DOI:
10.1039/C6RA25038F
(Paper)
RSC Adv., 2017,
7, 2766-2772
Single-molecule magnet behavior in a mononuclear dysprosium(III) complex with 1-methylimidazole†
Received
11th October 2016
, Accepted 21st December 2016
First published on 12th January 2017
Abstract
Three novel mononuclear lanthanide complexes coordinated with 1-methylimidazole [Ln(hfac)3·(1-MeIm)2] (Ln = Dy(1), Tb(2) and Ho(3), hfac = hexafluoroacetylacetonate, 1-MeIm = 1-methylimidazole) have been designed and characterized by single-crystal X-ray diffraction, luminescence and magnetic measurements. Studies indicate that the metal ions of all three mononuclear complexes adopt a slightly distorted D4d [LnO6N2] coordination environment, which is formed by two terminal 1-methylimidazole ligands and three bischelating hfac− anions. Frequency dependent out-of-phase signals were observed for complex 1 in dynamic magnetic susceptibility measurements, which indicates single-molecule magnetic behavior.
Introduction
Single molecular magnets (SMMs) have been an attractive research field owing to the memory effect exhibited by individual molecules, thus making them candidates for potential applications of high-density data storage technologies, quantum computing and single-spin manipulation.1 The large spin and strong intrinsic magneto-anisotropy of lanthanide ions (especially DyIII, TbIII, HoIII and ErIII) make them very attractive to construct SMMs of high spin-reversal barriers.2 In the past decade, many Ln-based SMMs have been reported, for example, mononuclear,3 dinuclear,4 trinuclear,5 tetranuclear6 pentanuclear7 and polynuclear8 lanthanide complexes. However, the challenge is to suppress the quantum tunneling of magnetization (QTM) in order to increase the anisotropy barrier, which depends mainly on the crystal field, inter-molecular interactions as well as the hyperfine interactions.3a,8a,9 Our previous work showed that [Dy(hfac)3(pz)2] (pz = pyrazole)10 was an SMM with a barrier of 28.32 K, and how a small change of the ligand field or intermolecular Dy⋯Dy dipolar coupling affects the magnetic properties of DyIII SMMs. To further investigate the effect of inter-molecular interactions on SMM behavior, it is interesting to design a similar compound using 1-methylimidazole instead of pyrazole. The presence of the methyl group will affect the intermolecular Ln⋯Ln dipolar coupling of lanthanide complexes, which will give rise to subtle but intriguing changes of the magnetic properties.11,12
As mentioned above, by utilizing the 1-methylimidazole (1-MeIm) ligand, three novel mononuclear lanthanide complexes [Ln(hfac)3·(1-MeIm)2] [Ln = Dy(1), Tb(2) and Ho(3)] have been obtained and characterized. Surprisingly, by using 1-methylimidazole instead of pyrazole to coordinate with Dy(hfac)3 units in complex 1, it significantly increases the energy barrier of Dy(III) ion from 28.32 to 42.54 K, with the change of ligand field symmetry from D2d for Dy(III) ion to D4d. In addition, the expansion of intermolecular Dy–Dy distances from 8.87 Å to 9.10 Å, as we expected, decreases the intermolecular Dy(III) coupling and suppresses QTM to some extent. Complex 1 represents an example of mononuclear lanthanide complexes displaying single-ion magnetic behavior.
Meanwhile, lanthanide compounds can exhibit highly efficient and long-lived luminescence with well-resolved multiline emissions in the UV-Vis.9a,13 So the luminescence spectra of complexes 1 and 2 are also investigated in the visible spectrum.
Experimental
Materials and physical techniques
All chemicals and reagents used in the syntheses were of analytical grade without further purification. C, H, N analyses were obtained on a Perking-Elmer elemental analyzer model 240. Fourier transform infrared spectroscopy was carried out in the range 400–4000 cm−1 on a Bruker TENOR 27 spectrophotometer using KBr pellets. Luminescence spectra and fluorescence lifetime studies were performed by using solid samples with an Edinburgh FLS-920P spectrophotometer. Inductively coupled plasma optical emission spectroscopy (ICP-OES) was measured with central mental (Dy, Tb, and Ho) by Spectro-Blue. All magnetic data were measured on a SQUID MPMS XL-7 magnetometer using polycrystalline samples. Diamagnetic corrections of experimental magnetic susceptibility data are estimated from Pascal's constants.9h,14
Synthesis of [Ln(hfac)3·(1-MeIm)2] (Ln = Dy(1), Tb(2), Ho(3))
