DOI:
10.1039/C6RA24769E
(Paper)
RSC Adv., 2017,
7, 1833-1840
Amphipathic monolith-supported palladium catalysts for chemoselective hydrogenation and cross-coupling reactions†
Received
5th October 2016
, Accepted 10th December 2016
First published on 12th January 2017
Abstract
A palladium catalyst immobilized on an amphipathic and monolithic polystyrene–divinylbenzene polymer bearing strongly acidic cation exchange functions (sulfonic acid moieties) (Pd/CM) was developed. It was used as a catalyst for hydrogenation and ligand-free cross-coupling reactions, such as the Suzuki–Miyaura, Mizoroki–Heck, and copper- and amine-free Sonogashira-type reactions, together with a palladium catalyst supported on monolithic polymer (Pd/AM) bearing basic anion exchange functions (ammonium salt moieties), which has been in practical use for the decomposition of hydrogen peroxide produced as a byproduct during the manufacture of ultrapure water. While the Pd/CM was highly active as a catalyst for the hydrogenation and a variety of reducible functional groups could be reduced, the use of Pd/AM led to a unique chemoselective hydrogenation. Aromatic carbonyl groups were tolerant under the Pd/AM-catalyzed hydrogenation conditions, although benzyl esters, benzyl ethers, and N-Cbz groups could be smoothly hydrocracked. The cross-coupling reactions readily proceeded using either catalyst. The palladium leaching from the Pd/CM into the reaction media was never observed during the Sonogashira-type reaction, which was hardly achieved by other palladium-supported heterogeneous catalysts due to the good affinity of the palladium species with alkynes.
Introduction
The development of heterogeneous palladium catalysts is an important subject in organic chemistry from environmental and economical viewpoints based on their recovery, reuse, and non-metal residual property.1 A wide variety of organic polymers possessing some functional groups within the molecule, which could act as ligands toward palladium species, has been used as supports for the heterogeneous palladium catalysts, especially for cross-coupling reactions.2,3 The variation in the supports of palladium catalysts is also known to affect the catalyst activity for hydrogenation,4 and we have developed a variety of heterogeneous palladium catalysts for chemoselective hydrogenation, such as palladium fibroin (Pd/Fib),5 palladium on synthetic adsorbent (Pd/HP20),2b,c palladium on molecular sieves (Pd/MS),6 palladium on boron nitride (Pd/BN),7 palladium on chelate resin (Pd/CR11 and Pd/CR20),8 and palladium on ceramic (Pd/ceramic).9
Monolithic polymers are supposed to be good supports for the palladium catalysts based on the effective incorporation of substrates into the porous supports, and such catalysts are expected to be used for flow reactions due to the excellent permeability of the reaction solution without resistance to the fluid flow. Palladium catalysts immobilized on polystyrene-based monolithic polymers bearing quaternary ammonium salts as the anion exchange functions have been used under flow conditions for the cross-coupling reactions, such as the Suzuki–Miyaura, Mizoroki–Heck, and Sonogashira-type reactions, and transfer hydrogenation in the absence of hydrogen gas.10 Other types of palladium catalysts supported on monolithic polymers derived from ferritin bionanoparticles,11 N-aminoethylamidine-bound polyacrylonitrile,12 and Shiff base-bound acrylate13 were also reported as effective catalysts for the Suzuki–Miyaura reaction11–13 under batch conditions and flow hydrogenation.11
A polystyrene-based monolithic polymer bearing quaternary ammonium moieties (AM) has been in practical use as a support for the palladium catalyst (Pd/AM), which decomposes hydrogen peroxide produced as a byproduct during the UV irradiation process to degrade contaminated trace organic substances for manufacturing ultrapure water.14 The polystyrene-based monolithic polymer bearing sulfonic acid moieties as cationic exchange functions (CM) are also manufactured.15 We anticipated that the hydrophilic sulfonic acid moieties would facilitate the incorporation of palladium species on/in the porous monolithic polymer in analogy with Pd/AM. Such ammonium and sulfonic acid moieties were expected to function as ligands for cross-coupling reactions and also as catalyst poisons for chemoselective hydrogenation based on the appropriate coordination to palladium metals.4a Furthermore, the amphipathic and porous nature of the supports would provide a broad substrate scope due to an easy access of both hydrophobic and hydrophilic substrates to the catalyst metals.3e,16 In this paper, we demonstrate the development of a novel palladium catalyst supported on CM (Pd/CM) as well as the application of both Pd/AM and Pd/CM to several organic reactions; i.e., hydrogenation and cross-coupling reactions under batch conditions.
