4@SiO2 supported ILs not only achieved good yields in these reactions, but also can be reused for at least five times by magnetic separation conveniently without significant loss of catalytic activity. Further discoveries on the applications of these supported aza-crown ether complex cation ionic liquids are underway in our laboratory.
Acknowledgements
This work was funded by the National Natural Science Foundation of China (NSFC 21173106, 21401091), the Fundamental Research Funds for the Central University (lzujbky-2016-K09) and by the Foundation of State Key Laboratory of Coal Conversion (Grant No. J16-17-913).
Notes and references
- H. Li, P. S. Bhadury, B. Song and S. Yang, RSC Adv., 2012, 2, 12525–12551 RSC.
- H. Olivier-Bourbigou, L. Magna and D. Morvan, Appl. Catal., A, 2010, 373, 1–55 CrossRef CAS.
- Z. S. Qureshi and K. M. Deshmukh, Clean Technol. Environ. Policy, 2014, 16, 1487–1513 CrossRef.
- S. Udayakumar, S. W. Park, D. W. Park and B. S. Choi, Catal. Commun., 2008, 9, 1563–1570 CrossRef CAS.
- X. X. Zheng, S. Z. Luo, L. Zhang and J. P. Cheng, Green Chem., 2009, 11, 455–458 RSC.
- Y. Y. Song, H. W. Jing, B. Li and D. S. Bai, Chem.–Eur. J., 2011, 17, 8731–8738 CrossRef CAS PubMed.
- Y. Y. Song, C. Cheng and H. W. Jing, Chem.–Eur. J., 2014, 20, 12894–12900 CrossRef CAS PubMed.
- C. Cheng and H. W. Jing, RSC Adv., 2014, 4, 34325–34331 RSC.
- Y. Liang, J. Wang, C. Cheng and H. Jing, RSC Adv., 2016, 6, 93546–93550 RSC.
- B. W. Xin and J. C. Hao, Chem. Soc. Rev., 2014, 43, 7171–7187 RSC.
- A. Q. Wang, X. Liu, Z. X. Su and H. W. Jing, Catal. Sci. Technol., 2014, 4, 71–80 CAS.
- D. S. Bai, Q. Wang, Y. Y. Song, B. Li and H. W. Jing, Catal. Commun., 2011, 12, 684–688 CrossRef CAS.
- H. W. Jing, X. M. Wang, Y. Liu and A. Q. Wang, Chin. J. Catal., 2015, 36, 244–251 CrossRef CAS.
- J. H. Ji, P. H. Zeng, S. F. Ji, W. Yang, H. F. Liu and Y. Y. Li, Catal. Today, 2010, 158, 305–309 CrossRef CAS.
- D. D. Shao, K. K. Xu, X. J. Song, J. H. Hu, W. L. Yang and C. C. Wang, J. Colloid Interface Sci., 2009, 336, 526–532 CrossRef CAS PubMed.
- Y. H. Deng, D. W. Qi, C. H. Deng, X. M. Zhang and D. Y. Zhao, J. Am. Chem. Soc., 2008, 130, 28–29 CrossRef CAS PubMed.
- D. W. Kim and D. Y. Chi, Angew. Chem., Int. Ed., 2004, 43, 483–485 CrossRef CAS PubMed.
- A. Riisager, P. Wasserscheid, R. van Hal and R. Fehrmann, J. Catal., 2003, 219, 452–455 CrossRef CAS.
- T. Selvam, A. Machoke and W. Schwieger, Appl. Catal., A, 2012, 445–446, 92–101 CrossRef CAS.
- L. Zhu, D. Pan, L. Ding, F. Tang, Q. L. Zhang, Q. Liu and S. Z. Yao, Talanta, 2010, 80, 1873–1880 CrossRef CAS PubMed.
- Z. Y. Lu, J. Dai, X. N. Song, G. Wang and W. S. Yang, Colloids Surf., A, 2008, 317, 450–456 CrossRef CAS.
- T. Sasaki, M. Tada, C. M. Zhong, T. Kume and Y. Iwasawa, J. Mol. Catal. A: Chem., 2008, 279, 200–209 CrossRef CAS.