Dy(hfac)3·2H2O (0.05 mmol, 0.041 g) was suspended in 20 ml of n-heptane and heated with stirring. After refluxing for about 2 h and cooling to 60 °C, 1-methylimidazole (0.1 mmol, 0.082 g) in CH2Cl2 (5 ml) was added, and the mixture was stirred for 30 minutes. By cooling the solution to room temperature, the filtrate was slowly evaporated at 4 °C. Colorless crystals of the product suitable for X-ray crystallography were obtained after a week. Complex 2 and 3 were synthesized following a similar procedure except that Dy(hfac)3·2H2O was substituted by Tb(hfac)3·2H2O and Ho(hfac)3·2H2O respectively. Yield: 21.8 mg, 45.9%, based on Dy. Anal. calcd (1): C23H15DyF18N4O6: C, 29.14; H, 1.60; N, 5.91. Found (1): C, 28.89; H, 1.60; N, 5.95%. ICP-OES anal. Found: Dy, 17.14; calcd. Found: 17.45; IR (KBr, ν/cm−1): 3150(w), 1645(vs), 1535(w), 1485(s), 1248(s), 1189(s), 1138(vs), 1096(s), 935(w), 800(s), 757(w), 664(vs), 580(s). Yield: 21.8 mg, 46.2%, based on Tb. Anal. calcd (2): C23H15TbF18N4O6: C, 29.21; H, 1.59; N, 5.95%. Found (2): C, 28.85; H, 1.61; N, 5.94% ICP-OES anal. Found: Tb, 16.83; calcd. Found: Tb, 16.51; IR (KBr, ν/cm−1): 3152(w), 1645(vs), 1535(w), 1468(s), 1256(s), 1189(s), 1138(vs), 1096(s), 935(w), 800(s), 757(w), 656(vs), 582(s) anal. calcd (3): C23H15HoF18N4O6: C, 29.07; H, 1.59; N, 5.90. Yield: 21.2 mg, 44.6%, based on Ho. Found (3): C, 29.13; H, 1.63; N, 5.88%. ICP-OES Anal. Found: Ho, 17.36; calcd. Found: Ho, 17.75; IR (KBr, ν/cm−1): 3150(w), 1644(vs), 1535(w), 1476(s), 1255(s), 1189(s), 1137(vs), 1097(s), 944(w), 801(s), 741(w), 655(vs), 580(s).15
Crystal structure determination and refinement
Suitable crystals were selected and mounted on a Bruker SMART 1000 diffractometer with graphite monochromated Mo Kα radiation (λ = 0.71073 Å) at 113 K for 1, 2 and 3. Lorentz-polarization correction was applied to the data. The structures were solved by direct methods using SHELXS-2014 16 program and refinements on F2 were performed using SHELXL-2014 by full-matrix least-squares procedure with anisotropic thermal parameters for all non-hydrogen atoms. Hydrogen atoms were added theoretically and refined with riding model position parameters and isotropically refined crystal data and details of structural determination and refinement are summarized in Table 1 and the selected bond lengths and angles have been provided in the ESI, Tables S1–S3.†
Table 1 Crystallographic data and structure refinement for complexes 1, 2, 3
|
1 |
2 |
3 |
Chemical formula |
C23H15DyF18N4O6 |
C23H15TbF18N4O6 |
C23H15HoF18N4O6 |
Formula weight |
947.89 |
944.32 |
950.32 |
Temperature/K |
113 |
113 |
113 |
Crystal system |
Monoclinic |
Monoclinic |
Monoclinic |
Space group |
C2/c |
C2/c |
C2/c |
a/Å |
20.103(4) |
20.173(4) |
20.079(7) |
b/Å |
10.649(2) |
10.666(2) |
10.639(2) |
c/Å |
18.062(4) |
18.074(4) |
18.035(9) |
α/° |
90 |
90 |
90 |
β/° |
113.88(3) |
113.82(3) |
113.89(3) |
γ/° |
90 |
90 |
90 |
V/Å−3 |
3535.7(15) |
3557.6(15) |
3523(2) |
Z |
4 |
4 |
4 |
ρ/(g cm−3) |
1.781 |
1.763 |
1.792 |
μ/(mm−1) |
2.248 |
2.122 |
2.382 |
F(000) |
1828 |
1824 |
1832 |
Reflections collected |
14 331 |
13 681 |
14 168 |
Unique/parameters |
3151/322 |
3136/322 |
3113/322 |
R(int) |
0.0705 |
0.0547 |
0.0705 |
Max./min. transmission |
0.698/0.0.644 |
0.661/0.702 |
0.717/0.751 |
Goodness-of-fit on F2 |
1.014 |
1.049 |
1.039 |
R1, wR2 [I > 2σ(I)] |
0.0434/0.0987 |
0.0428/0.1061 |
0.0423/0.1035 |
R1, wR2 (all data) |
0.0500/0.1032 |
0.0469/0.1108 |
0.0468/0.1081 |
Results and discussion
Crystal structures
Compared with the previously reported [Dy(hfac)3·(pz)2], complex 1 has a similar mononuclear structure and is synthesized by substituting two H2O molecules by two 1-methylimidazole ligands in a Dy(hfac)3·2H2O salt. It crystalizes as a symmetrical mononuclear compound [Dy(hfac)3·(1-MeIm)2] in monoclinic C2/c space group (Fig. 1). The DyIII core is also eight-coordinated in a [DyO6N2] environment with Dy–O bond lengths in the range of 2.346(4)–2.358(4) Å, which is shorter than that of Dy–N (2.467(4) Å). The angles of O–Dy–O is ranging from 71.2 to 145.1°, the bond angles O–Dy–N is ranging from 73.6 to 143.8°, and the angle of N(1)1–Dy(1)–N(1) is 141.7°. The [DyO6N2] geometry is studied using the SHAPE software17 to obtain the minimal deviation parameter S of 0.396 for D4d symmetry as shown in Table 2, rather than D2d as in [Dy(hfac)3·(pz)2]. The shortest intermolecular Dy⋯Dy separation distance of 9.098(8) Å is larger than that of [Dy(hfac)3(pz)2] (8.87 Å). The packing diagram of the compound 1 is given in the ESI, Fig. S1,† which shows no inter- and intramolecular π–π interaction. Hydrogen bonding interactions between the molecules were not found in the complex 1.