Results and discussion
Preparation of palladium on monolith-SO3H (Pd/CM)
The unfunctionalized monolithic polymer was first prepared through the two successive polymerization processes from styrene and divinylbenzene using sorbitan fatty acid ester as an emulsifier, radical initiators, such as 2,2′-azobis(isobutyronitrile), and water according to a previously reported method (Fig. 1).14 Subsequently, the sulfonic acid function was introduced to the resulting plain monolithic polymer by the general method for the preparation of a strongly acidic cation exchange resin using chlorosulfuric acid.15 The colorless monolith-SO3H polymer (CM) was cut into 1 mm cubes using a ceramic knife, then immersed in a reddish Pd(OAc)2 solution of MeOH. After the mixture was stirred at room temperature under an Ar atmosphere for 24 h, the solution became colorless, and the colorless CM was time-dependently changed to brown by the absorption of divalent palladium species. The brown CM was treated with hydrazine hydrate in water to give the zero valent palladium-supported black CM, which was collected on filter paper, washed with MeOH and water, and dried in vacuo to give the 5% Pd/monolith-SO3H (5% Pd/CM) (Scheme 1).17
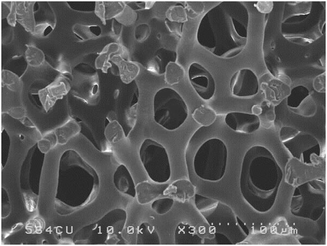 |
| Fig. 1 SEM image of unfunctionlized monolithic polymer. | |
 |
| Scheme 1 Preparation of 5% Pd/monolith-SO3H (Pd/CM). | |
A transmission electron microscope (TEM) image of the 5% Pd/CM shows that the palladium nanoparticles (approximately 5–10 nm) aggregate to form 5–30 nm size clusters (Fig. 2), which were revealed to be evenly distributed from the surface of the 5% Pd/CM to the inside by an electron probe microanalysis (EPMA) (Fig. 3). The aggregation pattern of palladium species as well as the palladium lording on 5% Pd/CM was quite similar to the case of 3.9% Pd/AM.18
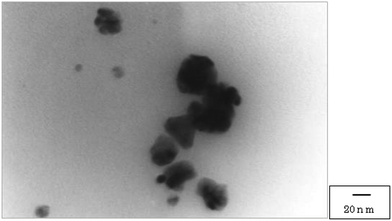 |
| Fig. 2 TEM image of 5% Pd/CM. | |
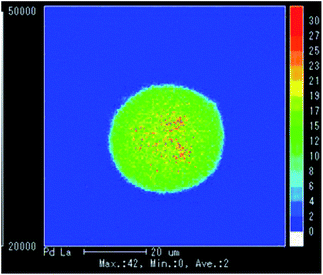 |
| Fig. 3 EPMA of 5% Pd/CM. | |
Pd/monolith-NMe3+NO3− (Pd/AM)- and Pd/monolith-SO3H (Pd/CM)-catalyzed hydrogenation
The catalytic activity of the 3.9% Pd/monolith-NMe3+NO3− (Pd/AM)19 and 5% Pd/CM for the hydrogenation of various reducible functionalities was first evaluated (Table 1). Alkyne (entries 1, 2, 29, and 30), alkene (entries 3, 4, 13–20, 25, and 26), azido (entries 5 and 6), and nitro (entries 7 and 8) functionalities were readily reduced to the corresponding products in good yields by either catalyst. These catalysts can also be used for the deprotection of aromatic (entries 9 and 10) and aliphatic N-Cbz groups (entries 11–16), benzyl esters (entries 17, 18, 21, and 22), and aryl benzyl ethers (entries 19–22), although the benzyl ester underwent partial transesterification with MeOH to afford the methyl ester due to the strong acidity of the support (CM) of Pd/CM (entry 18). While the Pd/CM effectively catalyzed the hydrogenolysis of aromatic ketones (entries 24 and 26) and benzyl alcohols (entries 24, 26, 28, and 30) to give the corresponding deoxygenated products, these reducible functionalities were virtually untouched under the Pd/AM-catalyzed conditions (entries 23, 25, 27, and 29). An exposition of benzhydrol under the Pd/AM-catalyzed hydrogenation conditions afforded a very small amount of the dehydrogenated product, benzophenone (entry 27). The different chemoselectivity toward the hydrogenolysis of aromatic carbonyls and benzyl alcohols, i.e., higher activity of 5% Pd/CM compared with 3.9% Pd/AM, would be attributed to the strong Brønsted acidity of CM; thus, the activation of carbonyl groups and benzyl alcohols by the protonation would promote the reactions.
Table 1 3.9% Pd/AM- and 5% Pd/CM-catalyzed hydrogenations
The catalyst activities of the 3.9% Pd/AM and 5% Pd/CM together with catalysts previously developed by us are summarized in Fig. 4. The reducible functional groups in the specific frameworks could be reduced using the corresponding catalysts. While 5% Pd/CM can catalyze the hydrogenation of a wide range of reducible functionalities as with Pd/C and Pd/HP20,2b the use of 3.9% Pd/AM makes it possible to undergo a novel hydrogenation, i.e., aromatic carbonyl groups are tolerant, while N-Cbz, benzyl ester, and aryl benzyl ether can be hydrocracked.