- M. V. Khedkar, T. Sasaki and B. M. Bhanage, ACS Catal., 2013, 3, 287–293 CrossRef CAS.
- T. P. Pathak, J. G. Osiak, R. M. Vaden, B. E. Welm and M. S. Sigman, Tetrahedron, 2012, 68, 5203–5208 CrossRef CAS PubMed.
- K. R. M. Naidu, S. I. Khalivulla, S. Rasheed, S. Fakurazi, P. Arulselvan, O. Lasekan and F. Abas, Int. J. Mol. Sci., 2013, 14, 1843–1853 CrossRef CAS PubMed.
- D. K. Sharma, A. K. Tripathi, R. Sharma, R. Chib, R. U. Rasool, A. Hussain, B. Singh, A. Goswami, I. A. Khan and D. Mukherjee, Med. Chem. Res., 2014, 23, 1643–1653 CrossRef CAS.
- T. Abe, S. Nakamura, R. Yanada, T. Choshi, S. Hibino and M. Ishikura, Org. Lett., 2013, 15, 3622–3625 CrossRef CAS PubMed.
- A. S. Azmi, A. Ahmad, S. Banerjee, V. M. Rangnekar and R. M. Mohammad, Pharm. Res., 2008, 25, 2117–2124 CrossRef CAS PubMed.
- D. K. Sharma, B. Rah, M. R. Lambu, A. Hussain, S. K. Yousuf, A. K. Tripathi, B. Singh, G. Jamwal, Z. Ahmed, N. Chanauria, A. Nargotra, A. Goswami and D. Mukherjee, MedChemComm, 2012, 3, 1082–1091 RSC.
- M. L. Deb and P. J. Bhuyan, Tetrahedron Lett., 2006, 47, 1441–1443 CrossRef CAS.
- C. Velázquez and E. E. Knaus, Bioorg. Med. Chem., 2004, 12, 3831–3840 CrossRef PubMed.
- S. V. Nadkarni, M. B. Gawande, R. V. Jayaram and J. M. Nagarkar, Catal. Commun., 2008, 9, 1728–1733 CrossRef CAS.
- P. R. Singh, D. U. Singh and S. D. Samant, Synth. Commun., 2005, 35, 2133–2138 CrossRef CAS.
- Y. L. Chen, K. C. Fang, J. Y. Sheu, S. L. Hsu and C. C. Tzeng, J. Med. Chem., 2001, 44, 2374–2377 CrossRef CAS PubMed.
- J. P. Wan and Y. Y. Liu, RSC Adv., 2012, 2, 9763–9777 RSC.
- J. E. Biggs-Houck, A. Younai and J. T. Shaw, Curr. Opin. Chem. Biol., 2010, 14, 371–382 CrossRef CAS PubMed.
- L. M. Wang, J. Sheng, L. Zhang, J. W. Han, Z. Y. Fan, H. Tian and C. T. Qian, Tetrahedron, 2005, 61, 1539–1543 CrossRef CAS.
- G. Sabitha, G. S. K. K. Reddy, C. S. Reddy and J. S. Yadav, Tetrahedron Lett., 2003, 44, 4129–4131 CrossRef CAS.
- S. Ko, M. N. V. Sastry, C. C. Lin and C. F. Yao, Tetrahedron Lett., 2005, 46, 5771–5774 CrossRef CAS.
- C. S. Reddy and M. Raghu, Chin. Chem. Lett., 2008, 19, 775–779 CrossRef CAS.
- M. Cai and X. Wang, Asian J. Chem., 2015, 27(2), 649–653 CrossRef CAS.
- X. Z. Liang, Ind. Eng. Chem. Res., 2014, 53, 17325–17332 CrossRef CAS.
Footnotes |
† Electronic supplementary information (ESI) available: The FT-IR spectra and TGA curves of supported aza-crown ether cation ionic liquids. See DOI: 10.1039/c6ra25291e |
‡ Contribution equally. |
|
This journal is © The Royal Society of Chemistry 2017 |