 |
| Fig. 1 Perspective view of complex [Dy(hfac)3·(1-MeIm)2] (H atoms were all omitted for clarity, left) and the coordination polyhedron of the DyIII ions (right). | |
Table 2 The minimal deviation parameter S for complex 1, 2, 3 of LnIII ion geometry analysis by using the SHAPE software
LnIII |
D2d-DD |
C2v-TP |
D4d-SAP |
DyIII |
1.397 |
2.734 |
0.396 |
TbIII |
1.457 |
1.439 |
1.971 |
HoIII |
1.959 |
0.359 |
0.380 |
The structures of complex 2 and 3 consist of similar mononuclear units as those in complex 1 with only small differences in the bond lengths and angles. Their molecular structures, D4d-symmetry polyhedrons and packing diagrams are given in the ESI, Fig. S2–S5.†
Luminescence properties
Fig. 2 shows the emission spectrum of the DyIII and TbIII complexes recorded at room temperature under irradiation of UV light at 350 and 347 nm, respectively. Well-resolved multiline emissions of DyIII ion were observed around 480, 573 and 661 nm, which can be attributed to 4F9/2 → 6HJ with J = 15/2, 13/2 and 11/2, respectively (Fig. 2a).13b,d Four characteristic peaks of the TbIII complex shown in Fig. 2b are attributed to the metal-centered 5D4 excited state to the 7FJ ground-state multiplet. Maximum emission peaks at 489, 544, 587 and 621 nm, were observed for the J = 3, 4, 5 and 6 transitions, respectively.13k
 |
| Fig. 2 The luminescence spectra of 1 (a) and 2 (b) in the solid state at room temperature. | |
The decay lifetime curves reveal single-exponential mode with the lifetimes of τ1 = 0.4549 μs (100%) for 1, while double-exponential mode was observed for 2, giving lifetime values (17.06 μs (31.66%) and 237.0 μs (68.34%)), as determined by monitoring the 5D4 → 7F5 transition (Fig. S6†). Moreover, for complex 2, the fluorescence lifetime is a little higher than that of [Tb(hfac)3(pz)2]10 (77 μs), showing that the ancillary ligand of 1-methylimidazole has an antenna effect on the luminescence of the TbIII complex, with the adding of methyl to give more electron density to the aromatic ring.
Magnetic properties
Static magnetic properties of 1. Magnetic susceptibility measurement of 1 was carried out under an external direct current (dc) magnetic field of 0.1 T at 2–300 K. At 300 K, the χMT value of 14.60 cm3 K mol−1 were obtained, which is slightly higher than the expected value of 14.17 cm3 K mol−1 for one uncoupled DyIII cation (DyIII:S = 5/2, L = 5, 6H15/2, g = 4/3). The χMT value keeps roughly constant from 300 K to 50 K but decreases more and more rapidly as temperature drops from 50 K and reaches a minimum value of 13.13 cm3 K mol−1 at 2 K (Fig. 3), which probably results from the progressive depopulation of the DyIII stark sublevels and/or intermolecular interactions. Reduced magnetization data for complex 1 was determined at different temperatures (inset of Fig. 3). The magnetization values show poor superposition even at the highest available fields. Moreover, the maximum value of 8.86 Nβ is considerably lower than the expected value, which indicates the presence of a significant magnetic anisotropy and/or low-lying excited states.3i,9h,18
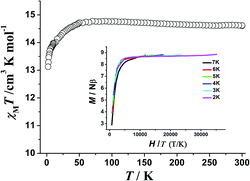 |
| Fig. 3 Temperature dependency of χMT in the range of 2–300 K in 0.1 T for 1. Inset: M vs. H/T plot measured in different fields below 7 T. | |
Dynamic magnetic properties of 1. The frequency- and temperature-dependent alternating current (ac) susceptibilities for 1 were obtained under zero-dc field (Fig. 4 and S7†). With the increasing temperature the peaks of the χ′′M data move to higher frequency, which reveals the typical features associated with the SMM behavior. To further ensure the complex 1 is a SMM but not a spin-glass (0.01 < ϕ < 0.08), the indicative parameter of spin disorder ϕ of 0.21 was obtained based on the data of the temperature dependencies of χ′M, using Mydosh's formula in eqn (1).19 |
ϕ = (ΔTp/Tp)/Δ(log ω)
| (1) |
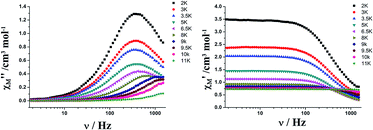 |
| Fig. 4 Frequency dependence of the in-phase (left) and out-of-phase (right) ac susceptibility of 1 below 11 K, under zero-dc field. | |
The magnetization relaxation time in the form of ln
τ derived from the frequency-dependence measurements is plotted as a function of 1/T between 2 and 11 K (Fig. 5). Above 7.7 K, the relaxation follows a thermally activated process permitting the estimation of an energy barrier of 42.54 K with a pre-exponential factor (τ0) of 1.13 × 10−6 s based on the Arrhenius law, which is consistent with the expected τ0 of 10−6 to 10−11 for a SMM.3g–i At lower temperatures a gradual crossover to a temperature-independent regime is observed and below about 3.5 K, a dominant quantum regime with a τ value of 0.0004 s explains the absence of M vs. H hysteresis effect at 2 K (Fig. S8†), which is likely due to the hyperfine coupling and dipolar spin–spin interactions of the metal cations. As a result, fast quantum tunneling of magnetization was observed.