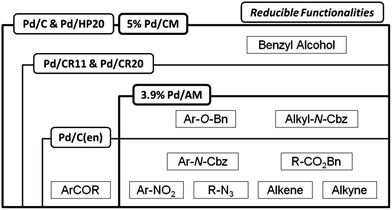 |
| Fig. 4 Chemoselectivity of 3.9% Pd/AM- and 5% Pd/CM-catalyzed hydrogenation. | |
Pd/AM- and Pd/CM-catalyzed cross-coupling reactions
The Suzuki–Miyaura coupling reaction between aryl bromides and arylboronic acids (1.5 equiv.) were carried out using 0.5 mol% Pd/AM or Pd/CM and Na3PO4·12H2O (3.5 equiv.) in 50% aqueous iPrOH (Table 2). Although the reaction time was dependent on the kind of aryl bromides, the desired biaryls could be smoothly obtained using either 3.9% Pd/AM or 5% Pd/CM at room temperature as is the case in 10% Pd/C that we have previously reported.20 The phenylation of the non-protected free bromoaniline was completed within only 1.5 h (entries 10 and 11), while 24 h was not long enough for the reaction completion by 10% Pd/C (entry 12). These results were probably caused by the hydrophilicity of the amino group, and the bromoaniline could be more effectively absorbed into the amphipathic monolith.
Table 2 3.9% Pd/AM- and 5% Pd/CM-catalyzed Suzuki–Miyaura reactions
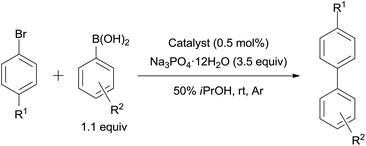
|
Entry |
R1 |
R2 |
Catalyst |
Time (h) |
Yield (%) |
Results from ref. 20. 80 °C. Results from ref. 2c. The reaction was carried out in H2O. |
1 |
NO2 |
H |
3.9% Pd/AM |
24 |
93 |
2 |
|
|
5% Pd/CM |
6 |
92 |
3a |
|
|
10% Pd/C |
3.5 |
93 |
4 |
CO2Et |
H |
3.9% Pd/AM |
24 |
99 |
5 |
|
|
5% Pd/CM |
2 |
96 |
6a |
|
|
10% Pd/C |
6 |
99 |
7 |
CO2H |
H |
3.9% Pd/AM |
7 |
96 |
8 |
|
|
5% Pd/CM |
7 |
100 |
9a |
|
|
10% Pd/C |
3.5 |
100 |
10b |
NH2 |
H |
3.9% Pd/AM |
0.5 |
95 |
11b |
|
|
5% Pd/CM |
1.5 |
84 |
12b,c |
|
|
10% Pd/C |
24 |
65 |
13 |
OMe |
H |
3.9% Pd/AM |
24 |
94 |
14 |
|
|
5% Pd/CM |
19 |
97 |
15a |
|
|
10% Pd/C |
24 |
93 |
16 |
OH |
H |
3.9% Pd/AM |
24 |
94 |
17 |
|
|
5% Pd/CM |
24 |
96 |
18a |
|
|
10% Pd/C |
3.5 |
91 |
19d |
OMe |
2-OMe |
3.9% Pd/AM |
12 |
86 |
20d |
|
|
5% Pd/CM |
4 |
94 |
21a |
|
|
10% Pd/C |
6 |
90 |
22 |
NO2 |
4-Ac |
3.9% Pd/AM |
23 |
98 |
23 |
|
|
5% Pd/CM |
2 |
88 |
24c |
|
|
10% Pd/C |
12 |
91 |
The 0.2 mol% of 3.9% Pd/AM- or 5% Pd/CM-catalyzed Mizoroki–Heck reaction of aryl iodides with benzyl acrylate (1.2 equiv.) in the presence of Bu3N (1.1 equiv.) in N,N-dimethylacetamide (DMA) at 100 °C efficiently proceeded regardless of the electronic property of the substituent on the benzene ring of the aryl iodides to give the corresponding butyl cinnamate derivatives in excellent yields (Table 3, entries 1, 2, 4, 5, 7, 8, 10, and 11). The 3.9% Pd/AM or 5% Pd/CM catalyzed the cross-coupling between iodobenzene and styrene in a manner similar to 10% Pd/HP20 (entries 13–15).2c It is notable that both catalysts effectively worked for the β-phenylation of the acryl amide and acrylonitrile (entries 16, 17, 19, and 20), the catalyst efficiency of which was quite low under the Pd/HP20-catalyzed conditions (entries 18 and 21). The polarity of the functional groups (trimethylammonium and sulfonic acid) on the monolith resin would increase the affinity for polar substrates in comparison to the simple polystyrene–divinyl benzene-based polymer of HP20 thus leading to the enhanced reaction efficiency.