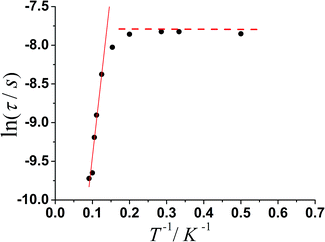 |
| Fig. 5 Magnetization relaxation time, ln τ vs. T−1 plot for 1 under zero-dc field. The solid line is with the fitting to the Arrhenius law. | |
Cole–cole plots (Fig. 6) in the temperature range of 2–10 K with the form of χ′′M vs. χ′M, which can be well fitted by the generalized Debye model,20a,b exhibit good semicircle shapes and small distribution parameters α have been obtained (Table S4†). All of these magnetic parameters reveal a single relaxation process in complex 1.20c,d All the magnetic analyses as discussed above support the SMM nature of the mononuclear Dy complex 1. Compared with [Dy(hfac)3(pz)2]10, the use of 1-methylimidazole instead of pyrazole to coordinate with Dy(hfac)3 units leads to the improvement of the ligand field (from D2d for lanthanide ion to D4d), and further enlarges the energy barrier of Dy ion from 28.32 to 42.54 K. The higher symmetric coordination environments (as an example (D4d)3a,g,21) reduce the admixing of states on opposite sides of the barrier and successfully suppress QTM, which proved the conclusion already obtained by Tong et al. that SIMs with the five symmetries (C∞v, D∞h, S8 (I4), D5h, and D4d) usually showing higher energy barriers.3a,c,e,h,22 And the intermolecular Dy–Dy distance changes from 8.87 Å to 9.10 Å, also decreases the intermolecular Dy ions dipolar coupling then minimize the QTM to some extent.
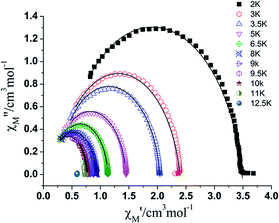 |
| Fig. 6 Cole–cole plots measured at 2–11 K in zero-dc field. | |
Static magnetic properties for 2 and 3. As depicted in Fig. 7 and 8, the temperature dependence of magnetic susceptibilities for 2 and 3 are also studied over the range from 300 to 2 K. At room temperature, the χMT values are 11.85 and 13.85 cm3 K mol−1 for 2 and 3, respectively. The value of complex 2 is in good agreement with the expected value of 11.81 for one uncoupled TbIII ion with 7F6 ground state, but 3 is slightly lower than 14.06 cm3 K mol−1 for one uncoupled HoIII ion with 5I8 ground state. Upon cooling, the χMT value of 2 goes down slowly and reaches a minimum of 11.57 cm3 K mol−1 at 77 K, then gradually rises to a maximum of 11.75 at 53.4 K and further declines more and more rapidly to 10.13 cm3 K mol−1 at 2 K. However, the χMT value for 3 gradually deceases over the temperature range 120–300 K and further goes down dramatically to reach a minimum value of 5.89 cm3 K mol−1 at 2 K. To obtain a rough quantitative estimation of the magnetic interaction between paramagnetic species,23 the magnetic data of 2 and 3 can be analyzed by the approximate model,11 which is given in the ESI, eqn (2)–(4)† using an anisotropic exchange Hamiltonian H = ΔJz2. The intermolecular interactions were considered by the term zj′.
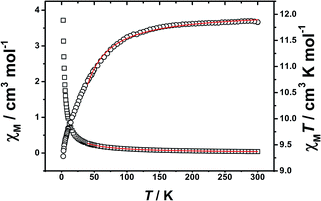 |
| Fig. 7 Temperature dependence of χM and χMT product in the range of 2–300 K in 0.1 T for 2. | |
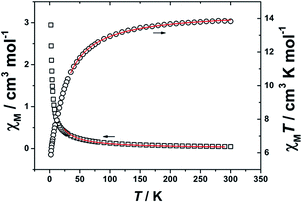 |
| Fig. 8 Temperature dependence of χM and χMT product in the range of 2–300 K in 0.1 T for 3. | |
The best fitting results yielded, zj′ = 0.106 cm−1 (Δ = 0.526 cm−1) with the g-factor of 1.516 for Tb complex, and zj′ = −0.230 cm−1 (Δ = −0.154 cm−1) with the g-factor of 1.267 for Ho complex. The small value of zj′ is indicative of the very weak magnetic interaction between LnIII ions in 2 and 3.
Variable temperature ac susceptibility data for complexes 2 and 3 are also collected, which are given in the ESI, Fig. S9 and S10.† No frequency-dependent signals of imaginary component χ′′M have been observed for two complexes even at 2 K. That might be due to the Tb and Ho ions spin states are more sensitive to crystal-field, which usually give Tb and Ho ions very small J grand state and/or QTM, to induce their complexes have too small energy barrier to prevent the inversion of the spin.
Conclusion
Using 1-methylimidazole ligand in association with DyIII, we successfully synthesized a symmetrical mononuclear complex of [Dy(hfac)3·(1-MeIm)2], which exhibits slow relaxation of magnetization considered as one of the examples of a SMM as well as the DyIII characteristic emission at room temperature. The change of ligand field symmetry from D2d for lanthanide ion to D4d resulted in a larger energy barrier of Dy ion from 28.32 to 42.54 K in complex 1 where 1-methylimidazole was coordinated, as compared to [Dy(hfac)3(pz)2]. The higher symmetry coordination environments reduce the admixing of states on opposite sides of the barrier, which controls QTM by tuning the local symmetry of the metal centers. The expansion of intermolecular Dy–Dy distance from 8.87 Å to 9.10 Å as we expected probably decreases the intermolecular Dy ions dipolar coupling and suppresses QTM effectively as well. It is obvious that the variation in ligands leads to the change of ligand field and magnetic interaction, which affect the magnetic properties of lanthanide complexes. This proves to be a powerful approach to understand how ligand field symmetry and magnetic interactions affect the SMM behaviors of lanthanide-based systems.
Acknowledgements
This work was supported by the National Natural Science Foundation of China 21471084, 21471083, 21371104. National Undergraduates Innovating Experimentation Project 201410055097.
Notes and references
-
(a) R. J. Blagg, L. Ungur, F. Tuna, J. Speak, P. Comar, D. Collison, W. Wernsdorfer, E. J. L. Mclnnes, L. F. Chibotaru and R. E. P. Winpenny, Nat. Chem., 2013, 5, 673–678 CrossRef CAS PubMed;
(b) L. Bogani and W. Wernsdorfer, Nat. Mater., 2008, 7, 179–186 CrossRef CAS PubMed;
(c) R. A. Layfield, Organometallics, 2014, 33, 1084–1099 CrossRef CAS;
(d) A. Cornia, M. Mannini, P. Sainctavit and R. Sessoli, Chem. Soc. Rev., 2011, 40, 3076–3091 RSC;
(e) M. Urdampilleta, S. Klyatskaya, J.-P. Cleuziou, M. Ruben and W. Wernsdorfer, Nat. Mater., 2011, 10, 502–506 CrossRef CAS PubMed;
(f) D. N. Woodruff, R. E. P. Winpenny and R. A. Layfield, Chem. Rev., 2013, 113, 5110–5148 CrossRef CAS PubMed.
-
(a) J. L. Liu, J. Y. Wu, G. Z. Huang, Y. C. Chen, J. H. Jia, L. Ungur, L. F. Chibotaru, X. M. Chen and M. L. Tong, Sci. Rep., 2015, 5, 16621 CrossRef CAS PubMed;
(b) R. J. Blagg, L. Ungur, F. Tuna, J. Speak, P. Comar, D. Collison, W. Wernsdorfer, E. J. L. McInnes, L. F. Chibotaru and R. E. P. Winpenny, Nat. Chem., 2013, 5, 673–678 CrossRef CAS PubMed;
(c) S. D. Jiang, B. W. Wang, H. L. Sun, Z. M. Wang and S. Gao, J. Am. Chem. Soc., 2011, 133, 4730–4733 CrossRef CAS PubMed;
(d) J. L. Liu, Y. C. Chen, Y. Z. Zheng, W. Q. Lin, L. Ungur, W. Wernsdorfer, L. F. Chibotaru and M. L. Tong, Chem. Sci., 2013, 4, 3310–3316 RSC;
(e) J. Liu, Y. C. Chen, J. L. Liu, V. Vieru, L. Ungur, J. H. Jia, L. F. Chibotaru, Y. Lan, W. Wernsdorfer, S. Gao, X. M. Chen and M. L. Tong, J. Am. Chem. Soc., 2016, 138, 5441–5450 CrossRef CAS PubMed.
-
(a) N. Ishikawa, M. Sugita, T. Ishikawa, S.-y. Koshihara and Y. Kaizu, J. Am. Chem. Soc., 2003, 125, 8694–8695 CrossRef CAS PubMed;
(b) N. Ishikawa, M. Sugita and W. Wernsdorfer, Angew. Chem., Int. Ed., 2005, 44, 2931–2935 CrossRef CAS PubMed;
(c) M. A. AlDamen, J. M. Clemente-Juan, E. Coronado, C. Martí-Gastaldo and A. Gaita-Ariño, J. Am. Chem. Soc., 2008, 130, 8874–8875 CrossRef CAS PubMed;
(d) F. Branzoli, P. Carretta, M. Filibian, G. Zoppellaro, M. J. Graf, J. R. Galan-Mascaros, O. Fuhr, S. Brink and M. J. Ruben, J. Am. Chem. Soc., 2009, 131, 4387–4396 CrossRef CAS PubMed;
(e) Y.-C. Chen, J.-L. Liu, L. Ungur, J. Liu, Q.-W. Li, L.-F. Wang, Z.-P. Ni, L. F. Chibotaru, X.-M. Chen and M.-L. Tong, J. Am. Chem. Soc., 2016, 138, 2829–2837 CrossRef CAS PubMed;
(f) M. Gonidec, F. Luis, À. Vílchez, J. Esquena, D. B. Amabilino and J. Veciana, Angew. Chem., Int. Ed., 2010, 49, 1623–1626 CrossRef CAS PubMed;
(g) G. J. Chen, C. Y. Gao, J. L. Tian, J. K. Tang, W. Gu, X. Liu, S. P. Yan, D. Z. Liao and P. Cheng, Dalton Trans., 2011, 40, 5579–5583 RSC;
(h) S. D. Jiang, B. W. Wang, G. Su, Z. M. Wang and S. Gao, Angew. Chem., Int. Ed., 2010, 49, 7448–7451 CrossRef CAS PubMed;
(i) K. Bernot, J. Luzon, L. Bogani, M. Etienne, C. Sangregorio, M. Shanmugam, A. Caneschi, R. Sessoli and D. Gatteschi, J. Am. Chem. Soc., 2009, 131, 5573–5579 CrossRef CAS PubMed.