Table 3 3.9% Pd/AM- and 5% Pd/CM-catalyzed Mizoroki–Heck reactions
The 3.9% Pd/AM and 5% Pd/CM could be used for the copper- and amine-free Sonogashira-type reaction in the absence of ligands (Table 4). A wide variety of aryl iodides coupled with aromatic or aliphatic alkynes (1.2 equiv.) in the presence of 0.4 mol% of 3.9% Pd/AM or 5% Pd/CM in 50% aqueous iPrOH at 80 °C afforded the corresponding disubstituted alkynes.
Table 4 3.9% Pd/AM- and 5% Pd/CM-catalyzed Sonogashira-type reactions
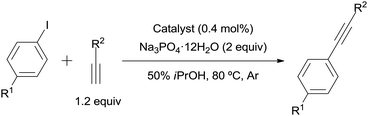
|
Entry |
R1 |
R2 |
Catalyst |
Time (h) |
Yield (%) |
Results from ref. 21. 2 equiv. of ethynylbenzene were used. |
1 |
Ac |
Ph |
3.9% Pd/AM |
1 |
99 |
2 |
|
|
5% Pd/CM |
1 |
100 |
3a |
|
|
10% Pd/C |
0.5 |
95 |
4 |
OMe |
Ph |
3.9% Pd/AM |
17.5 |
61 |
5b |
|
|
5% Pd/CM |
24 |
61 |
6a |
|
|
10% Pd/C |
0.5 |
51 |
7 |
H |
Ph |
3.9% Pd/AM |
1 |
56 |
8 |
|
|
5% Pd/CM |
1 |
64 |
9a |
|
|
10% Pd/C |
0.5 |
66 |
10 |
Ac |
2-CF3C6H4 |
3.9% Pd/AM |
1 |
87 |
11 |
|
|
5% Pd/CM |
1 |
84 |
12a |
|
|
10% Pd/C |
1 |
90 |
13 |
Ac |
(CH2)2OH |
3.9% Pd/AM |
7 |
79 |
14 |
|
|
5% Pd/CM |
1 |
76 |
15a |
|
|
10% Pd/C |
1 |
85 |
16 |
Ac |
4-MeC6H4 |
3.9% Pd/AM |
4 |
94 |
17 |
|
|
5% Pd/CM |
4 |
80 |
Reuse test of Pd/AM and Pd/CM for hydrogenation and Sonogashira-type reaction
After the Pd/AM- and Pd/CM-catalyzed hydrogenations of 1,2-dimethoxy-4-(1-propenyl)benzene in MeOH at room temperature, the palladium species leached into the filtrate were measured by atomic absorption spectrometry. In each case, no palladium species were observed in the filtrate within the detectable limits (<1 ppm). Therefore, the hydrogenation would be expected to cause no or little degradation to the Pd/AM and Pd/CM, and they could be reused until at least the 5th run without any loss of catalyst activities (Table 5).
Table 5 Reuse test of Pd/AM and Pd/CM for hydrogenation
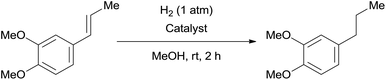
|
Run |
3.9% Pd/AM |
5% Pd/CM |
Yield (%) of product |
Recovered catalyst |
Yield (%) of product |
Recovered catalyst |
1st |
100 |
96 |
94 |
100 |
2nd |
97 |
97 |
99 |
99 |
3rd |
100 |
99 |
100 |
99 |
4th |
99 |
96 |
100 |
99 |
5th |
100 |
100 |
100 |
100 |
We have previously reported that approximately 25% of the palladium metal was leached from 10% Pd/C during the copper- and amine-free Pd/C-catalyzed Sonogashira-type reaction between 4′-iodoacetophenone and 3-butyn-1-ol [inductively coupled plasma-atomic emission spectrometry (ICP-AES)],21 and the catalyst could be reused only once. The use of simple polystyrene–divinylbenzene copolymer-supported 10% Pd/HP20 for the reaction of 4′-iodoacetophenone with ethynylbenzene led to a reduction in the palladium leaching ratio to 2.4% probably due to the relatively strong interaction between the palladium species and aromatic rings of HP20.2c Since no detectable palladium leaching from Pd/C and Pd/HP20 has been observed in the cases of other cross-coupling reactions, such as the Suzuki–Miyaura2c,20 and Mizoroki–Heck2c reactions, the significant palladium leaching during the copper-free, heterogeneous Sonogashira-type reaction is an important issue that needs to be addressed. While a very small amount of palladium leaching (1.9%) was observed when 3.9% Pd/AM was used for the cross-coupling reaction between 4′-iodoacetophenone and ethynylbenzene, palladium species were never detected by ICP-AES in the case of the 5% Pd/CM. The less palladium leaching would be arisen from the good interaction of palladium metals with functional groups on the highly porous monolithic polymer. Hence, the reuse test of the 5% Pd/CM was investigated (Table 6). The weight of the recovered Pd/CM after the first run increased compared to the initial use probably because of the partial or full exchange of protons of the monolith-SO3H to the sodium cations of Na3PO4·12H2O. The recovered catalyst was used for the second run without any further acid treatment, and no increase in the weight of the recovered catalyst was observed after the second run. Although the catalyst activity only slightly decreased after the fourth run, the catalyst could be reused without any significant decrease in the catalyst activity. The palladium leaching-free and reusable properties of the copper- and amine-free Sonogashira-type reaction are remarkable advantages of the 5% Pd/monolith-SO3H.