-
(a) J. Long, F. Habib, P. H. Lin, I. Korobkov, G. Enright, L. Ungur, W. Wernsdorfer, L. F. Chibotaru and M. Murugesu, J. Am. Chem. Soc., 2011, 133, 5319–5328 CrossRef CAS PubMed;
(b) C. Wang, S. Y. Lin, J. Wu, S. W. Yuan and J. Tang, Dalton Trans., 2015, 44, 4648–4654 RSC;
(c) L. F. Chibotaru, L. Ungur and A. Soncini, Angew. Chem., Int. Ed., 2008, 47, 4126–4129 CrossRef CAS PubMed;
(d) J. Luzon, K. Bernot, I. J. Hewitt, C. E. Anson, A. K. Powell and R. Sessoli, Phys. Rev. Lett., 2008, 100, 247205 CrossRef PubMed;
(e) F. Habib, P. H. Lin, J. Long, I. Korobkov, W. Wernsdorfer and M. Murugesu, J. Am. Chem. Soc., 2011, 133, 8830–8833 CrossRef CAS PubMed.
-
(a) J. K. Tang, I. Hewitt, N. T. Madhu, G. Chastanet, W. Wernsdorfer, C. E. Anson, C. Benelli, R. Sessoli and A. K. Powell, Angew. Chem., Int. Ed., 2006, 45, 1729–1733 CrossRef CAS PubMed;
(b) S. Xue, X. H. Chen, L. Zhao, Y. N. Guo and J. K. Tang, Inorg. Chem., 2012, 51, 13264–13270 CrossRef CAS PubMed;
(c) I. J. Hewitt, Y. Lan, C. E. Anson, J. Luzon, R. Sessoli and A. K. Powell, Chem. Commun., 2009, 6765–6767 RSC.
-
(a) Y. N. Guo, G. F. Xu, P. Gamez, L. Zhao, S. Y. Lin, R. Deng, J. K. Tang and H. J. Zhang, J. Am. Chem. Soc., 2010, 132, 8538–8539 CrossRef CAS PubMed;
(b) B. H. Koo, K. S. Lim, D. W. Ryu, W. R. Lee, E. K. Koh and C. S. Hong, Chem. Commun., 2012, 48, 2519–2521 RSC;
(c) P. H. Lin, T. J. Burchell, L. Ungur, L. F. Chibotaru, W. Wernsdorfer and M. Murugesu, Angew. Chem., Int. Ed., 2009, 48, 9489–9492 CrossRef CAS PubMed;
(d) P. H. Lin, I. Korobkov, W. Wernsdorfer, L. Ungur, L. F. Chibotaru and M. Murugesu, Eur. J. Inorg. Chem., 2011, 1535–1539 CrossRef CAS;
(e) M. U. Anwar, L. K. Thompson, L. N. Dawe, F. Habib and M. Murugesu, Chem. Commun., 2012, 48, 4576–4578 RSC;
(f) V. Chandrasekhar, S. Das, A. Dey, S. Hossain and J.-P. Sutter, Inorg. Chem., 2013, 52, 11956–11965 CrossRef CAS PubMed.
-
(a) R. J. Blagg, C. A. Muryn, E. J. L. McInnes, F. Tuna and R. E. P. Winpenny, Angew. Chem., Int. Ed., 2011, 50, 6530–6533 CrossRef CAS PubMed;
(b) R. J. Blagg, F. Tuna, E. J. L. McInnes and R. E. P. Winpenny, Chem. Commun., 2011, 47, 10587–10589 RSC.
-
(a) Y. N. Guo, X. H. Chen, S. Xue and J. K. Tang, Inorg. Chem., 2012, 51, 4035–4042 CrossRef CAS PubMed;
(b) H. Tian, M. Wang, L. Zhao, Y. N. Guo, Y. Guo, J. K. Tang and Z. Liu, Chem.–Eur. J., 2012, 18, 442–445 CrossRef CAS PubMed;
(c) H. Tian, Y. N. Guo, L. Zhao, J. K. Tang and Z. Liu, Inorg. Chem., 2011, 50, 8688–8690 CrossRef CAS PubMed;
(d) J. W. Sharples, Y. Zheng, F. Tuna, E. J. L. McInnes and D. Collison, Chem. Commun., 2011, 47, 7650–7652 RSC;
(e) H. Tian, L. Zhao, Y. N. Guo, Y. Guo, J. K. Tang and Z. Liu, Chem. Commun., 2012, 48, 708–710 RSC;
(f) Y. L. Miao, J. L. Liu, J. D. Leng, Z. J. Lina and M. L. Tong, CrystEngComm, 2011, 13, 3345–3348 RSC;
(g) Y. Z. Zheng, Y. Lan, W. Wernsdorfer, C. E. Anson and A. K. Powell, Chem.–Eur. J., 2009, 15, 12566–15270 CrossRef CAS PubMed;
(h) I. J. Hewitt, J. K. Tang, N. T. Madhu, C. E. Anson, Y. Lan, J. Luzon, M. Etienne, R. Sessoli and A. K. Powell, Angew. Chem., Int. Ed., 2010, 49, 6352–6356 CrossRef CAS PubMed.
-
(a) G. Cucinotta, M. Perfetti, J. Luzon, M. Etienne, P.-E. Car, A. Caneschi, G. Calvez, K. Bernot and R. Sessoli, Angew. Chem., Int. Ed., 2012, 51, 1606–1610 CrossRef CAS PubMed;
(b) M. J. Martínez-Pérez, S. Cardona-Serra, C. Schlegel, F. Moro, P. J. Alonso, H. Prima-García, J. M. Clemente-Juan, M. Evangelisti, A. Gaita-Ariño, J. Sesé, J. van Slageren, E. Coronado and F. Luis, Phys. Rev. Lett., 2012, 108, 247213 CrossRef PubMed;
(c) F. Habib, J. Long, P. H. Lin, I. Korobkov, L. Ungur, W. Wernsdorfer, L. F. Chibotaru and M. Murugesu, Chem. Sci., 2012, 3, 2158–2164 RSC;
(d) U. J. Williams, B. D. Mahoney, P. T. DeGregorio, P. J. Carroll, E. Nakamaru-Ogiso, J. M. Kikkawa and E. J. Schelter, Chem. Commun., 2012, 48, 5593–5595 RSC;
(e) S. K. Langley, N. F. Chilton, B. Moubaraki and K. S. Murray, Chem. Commun., 2013, 49, 6965–6967 RSC;
(f) X. Yi, K. Bernot, F. Pointillart, G. Poneti, G. Calvez, C. Daiguebonne, O. Guillou and R. Sessoli, Chem.–Eur. J., 2012, 18, 11379–11387 CrossRef CAS PubMed;
(g) F. Pointillart, Y. Le Gal, S. Golhen, O. Cador and L. Ouahab, Chem.–Eur. J., 2011, 17, 10397–10404 CrossRef CAS PubMed;
(h) P. H. Lin, T. J. Burchell, R. Clérac and M. Murugesu, Angew. Chem., Int. Ed., 2008, 47, 8848–8851 CrossRef CAS PubMed;
(i) K. Bernot, F. Pointillart, P. Rosa, M. Etienne, R. Sessoli and D. Gatteschi, Chem. Commun., 2010, 46, 6458–6460 RSC;
(j) F. Pointillart, K. Bernot, G. Poneti and R. Sessoli, Inorg. Chem., 2012, 51, 12218–12229 CrossRef CAS PubMed.
- Y. L. Wang, B. Gu, Y. Ma, C. Xing, Q. L. Wang, L. C. Li, P. Cheng and D. Z. Liao, CrystEngComm, 2014, 16, 2283–2289 RSC.
- Y. Liao, W. W. Shum and J. S. Miller, J. Am. Chem. Soc., 2002, 124, 9336–9337 CrossRef CAS PubMed.
- C. Aronica, G. Pilet, G. Chastanet, W. Wernsdorfer, J. F. Jacquot and D. Luneau, Angew. Chem., Int. Ed., 2006, 45, 4659–4662 CrossRef CAS PubMed.
-
(a) J. Ruiz, A. J. Mota, A. Rodriguez-Dieguez, S. Titos, J. M. Herrera, E. Ruiz, E. Cremades, J. P. Costes and E. Colacio, Chem. Commun., 2012, 48, 7916–7918 RSC;
(b) C. E. Burrow, T. J. Burchell, P. H. Lin, F. Habib, W. Wernsdorfer, R. Clérac and M. Murugesu, Inorg. Chem., 2009, 48, 8051–8053 CrossRef CAS PubMed;
(c) J. C. G. Bünzli and C. Piguet, Chem. Soc. Rev., 2005, 34, 1048–1077 RSC;
(d) C. Freund, W. Porzio, U. Giovanella, G. Vignali, M. Pasini and S. Destri, Inorg. Chem., 2011, 50, 5417–5429 CrossRef CAS PubMed;
(e) D. B. A. Raj, B. Francis, M. L. P. Reddy, R. R. Butorac, V. M. Lynch and A. H. Cowley, Inorg. Chem., 2010, 49, 9055–9063 CrossRef CAS PubMed;
(f) H. Wang, D. Zhang, Z. H. Ni, X. Li, L. Tian and J. Jiang, Inorg. Chem., 2009, 48, 5946–5946 CrossRef CAS PubMed;
(g) J. An, C. M. Shade, D. A. Chengelis-Czegan, S. Petoud and N. L. Rosi, J. Am. Chem. Soc., 2011, 133, 1220–1223 CrossRef CAS PubMed;
(h) K. A. White, D. A. Chengelis, K. A. Gogick, J. Stehman, N. L. Rosi and S. Petoud, J. Am. Chem. Soc., 2009, 131, 18069–18071 CrossRef CAS PubMed;
(i) S. V. Eliseevaa and J. C. G. Bünzli, Chem. Soc. Rev., 2010, 39, 189–227 RSC;
(j) S. Swavey and R. Swavey, Coord. Chem. Rev., 2009, 253, 2627–2638 CrossRef CAS;
(k) K. Binnemans, L. Malykhina, V. S. Mironov, W. Haase, K. Driesen, R. Van Deun, L. Fluyt, C. Görller-Walrand and Y. G. Galyametdinov, ChemPhysChem, 2001, 2, 680–683 CrossRef CAS PubMed;
(l) J. P. Leonard, P. Jensen, T. McCabe, J. E. O'Brien, R. D. Peacock, P. E. Kruger and T. Gunnlaugsson, J. Am. Chem. Soc., 2007, 129, 10986–10987 CrossRef CAS PubMed.