Table 6 Reuse test of 5% Pd/CM for Sonogashira-type reaction
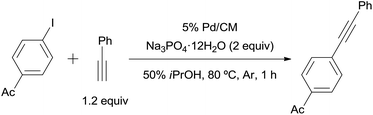
|
Run |
Yield (%) of producta |
Recovered Pd/CM (%) |
Determined by 1H NMR using 1,4-dioxane as the internal standard. Yield of recovered 4′-iodoacetophenone. |
1st |
100 |
>100 |
2nd |
100 |
91 |
3rd |
95 (5)b |
95 |
4th |
93 (7)b |
88 |
Conclusions
A palladium catalyst supported on the amphipathic, monolithic polystyrene–divinylbenzene polymer bearing ammonium functions (Pd/AM) as well as a newly-developed sulfonic acid-bound monolithic polymer-supported palladium (Pd/CM) could be used for hydrogenation reactions. It is noteworthy that a novel chemoselective hydrogenation was successively achieved under the Pd/AM-catalyzed conditions; aromatic ketones could not be reduced to the corresponding benzyl alcohols, and benzyl alcohols were also intact, while Pd/CM catalyzed the hydrogenation of various reducible functionalities. Both catalysts were applicable for the ligand-free cross-coupling reactions, Suzuki–Miyaura, Mizoroki–Heck, and copper- and amine-free Sonogashira-type reactions. When Pd/CM was used for the Sonogashira-type reaction, no palladium leaching into the reaction media was observed despite the use of an alkyne as a substrate. Furthermore, Pd/CM could be recovered and reused for the Sonogashira-type reactions for at least four runs. The application of these catalysts for the flow reactions is now ongoing in our laboratory.
Experimental
Preparation of 5% Pd/CM (Scheme 1)
The monolith-SO3H polymer (CM) was cut into 1 mm cubes using a ceramic knife, washed with H2O and MeOH, and dried overnight under reduced pressure. The dried CM (1.00 g) was immersed in a solution of Pd(OAc)2 [111 mg, 495 μmol (52.6779 mg for Pd)] in MeOH (30 mL), and stirred at room temperature under an Ar atmosphere for 24 h. The resulting brown polymer was collected on a Kiriyama funnel (1 μm), washed with MeOH (5 × 20 mL) and H2O (5 × 20 mL), then MeOH (5 × 20 mL), and dried under vacuum for 12 h. The filtrate was concentrated in vacuo, then transferred to a 100 mL volumetric flask with H2O. Its atomic absorption analysis indicated that 0.32224 mg L−1 (32.224 μg) of palladium species was present. To a suspension of the collected solid in H2O (20 mL) was added NH2NH2·H2O (74.3 mg, 1.47 mmol), and the mixture was stirred at room temperature under an Ar atmosphere for 24 h. The black solid was collected on a Kiriyama funnel (1 μm), washed with H2O (5 × 20 mL) and MeOH (5 × 20 mL), then dried under vacuum for 24 h to produce the Pd/CM (1.05 g). The filtrate was concentrated in vacuo, then transferred to a 100 mL volumetric flask with H2O. Its atomic absorption analysis indicated that 0.82912 mg L−1 (82.912 μg) of the palladium species was present. The palladium amount, which was not captured on the CM, was found to be 115.136 μg (32.224 + 82.912 μg), which means that the palladium ratio of Pd/CM was 5.0% [(52.6779 − 0.115136)/1050 × 100].
Typical procedure for the 3.9% Pd/AM- and 5% Pd/CM-catalyzed hydrogenation (Table 1)
A mixture of the substrate (200 μmol) and 3.9% Pd/AM (5.5 mg, 2.00 μmol) or 5% Pd/CM (4.3 mg, 2.00 μmol) in MeOH (1 mL) was stirred under an H2 atmosphere (balloon) at room temperature for a specific time, then passed through a cotton filter to remove the catalyst. The filtrate was concentrated in vacuo to give the analytically pure product. The spectral data of the product were identical to those in the literature.
Typical procedure for the 3.9% Pd/AM- and 5% Pd/CM-catalyzed Suzuki–Miyaura reactions (Table 2)
A mixture of the aryl halide (500 μmol), arylboronic acid (550 μmol), Na3PO4·12H2O (665 mg, 1.75 mmol) and Pd/AM (6.8 mg, 2.50 μmol) or Pd/CM (5.3 mg, 2.50 μmol) in H2O (1 mL)–iPrOH (1 mL) or in H2O (2 mL) in a test tube under an Ar atmosphere was stirred at room temperature or 80 °C. After complete consumption of the aryl halide was confirmed by TLC analyses or after 24 h (if the reaction was incomplete), the mixture was passed through a cotton filter to remove the catalyst. To the filtrate were added EtOAc (20 mL) and saturated aqueous NH4Cl (20 mL), then the layers were separated. The organic layer was washed with saturated aqueous NH4Cl (2 × 20 mL) and brine (20 mL), dried over MgSO4, filtered, and concentrated in vacuo. The residue was purified by flash silica gel column chromatography (n-hexane/EtOAc or CH2Cl2/MeOH/AcOH) to give the corresponding biaryl. The spectral data of the product were identical to those in the literature.