-
(a) Theory and Applications of Molecular Paramagnetism, ed. E. A. Boudreaux and L. N. Mulay, Wiley-Interscience, New York, 1976 Search PubMed;
(b) G. A. Bain and J. F. Berry, J. Chem. Educ., 2008, 85, 532–536 CrossRef CAS.
- Z. Ahmed, W. Ahmed Dar and K. Iftikhar, Inorg. Chim. Acta, 2012, 392, 446–453 CrossRef CAS.
-
(a) G. M. Sheldrick, SHELXS-2014, Program for structure solution, Universität of Göttingen, Germany Search PubMed;
(b) G. M. Sheldrick, SHELXL-2014, Program for structure refinement, Universität of Göttingen, Göttingen, Germany, 2014 Search PubMed.
-
(a) SHAPE, version 2.0; continuous shape measures calculation; Electronic Structure Group, Universiat de Barcelona, Barcelona, Spain, 2010 Search PubMed;
(b) H. Zabrodsky, S. Peleg and D. Avnir, J. Am. Chem. Soc., 1992, 114, 7843–7851 CrossRef CAS;
(c) M. Pinsky and D. Avnir, Inorg. Chem., 1998, 37, 5575–5582 CrossRef CAS PubMed.
- S. Kanegawa, M. Maeyama, M. Nakano and N. Koga, J. Am. Chem. Soc., 2008, 130, 3079–3094 CrossRef CAS PubMed.
-
(a) D. Gatteschi, R. Sessoli and J. Villain, Molecular Nanomagnets, Oxford University Press, New York, 2006, pp. 69–75 Search PubMed;
(b) J. A. Mydosh, Spin Glasses: An Experimental Introduction, Taylor&Francis, London, 1993 Search PubMed.
-
(a) K. S. Cole and R. H. Cole, J. Chem. Phys., 1941, 9, 341–351 CrossRef CAS;
(b) M. Hagiwara, J. Magn. Magn. Mater., 1998, 89, 177–181 Search PubMed;
(c) T. Kajiwara, M. Nakano, K. Takahashi, S. Takaishi and M. Yamashita, Chem.–Eur. J., 2011, 17, 196–205 CrossRef CAS PubMed;
(d) S. Dhers, J. P. Costes, P. Guionneau, C. Paulsen, L. Vendier and J. P. Sutter, Chem. Commun., 2015, 51, 7875–7878 RSC.
-
(a) X.-J. Zhang, K. Liu, Y.-m. Bing, N. Xu, W. Shi and P. Cheng, Dalton Trans., 2015, 44, 757–7760 Search PubMed;
(b) T. Li, S. Y. Zhou, X. Li, L. Tian, D. Z. Liao, Z. Y. Liu and J. H. Guo, Dalton Trans., 2016, 45, 3863–3873 RSC;
(c) M. A. Hareri, E. L. Gavey, J. Regier, Z. R. Ali, L. D. Carlos, R. A. S. Ferreira and M. Pilkington, Chem. Commun., 2016, 52, 11335–11338 RSC.
-
(a) J. M. Zadrozny, D. J. Xiao, M. Atanasov, G. J. Long, F. Grandjean, F. Neese and J. R. Long, Nat. Chem., 2013, 5, 577–581 CrossRef CAS PubMed;
(b) N. Ishikawa, M. Sugita, T. Ishikawa, S. Koshihara and Y. Kaizu, J. Phys. Chem. B, 2004, 108, 11265–11271 CrossRef CAS;
(c) C. R. Ganivet, B. Ballesteros, G. de la Torre, J. M. Clemente-Juan, E. Coronado and T. Torres, Chem.–Eur. J., 2013, 19, 1457–1465 CrossRef CAS PubMed.
-
(a) I. A. Kahwa, J. Selbin, C. J. O'Connor, J. W. Foise and G. L. McPherson, Inorg. Chim. Acta, 1988, 148, 265–272 CrossRef CAS;
(b) J. X. Xu, Y. Ma, D. Z. Liao, G. F. Xu, J. K. Tang, C. Wang, N. Zhou, S. P. Yan, P. Cheng and L. C. Li, Inorg. Chem., 2009, 48, 8890–8896 CrossRef CAS PubMed;
(c) Y. L. Wang, N. Zhou, Y. Ma, Z. X. Qin, Q. L. Wang, L. C. Li, P. Cheng and D. Z. Liao, CrystEngComm, 2012, 14, 235–239 RSC;
(d) Y. Ouyang, W. Zhang, N. Xu, G. F. Xu, D. Z. Liao, K. Yoshimura, S. P. Yan and P. Cheng, Inorg. Chem., 2007, 46, 8454–8456 CrossRef CAS PubMed;
(e) Y. L. Wang, Y. Y. Gao, Y. Ma, Q. L. Wang, L. C. Li and D. Z. Liao, CrystEngComm, 2012, 14, 4706–4712 RSC.
Footnote |
† Electronic supplementary information (ESI) available: X-ray crystallographic data for complexes 1–3 in CIF format. Selected bond lengths and angles, figures of crystal structures, luminescence measurements, and magnetic measurements. CCDC 800739, 798521 and 1515832. For ESI and crystallographic data in CIF or other electronic format see DOI: 10.1039/c6ra25038f |
|
This journal is © The Royal Society of Chemistry 2017 |