Typical procedure for the 3.9% Pd/AM- and 5% Pd/CM-catalyzed Mizoroki–Heck reactions (Table 3)
A mixture of the aryl iodide (500 μmol), mono-substituted alkene (600 μmol), Bu3N (102 mg, 550 μmol), and Pd/AM (2.7 mg, 1.00 μmol) or Pd/CM (2.1 mg, 1.00 μmol) in DMA (2 mL) in a test tube under an Ar atmosphere was stirred at 100 °C or 80 °C. After complete consumption of the aryl iodide was confirmed by TLC analyses or after 24 h (if the reaction was incomplete), the mixture was passed through a cotton filter to remove the catalyst. To the filtrate were added EtOAc (20 mL) and saturated aqueous NH4Cl (20 mL), then the layers were separated. The organic layer was washed with saturated aq. NH4Cl (2 × 20 mL) and brine (20 mL), dried over MgSO4, and concentrated in vacuo. The residue was purified by silica gel flash column chromatography (n-hexane/EtOAc) to give the corresponding disubstituted alkene derivative. The spectral data of the product were identical to those in the literature. For entries 19 and 20, the reactions were performed using 2 mol% of Pd/AM (27.3 mg, 10.0 μmol) or Pd/CM (21.3 mg, 10.0 μmol).
Typical procedure for the 3.9% Pd/AM- and 5% Pd/CM-catalyzed Sonogashira-type reactions (Table 4)
A mixture of the aryl iodide (500 μmol), the mono-substituted alkyne (600 μmol), Na3PO4·12H2O (380 mg, 1.00 mmol) and Pd/AM (5.5 mg, 2.00 μmol) or Pd/CM (4.3 mg, 2.00 μmol) in H2O (1 mL)–iPrOH (1 mL) in a test tube under an Ar atmosphere was stirred at 80 °C. After complete consumption of the aryl iodide was confirmed by TLC analyses, the mixture was passed through a cotton filter to remove the catalyst. To the filtrate were added EtOAc (20 mL) and saturated aqueous NH4Cl (20 mL), then the layers were separated. The organic layer was washed with saturated aqueous NH4Cl (2 × 20 mL) and brine (20 mL), dried over MgSO4, filtered, and concentrated in vacuo. The residue was purified by flash silica gel column chromatography (n-hexane/EtOAc) to give the corresponding disubstituted alkyne. The spectral data of the product were identical to those in the literature. For entry 5, the reaction was performed using 2 equiv. of ethynylbenzene (102 mg, 1.00 mmol).
Notes and references
-
(a) L. Yin and J. Liebscher, Chem. Rev., 2007, 107, 133 CrossRef CAS PubMed;
(b) M. J. Climent, A. Corma and S. Iborra, Chem. Rev., 2011, 111, 1072 CrossRef CAS PubMed.
- For unfunctionalized polymer as the support:
(a) R. Akiyama and S. Kobayashi, Angew. Chem., Int. Ed., 2001, 40, 3469 (Angew. Chem., 2001, 113, 3577) CrossRef CAS;
(b) Y. Monguchi, Y. Fujita, K. Endo, S. Takao, M. Yoshimura, Y. Takagi, T. Maegawa and H. Sajiki, Chem.–Eur. J., 2009, 15, 834 CrossRef CAS PubMed;
(c) Y. Monguchi, K. Sakai, K. Endo, Y. Fujita, M. Niimura, M. Yoshimura, T. Mizusaki, Y. Sawama and H. Sajiki, ChemCatChem, 2012, 4, 546 CrossRef CAS.
-
(a) D. Sengupta, J. Saha, G. De and B. Basu, J. Mater. Chem. A, 2014, 2, 3986 RSC;
(b) V. Pandarus, D. Desplantier-Giscard, G. Gingras, F. Béland, R. Ciriminna and M. Pagliaro, Org. Process Res. Dev., 2013, 17, 1492 CrossRef CAS;
(c) B. Tamami, M. M. Nezhad, S. Ghasemi and F. Farjadian, J. Organomet. Chem., 2013, 743, 10 CrossRef CAS;
(d) M. Bakherad, A. Keivanloo and S. Samangooei, Tetrahedron Lett., 2012, 53, 5773 CrossRef CAS;
(e) Y. M. A. Yamada, S. M. Sarkar and Y. Uozumi, J. Am. Chem. Soc., 2012, 134, 3190 CrossRef CAS PubMed;
(f) G. Li, H. Yang, W. Li and G. Zhang, Green Chem., 2011, 13, 2939 RSC;
(g) B. Karimi and P. F. Akhavan, Inorg. Chem., 2011, 50, 6063 CrossRef CAS PubMed;
(h) P. Das, D. Sharma, A. K. Shil and A. Kumari, Tetrahedron Lett., 2011, 52, 1176 CrossRef CAS;
(i) M.-J. Jin and D.-H. Lee, Angew. Chem., Int. Ed., 2010, 49, 1119 (Angew. Chem., 2010, 122, 1137) CrossRef CAS PubMed;
(j) D.-H. Lee, M. Choi, B.-W. Yu, R. Ryoo, A. Taher, S. Hossain and M.-J. Jin, Adv. Synth. Catal., 2009, 351, 2912 CrossRef CAS;
(k) B. Basu, S. Das, P. Das, B. Mandal, D. Banerjee and F. Almqvist, Synthesis, 2009, 7, 1137 CrossRef;
(l) S. Schweizer, J.-M. Becht and C. L. Drian, Org. Lett., 2007, 9, 3777 CrossRef CAS PubMed;
(m) R. Sayah, K. Glegola, E. Framery and V. Dufaud, Adv. Synth. Catal., 2007, 349, 373 CrossRef CAS;
(n) L. Wu, B.-O. Li, Y.-Y. Huang, H.-F. Zhou, Y.-M. He and Q.-H. Fan, Org. Lett., 2006, 8, 3605 CrossRef CAS PubMed;
(o) K. Okamoto, R. Akiyama, H. Yoshida, T. Yoshida and S. Kobayashi, J. Am. Chem. Soc., 2005, 127, 2125 CrossRef CAS PubMed;
(p) A. N. Cammidge, N. J. Baines and R. K. Bellingham, Chem. Commun., 2001, 2588 RSC.
-
(a) Y. Yabe, Y. Sawama, Y. Monguchi and H. Sajiki, Catal. Sci. Technol., 2014, 4, 260 RSC;
(b) H.-Y. Lee, S. Ryu, H. Kang, Y.-w. Jun and J. Cheon, Chem. Commun., 2006, 1325 RSC;
(c) H. Hagiwara, T. Nakamura, T. Hoshi and T. Suzuki, Green Chem., 2011, 13, 1131 RSC;
(d) M. Armbrüster, M. Behrens, F. Cinquini, K. Föttinger, Y. Grin, A. Haghofer, B. Klötzer, A. Knop-Gericke, H. Lorenz, A. Ota, S. Penner, J. Prinz, C. Rameshan, Z. Révay, D. Rosenthal, G. Rupperchter, P. Sautet, R. Schlögl, L. Shao, L. Szentmiklósi, D. Teschner, D. Torres, R. Wagner, R. Widmer and G. Wowsnick, ChemCatChem, 2012, 4, 1048 CrossRef;
(e) M. V. Vasylyev, G. Maayan, Y. Hovav, A. Haimov and R. Neumann, Org. Lett., 2006, 8, 5445 CrossRef CAS PubMed;
(f) J. Le Bras, D. K. Mukherjee, S. González, M. Tristany, B. Ganchegui, M. Moreno-Maňas, R. Pleixats, F. Henin and J. Muzart, New J. Chem., 2004, 28, 1550 RSC;
(g) E. Thiery, J. Le Bras and J. Muzart, Green Chem., 2007, 9, 326 RSC;
(h) N. M. Callis, E. Thiery, J. Le Bras and J. Muzart, Tetrahedron Lett., 2007, 48, 8128 CrossRef CAS.
-
(a) H. Sajiki, T. Ikawa and K. Hirota, Tetrahedron Lett., 2003, 44, 171 CrossRef CAS;
(b) H. Sajiki, T. Ikawa and K. Hirota, Tetrahedron Lett., 2003, 44, 8437 CrossRef CAS;
(c) T. Ikawa, H. Sajiki and K. Hirota, Tetrahedron, 2005, 61, 2217 CrossRef CAS;
(d) Y. Kitamura, A. Tanaka, M. Sato, K. Oono, T. Ikawa, T. Maegawa, Y. Monguchi and H. Sajiki, Synth. Commun., 2007, 37, 4381 CrossRef CAS;
(e) T. Ikawa, H. Sajiki and K. Hirota, J. Synth. Org. Chem., Jpn., 2005, 63, 1218 CrossRef CAS.
-
(a) T. Maegawa, T. Takahashi, M. Yoshimura, H. Suzuka, Y. Monguchi and H. Sajiki, Adv. Synth. Catal., 2009, 351, 2091 CrossRef CAS;
(b) T. Takahashi, M. Yoshimura, H. Suzuka, T. Maegawa, Y. Sawama, Y. Monguchi and H. Sajiki, Tetrahedron, 2012, 68, 8293 CrossRef CAS.
-
(a) Y. Yabe, T. Yamada, S. Nagata, Y. Sawama, Y. Monguchi and H. Sajiki, Adv. Synth. Catal., 2012, 354, 1264 CrossRef CAS;
(b) Y. Yabe, Y. Sawama, Y. Monguchi and H. Sajiki, Chem.–Eur. J., 2013, 19, 484 CrossRef CAS PubMed;
(c) A. Kawanishi, C. Miyamoto, Y. Yabe, M. Inai, T. Asakawa, Y. Hamashima, H. Sajiki and T. Kan, Org. Lett., 2013, 15, 1306 CrossRef CAS PubMed;
(d) Y. Yabe, Y. Sawama, T. Yamada, S. Nagata, Y. Monguchi and H. Sajiki, ChemCatChem, 2013, 5, 2360 CrossRef CAS.
- Y. Monguchi, T. Ichikawa, K. Nozaki, K. Kihara, Y. Yamada, Y. Miyake, Y. Sawama and H. Sajiki, Tetrahedron, 2015, 71, 6499 CrossRef CAS.
- Y. Monguchi, T. Marumoto, T. Ichikawa, Y. Miyake, Y. Nagae, M. Yoshida, Y. Oumi, Y. Sawama and H. Sajiki, ChemCatChem, 2015, 7, 2155 CrossRef CAS.
-
(a) K. Mennecke and A. Kirschning, Beilstein J. Org. Chem., 2009, 5, 21 Search PubMed;
(b) W. Solodenko, H. Wen, S. Leue, F. Stuhlmann, G. Sourkouni-Argirusi, G. Jas, H. Schönfeld, U. Kunz and A. Kirschning, Eur. J. Org. Chem., 2004, 3601 CrossRef CAS;
(c) K. Mennecke, R. Cecilia, T. N. Glasnov, S. Gruhl, C. Vogt, A. Feldhoff, M. A. L. Vargas, C. O. Kappe, U. Kunz and A. Kirschning, Adv. Synth. Catal., 2008, 350, 717 CrossRef CAS.
- S. Kumari, A. Kulkarni, G. Kumarasawamy and S. S. Gupta, Chem. Mater., 2013, 25, 4813 CrossRef CAS.
- D. Kundu, A. K. Patra, J. Sakamoto and H. Uyama, React. Funct. Polym., 2014, 79, 8 CrossRef CAS.
- M. Nandi and H. Uyama, RSC Adv., 2014, 4, 20847 RSC.
- H. Inoue, H. Takada and M. Murayama, Kobunshi Ronbunshu, 2011, 68, 320 CrossRef . Pd/AM was industrially produced by Organo Corporation according to the procedure described in Note and reference;14 palladium species were immobilized on the ammonium-functionalized monolithic polymer (AM), and then reduced with NH2NH2. The palladium loading was determined to be 3.9 weight% by the inductively coupled plasma-atomic emission spectrometry (ICP-AES) after the degradation of Pd/AM using a microwave heating.
- H. Inoue, K. Yamanaka, A. Yoshida, T. Aoki, T. Kaneko and M. Teraguchi, Kobunshi Ronbunshu, 2004, 61, 301 CrossRef CAS.
- Reviews including transition metal catalysts immobilized on amphipathic supports:
(a) Á. Molnár, Chem. Rev., 2011, 111, 2251 CrossRef PubMed;
(b) J. Lu and P. H. Toy, Chem. Rev., 2009, 109, 815 CrossRef CAS PubMed;
(c) S. Ikegami and H. Hamamoto, Chem. Rev., 2009, 109, 583 CrossRef CAS PubMed.
- 4.6 mmol g−1 of sulfonic acid groups are distributed evenly on the polymer, see the ESI.† The molar ratio of sulfonic acids to palladium metals is 9.8.
- The TEM image of 3.9% Pd/AM indicates that 10–30 nm-Pd clusters are formed by the aggregation of Pd nanoparticles (approximately 5 nm). The palladium species were found to be dispersed into the whole monolithic polymer by EPMA, although a partial localization of the species inside the monolithic polymer was also observed, see the ESI.†.
- 4.3 mmol g−1 of ammonium groups are distributed evenly on the polymer, see the ESI.† The molar ratio of ammonium functionalities to palladium metals is 10.5.
- T. Maegawa, Y. Kitamura, S. Sako, T. Udzu, A. Sakurai, A. Tanaka, Y. Kobayashi, K. Endo, U. Bora, T. Kurita, A. Kozaki, Y. Monguchi and H. Sajiki, Chem.–Eur. J., 2007, 13, 5937 CrossRef CAS PubMed.
- S. Mori, T. Yanase, S. Aoyagi, Y. Monguchi, T. Maegawa and H. Sajiki, Chem.–Eur. J., 2008, 14, 6994 CrossRef CAS PubMed.
Footnote |
† Electronic supplementary information (ESI) available: Experimental details, spectral data and NMR spectra of products. See DOI: 10.1039/c6ra24769e |
|
This journal is © The Royal Society of Chemistry 2017 |
Click here to see how this site uses Cookies. View our privacy policy here.